Highlights
- •
The increase in 1 nm (from 6 to 7) increased the Mg adsorption.
- •
Mg adsorption did not alter the mesoporous surface topography.
- •
Osteogenesis is promoted by Mg released from 7 nm films.
- •
Mg form 7 nm films significantly increased bone morphogenic protein 4 expression.
- •
Mesoporous bioactivity is enhanced by 1 nm pore size increase.
Abstract
Objective
Mesoporous (MP) titania films used as implant coatings have recently been considered as release systems for controlled administration of magnesium to enhance initial osteoblast proliferation in vitro . Tuning of the pore size in such titania films is aimed at increasing the osteogenic potential through effects on the total loading capacity and the release profile of magnesium.
Methods
In this study, evaporation-induced self-assembly (EISA) was used with different structure-directing agents to form three mesoporous films with average pore sizes of 2 nm (MP1), 6 nm (MP2) and 7 nm (MP3). Mg adsorption and release was monitored using quartz crystal microbalance with dissipation (QCM-D). The film surfaces were characterized with atomic force microscopy (AFM), scanning electron microscopy (SEM) and X-ray photoelectron spectroscopy (XPS). The effect of different Mg release on osteogenesis was investigated in human fetal osteoblasts (hFOB) using pre-designed osteogenesis arrays and real-time polymerase chain reaction (RT-PCR).
Results
Results showed a sustained release from all the films investigated, with higher magnesium adsorption into MP1 and MP3 films. No significant differences were observed in the surface nanotopography of the films, either with or without the presence of magnesium. MP3 films (7 nm pore size) had the greatest effect on osteogenesis, up-regulating 15 bone-related genes after 1 week of hFOB growth and significantly promoting bone morphogenic protein (BMP4) expression after 3 weeks of growth.
Significance
The findings indicate that the increase in pore width on the nano scale significantly enhanced the bioactivity of the mesoporous coating, thus accelerating osteogenesis without creating differences in surface roughness.
1
Introduction
One of the main goals in placing a dental implant is to achieve a rapid integration with the bone to attain a firm anchorage. Both surface topography and surface chemistry have proven to be crucial for bone apposition and formation around the implant and numerous modifications have been undertaken to contribute to the improvement of so-called osseointegration.
The application of mesoporous titania films to the surface has been proposed as a method to improve the bioactivity of an implant . The improvement is due to the ability to incorporate bioactive substances into the films, facilitating their release at the peri-implant region in a controlled and sustained manner. One approach is to load the films with different drugs, e.g. osteoporosis medications, in order to obtain stronger biomechanical interlocking of the implant into the host bone . Alendronate and raloxifene have been used as candidates to assess the capability of mesoporous films to act as carrier frameworks at the bone-implant site in vivo . These studies showed an increased local bone density around implants loaded with the drugs. In order to adjust the drug content that can be released from the implant, mesoporous films with different pore sizes have been investigated. It was demonstrated that the pore diameter can be modulated using different structure directing agents and that the amount of drugs and their release profiles is affected by the pore-size .
Despite the positive results using osteoporosis medications to improve osseointegration, clinical trials are needed prior to the launch of such drug-releasing medical devices to ensure that there are no negative clinical effects. For example, the intravenous application of bisphosphonates has shown negative side-effects when used in high concentrations, which can result in necrosis of the jaw (BRONJ), an impaired bone turnover which leads to the exposure of necrotic bone in the oral cavity .
An alternative strategy is to bio-functionalize the implant surface with bioactive elements that participate in the osseointegrative process, such as calcium, phosphate, fluoride and magnesium . Sul et al. have reported that Ca- and Mg-incorporation into titanium implants significantly increased the strength and speed of osseointegration compared to non-ion incorporated commercially pure titanium implants . Mg- and Ca-incorporated titanium surfaces may electrostatically bond with polyanionic proteins, such as proteoglycans, collagen, fibronectin, vitronectin, osteoadherin, osteopontin and bone sialoprotein. This process triggers the recruitment of osteoprogenitor cells and osteoblasts, which possibly explains the rapid and strong bone formation seen with Mg- and Ca-incorporated titanium surfaces. Magnesium ions also play a critical role in the DNA polymerase binding site, catalysing the DNA polymerase mechanisms for repair and synthesis .
The concept of drug delivery from smooth mesoporous surfaces is of significant interest since excessively rough surfaces, designed to stimulate bone growth, have been proved to provoke negative biological consequences. This is most likely bcause they can act as a substrate for colonization by oral bacteria, which can induce progressive peri-implant bone loss . In addition, even moderately rough surfaces have been proved a tendency for bacterial biofilm accumulation in vitro compared to smooth ones . Recent reports have suggested that implant surfaces with smoother microtopographies function as well as rougher surfaces in vivo and clinical studies .
Thus bone integration could be enhanced by the controlled release of osteogenic substances from mesoporous smooth surfaces. We previously investigated the use of mesoporous thin films as a carrier for magnesium that was gradually released when in contact with bone cells and bone tissues. Both in vitro and in vivo studies demonstrated that the magnesium release positively influenced differentiation of progenitor cells into cells with an osteoblast phenotype and activity as well as improving the osteogenic environment and bone anchorage in a rabbit model . These mesoporous films possessed a smooth surface (S a lower that 0.5 μm) in accordance to the Wennerberg and Albrektsson guidelines , an average pore size of 6.0 nm and successful loading of Mg onto the coating. Although positive biological responses were observed from these studies, we speculated that there were still possibilities to increase the Mg content and, therefore, its osteogenic potential in biological systems.
Thus, in the present study, the effect of pore size of mesoporous thin films as well as magnesium release from them was investigated in vitro . Three mesoporous titania films with 2 nm, 6 nm, and 7 nm average pore diameters, comprehensively characterized in terms of material properties and drug delivery by Karlsson et al. in a recent investigation , were used in this study. Mg adsorption and release, and its osteogenic effect were investigated using human fetal osteoblasts. It was hypothesized that larger pores would show increased magnesium adsorption, giving a stronger effect on the expression of bone-related proteins in the osteoblast population.
2
Materials and methods
2.1
Surface preparation
Commercially pure titanium (CpTi, grade 4) discs with a diameter of 12 mm and a thickness of 1 mm were used in this study. Samples were coated with mesoporous titania thin films with 3 different pore sizes formed by the evaporation-induced self-assembly (EISA) method. The discs were divided into 3 groups: mesoporous films with a 2 nm average pore size (MP1); mesoporous films with a 6 nm average pore size (MP2) and mesoporous films with a 7 nm average pore size (MP3). For the formation of the films, a titania precursor solution was prepared by adding titanium tetraethoxide (TEOT, 20%) in concentrated hydrochloric acid (HCL, 37%) to a mixture containing the different structure-directing agents. The two amphiphiles, Brij-S10 (C18H37 (OCH2CH2) n OH, Aldrich) and Pluronic P123 (triblock copolymer EO20PO70EO20, Aldrich) were used as structure-directing agents for the formation of MP1 and MP2 respectively. Larger pores (MP3) were provided with the aid of an organic additive, polypropylene glycol (PPG, Mn ∼ 4000), which was used as a swelling agent. Above a certain concentration, titania precursor molecules aggregate into micelles whose shape and dimension are determined by the nature and the length of the precursor units as well as by other parameters such as solvent, pH, and temperature. The specific recipes for each group are presented in Table 1 .
Template | Directing-agent (g) | TEOT (g) | HCL (g) | Ethanol (g) |
---|---|---|---|---|
Brij-S10 | 0.52 | 2.1 | 0.7 | 12 |
P123 | 0.5 | 2.1 | 1.6 | 8.5 |
P123 + PPG (1:1) | 0.5 | 2.1 | 1.6 | 8.5 |
To obtain uniform films, 60 μL of the final solution were dropped onto Ti discs and spin-coated at 7000 rpm for 60 s. MP1 and MP2 groups were aged overnight at room temperature and then calcined in a ramping temperature of 1 °C/min and kept at 350 °C for 4 h while MP3 was aged in a sealed chamber containing saturated NaCl solution (RH = 70%) for 1 day prior to the calcination .
Magnesium loading was performed by soaking the samples in magnesium chloride solution (MgCl 2 ·6H 2 O) for 1 h and drying in an oven at 100 °C for liquid evaporation, as described in previous studies .
2.2
Quartz crystal microbalance with dissipation (QCM-D) monitoring
The adsorption and release of magnesium from the three mesoporous titania coatings deposited on 14 mm quartz crystal discs with a titanium layer were monitored by QCM-D (Q-sense AB, Gothenburg, Sweden). The Mg adsorption-release profile was obtained by loading MgCl 2 solution into the system while its release was quantified after changing the rinsing solution to Milli-Q water. All the frequency shifts are based on the data recorded at the 7th overtone and the collected data were processed using Q-tools software (Q-sense). Non-coated quartz crystals were used as controls. All experiments were performed twice for reliability. Data were reported as changes in the resonance frequency (Δ f ) as a function of time, which that can be converted into mass adsorption or desorption in the nanogram range by applying the Sauerbrey equation (Eq. (1)):
(1) <SPAN role=presentation tabIndex=0 id=MathJax-Element-1-Frame class=MathJax style="POSITION: relative" data-mathml='Δm=−C×1n×Δf’>Δm=−C×1n×ΔfΔm=−C×1n×Δf
Δ m = − C × 1 n × Δ f
where Δ m = adorbed-desorbed mass, C = mass sensitivity constant (17.7 ng/cm 2 Hz), Δ f = change in frenquency (Hz) and n = selected overtone.
2.3
Surface characterization
The films were examined by high-resolution scanning electron microscopy (SEM) (LEO Ultra 55 FEG scanning electron microscope, Zeiss, Oberkochen, Germany) with an accelerating voltage of 5 KV and atomic force microscopy (AFM, XE-100, Park Systems Corp, Suwon, Korea). The SEM observations were performed on 2 discs of each group to evaluate pore direction of the mesoporous coatings. For the AFM measurements, a 10 μm × 10 μm scan area was selected and the images obtained subjected to leveling Gaussian filtering with a cut-off of 2.5 μm. The following 3-dimensional parameters were chosen to describe the surface topography: arithmetic mean roughness ( S a ), developed surface area ratio ( S dr ) and density of the summits per unit of sampling area ( S ds ). The mean value and standard error were obtained from 9 scans of each group, from random sites on the surface. 3-D reconstructions were obtained using MountainMap Universal 6.2 Surface Analysis Software, cropping each image to 1 μm × 1 μm area.
The chemical composition of the mesoporous surfaces with and without magnesium was analyzed with XPS, utilizing a Quantum 2000 scanning XPS microprobe (Physical Instrument, USA). The instrument was equipped with a monochromatic Al K α X-ray source with a beam size of 100 μm and take-off angle of 45°. The depth of the analysis was about 5 nm and the point diameter analysis was 100 μm. The analysis was performed on 5 samples from each group. Data are presented as atomic percentage (at%) of the elements.
2.4
Cell culture
Human fetal osteoblasts (hFOB 1.19, ATCC CRL-11372) were grown in calcium and magnesium-free DMEM/high glucose (1×) (Thermo Scientific) supplemented with 10% FBS, 4 mM L-glutamine and 1% penicillin/streptomycin solution in a humidified atmosphere of 5% CO 2 in air at 34 °C . At confluence, cells were treated with 3 mL of 0.05% trypsin-0.02% EDTA solution and seeded onto with the discs at a density of 10 4 /cm 2 /100 μL. 160 μL of the cell suspension was added to each disk and incubated for 30 min to allow early cell adhesion. Then 1 mL of fresh medium was added and cells were further cultured for 1 and 3 weeks at 37 °C, with medium renewal every 2–3 days.
2.5
Total mRNA isolation and quality control
All steps involved in RNA isolation were performed in an RNase-free environment. The instruments and working station were carefully wiped with RNase-ExituPlus (Panrease) to remove RNases. After 1 and 3 weeks total RNA was isolated using the Aurum™ total RNA fatty and fibrous tissue kit (Bio-Rad), following the spin format (column) protocol. Therefore, after cell lysis with 1.5 mL of PureZOL, 200 μL of chloroform was added to each lysate and incubated for 5 min at room temperature (RT). The mixture was centrifuged (Eppendorf 2420R) at 12,000 × g for 15 min at 4 °C, after which the colorless aqueous phase on the top of each column (RNA interphase) was transferred into a new tube and 600 μL of 70% ethanol added to each sample. Afterwards, 700 μL of the mixture was transferred to RNA binding columns and centrifuged for 30 sec at 12,000 × g . The filtrate was discarded and each column was then washed with 700 μL of LOW stringency wash buffer by centrifuging at 12,000 × g for 30 s. RNA binding columns were also treated with DNase I enzyme for 15 min to remove contaminating DNA. DNase digestion was followed by two washes, one with HIGH stringency wash buffer and one again with LOW stringency wash buffer, centrifuging each time at 12,000 × g for 30 s. The RNA columns were centrifuged additionally for 2 min to remove any residual wash solution. Each RNA column was then transferred to a new 1.5 mL tube and RNA eluted with 35 μL of elution solution, centrifuging at 12,000 × g for 2 min. Total RNA was quantified using a UV spectrophotometer (Thermo Scientific Nano Drop 2000).
2.6
Real-time RT-PCR analysis
An osteogenesis-related gene array (Osteogenesis SAB Target List H96 Predesigned panel with SYBR Green, Bio-Rad) was used to reveal genes where expression changed in relation to magnesium release from the three mesoporous titania films. From each RNA sample (1000 ng of RNA sample from each group), cDNA was generated using an iScript Advanced cDNA Synthesis kit for RT-qPCR (Bio-Rad) and loading samples into a RT-PCR thermocycler (Bio-Rad CFX Connect™ Real-Time System). A total of 20 μL of PCR mixture, which included 10 μL of 2× SsoAdvanced universal SYBR Green super mix, 9 μL of high-purity water and 1 μL of cDNA, was loaded into each well of the pre-designed array. The PCR run was set up with 95 °C as the activation temperature for 2 min followed by 40 cycles of 95 °C for 5 s and 60 °C for 30 s. Data were analysed using the comparative cycle threshold method with normalization of the raw data to 5 housekeeping genes: ACTB (β-actin), β2M, RPLP0, HPRT1 and GAPDH.
Subsequently, 4 genes selected from the PCR array analyses were validated by quantitative real-time RT-PCR using 350 ng of RNA sample in triplicate from each group. 0.5 μL of each primer for each selected gene (BMP2, BMP4, MMP9, TGFB1 and GAPDH) was mixed with 5 μL of 2x SsoAdvanced universal SYBR Green super mix and 3.5 μL of high-purity water to give a total of 10 μL of PCR mixture for each reaction ( n = 3). The PCR run was set up following the protocol described above using the same RT-PCR system.
2.7
Statistical analysis
Statistical analysis of the AFM 3-D parameters was conducted by applying a non-paramentric Kruskal–Wallis test, followed by a Mann–Whitney U test for pairwise comparisons (SPSS Inc., Chicago. IL, USA). RT-PCR data were subjected to Student’s t -test using CFX Manager™ Software Gene Expression Analysis.
2
Materials and methods
2.1
Surface preparation
Commercially pure titanium (CpTi, grade 4) discs with a diameter of 12 mm and a thickness of 1 mm were used in this study. Samples were coated with mesoporous titania thin films with 3 different pore sizes formed by the evaporation-induced self-assembly (EISA) method. The discs were divided into 3 groups: mesoporous films with a 2 nm average pore size (MP1); mesoporous films with a 6 nm average pore size (MP2) and mesoporous films with a 7 nm average pore size (MP3). For the formation of the films, a titania precursor solution was prepared by adding titanium tetraethoxide (TEOT, 20%) in concentrated hydrochloric acid (HCL, 37%) to a mixture containing the different structure-directing agents. The two amphiphiles, Brij-S10 (C18H37 (OCH2CH2) n OH, Aldrich) and Pluronic P123 (triblock copolymer EO20PO70EO20, Aldrich) were used as structure-directing agents for the formation of MP1 and MP2 respectively. Larger pores (MP3) were provided with the aid of an organic additive, polypropylene glycol (PPG, Mn ∼ 4000), which was used as a swelling agent. Above a certain concentration, titania precursor molecules aggregate into micelles whose shape and dimension are determined by the nature and the length of the precursor units as well as by other parameters such as solvent, pH, and temperature. The specific recipes for each group are presented in Table 1 .
Template | Directing-agent (g) | TEOT (g) | HCL (g) | Ethanol (g) |
---|---|---|---|---|
Brij-S10 | 0.52 | 2.1 | 0.7 | 12 |
P123 | 0.5 | 2.1 | 1.6 | 8.5 |
P123 + PPG (1:1) | 0.5 | 2.1 | 1.6 | 8.5 |
To obtain uniform films, 60 μL of the final solution were dropped onto Ti discs and spin-coated at 7000 rpm for 60 s. MP1 and MP2 groups were aged overnight at room temperature and then calcined in a ramping temperature of 1 °C/min and kept at 350 °C for 4 h while MP3 was aged in a sealed chamber containing saturated NaCl solution (RH = 70%) for 1 day prior to the calcination .
Magnesium loading was performed by soaking the samples in magnesium chloride solution (MgCl 2 ·6H 2 O) for 1 h and drying in an oven at 100 °C for liquid evaporation, as described in previous studies .
2.2
Quartz crystal microbalance with dissipation (QCM-D) monitoring
The adsorption and release of magnesium from the three mesoporous titania coatings deposited on 14 mm quartz crystal discs with a titanium layer were monitored by QCM-D (Q-sense AB, Gothenburg, Sweden). The Mg adsorption-release profile was obtained by loading MgCl 2 solution into the system while its release was quantified after changing the rinsing solution to Milli-Q water. All the frequency shifts are based on the data recorded at the 7th overtone and the collected data were processed using Q-tools software (Q-sense). Non-coated quartz crystals were used as controls. All experiments were performed twice for reliability. Data were reported as changes in the resonance frequency (Δ f ) as a function of time, which that can be converted into mass adsorption or desorption in the nanogram range by applying the Sauerbrey equation (Eq. (1)):
(1) <SPAN role=presentation tabIndex=0 id=MathJax-Element-2-Frame class=MathJax style="POSITION: relative" data-mathml='Δm=−C×1n×Δf’>Δm=−C×1n×ΔfΔm=−C×1n×Δf
Δ m = − C × 1 n × Δ f
where Δ m = adorbed-desorbed mass, C = mass sensitivity constant (17.7 ng/cm 2 Hz), Δ f = change in frenquency (Hz) and n = selected overtone.
2.3
Surface characterization
The films were examined by high-resolution scanning electron microscopy (SEM) (LEO Ultra 55 FEG scanning electron microscope, Zeiss, Oberkochen, Germany) with an accelerating voltage of 5 KV and atomic force microscopy (AFM, XE-100, Park Systems Corp, Suwon, Korea). The SEM observations were performed on 2 discs of each group to evaluate pore direction of the mesoporous coatings. For the AFM measurements, a 10 μm × 10 μm scan area was selected and the images obtained subjected to leveling Gaussian filtering with a cut-off of 2.5 μm. The following 3-dimensional parameters were chosen to describe the surface topography: arithmetic mean roughness ( S a ), developed surface area ratio ( S dr ) and density of the summits per unit of sampling area ( S ds ). The mean value and standard error were obtained from 9 scans of each group, from random sites on the surface. 3-D reconstructions were obtained using MountainMap Universal 6.2 Surface Analysis Software, cropping each image to 1 μm × 1 μm area.
The chemical composition of the mesoporous surfaces with and without magnesium was analyzed with XPS, utilizing a Quantum 2000 scanning XPS microprobe (Physical Instrument, USA). The instrument was equipped with a monochromatic Al K α X-ray source with a beam size of 100 μm and take-off angle of 45°. The depth of the analysis was about 5 nm and the point diameter analysis was 100 μm. The analysis was performed on 5 samples from each group. Data are presented as atomic percentage (at%) of the elements.
2.4
Cell culture
Human fetal osteoblasts (hFOB 1.19, ATCC CRL-11372) were grown in calcium and magnesium-free DMEM/high glucose (1×) (Thermo Scientific) supplemented with 10% FBS, 4 mM L-glutamine and 1% penicillin/streptomycin solution in a humidified atmosphere of 5% CO 2 in air at 34 °C . At confluence, cells were treated with 3 mL of 0.05% trypsin-0.02% EDTA solution and seeded onto with the discs at a density of 10 4 /cm 2 /100 μL. 160 μL of the cell suspension was added to each disk and incubated for 30 min to allow early cell adhesion. Then 1 mL of fresh medium was added and cells were further cultured for 1 and 3 weeks at 37 °C, with medium renewal every 2–3 days.
2.5
Total mRNA isolation and quality control
All steps involved in RNA isolation were performed in an RNase-free environment. The instruments and working station were carefully wiped with RNase-ExituPlus (Panrease) to remove RNases. After 1 and 3 weeks total RNA was isolated using the Aurum™ total RNA fatty and fibrous tissue kit (Bio-Rad), following the spin format (column) protocol. Therefore, after cell lysis with 1.5 mL of PureZOL, 200 μL of chloroform was added to each lysate and incubated for 5 min at room temperature (RT). The mixture was centrifuged (Eppendorf 2420R) at 12,000 × g for 15 min at 4 °C, after which the colorless aqueous phase on the top of each column (RNA interphase) was transferred into a new tube and 600 μL of 70% ethanol added to each sample. Afterwards, 700 μL of the mixture was transferred to RNA binding columns and centrifuged for 30 sec at 12,000 × g . The filtrate was discarded and each column was then washed with 700 μL of LOW stringency wash buffer by centrifuging at 12,000 × g for 30 s. RNA binding columns were also treated with DNase I enzyme for 15 min to remove contaminating DNA. DNase digestion was followed by two washes, one with HIGH stringency wash buffer and one again with LOW stringency wash buffer, centrifuging each time at 12,000 × g for 30 s. The RNA columns were centrifuged additionally for 2 min to remove any residual wash solution. Each RNA column was then transferred to a new 1.5 mL tube and RNA eluted with 35 μL of elution solution, centrifuging at 12,000 × g for 2 min. Total RNA was quantified using a UV spectrophotometer (Thermo Scientific Nano Drop 2000).
2.6
Real-time RT-PCR analysis
An osteogenesis-related gene array (Osteogenesis SAB Target List H96 Predesigned panel with SYBR Green, Bio-Rad) was used to reveal genes where expression changed in relation to magnesium release from the three mesoporous titania films. From each RNA sample (1000 ng of RNA sample from each group), cDNA was generated using an iScript Advanced cDNA Synthesis kit for RT-qPCR (Bio-Rad) and loading samples into a RT-PCR thermocycler (Bio-Rad CFX Connect™ Real-Time System). A total of 20 μL of PCR mixture, which included 10 μL of 2× SsoAdvanced universal SYBR Green super mix, 9 μL of high-purity water and 1 μL of cDNA, was loaded into each well of the pre-designed array. The PCR run was set up with 95 °C as the activation temperature for 2 min followed by 40 cycles of 95 °C for 5 s and 60 °C for 30 s. Data were analysed using the comparative cycle threshold method with normalization of the raw data to 5 housekeeping genes: ACTB (β-actin), β2M, RPLP0, HPRT1 and GAPDH.
Subsequently, 4 genes selected from the PCR array analyses were validated by quantitative real-time RT-PCR using 350 ng of RNA sample in triplicate from each group. 0.5 μL of each primer for each selected gene (BMP2, BMP4, MMP9, TGFB1 and GAPDH) was mixed with 5 μL of 2x SsoAdvanced universal SYBR Green super mix and 3.5 μL of high-purity water to give a total of 10 μL of PCR mixture for each reaction ( n = 3). The PCR run was set up following the protocol described above using the same RT-PCR system.
2.7
Statistical analysis
Statistical analysis of the AFM 3-D parameters was conducted by applying a non-paramentric Kruskal–Wallis test, followed by a Mann–Whitney U test for pairwise comparisons (SPSS Inc., Chicago. IL, USA). RT-PCR data were subjected to Student’s t -test using CFX Manager™ Software Gene Expression Analysis.
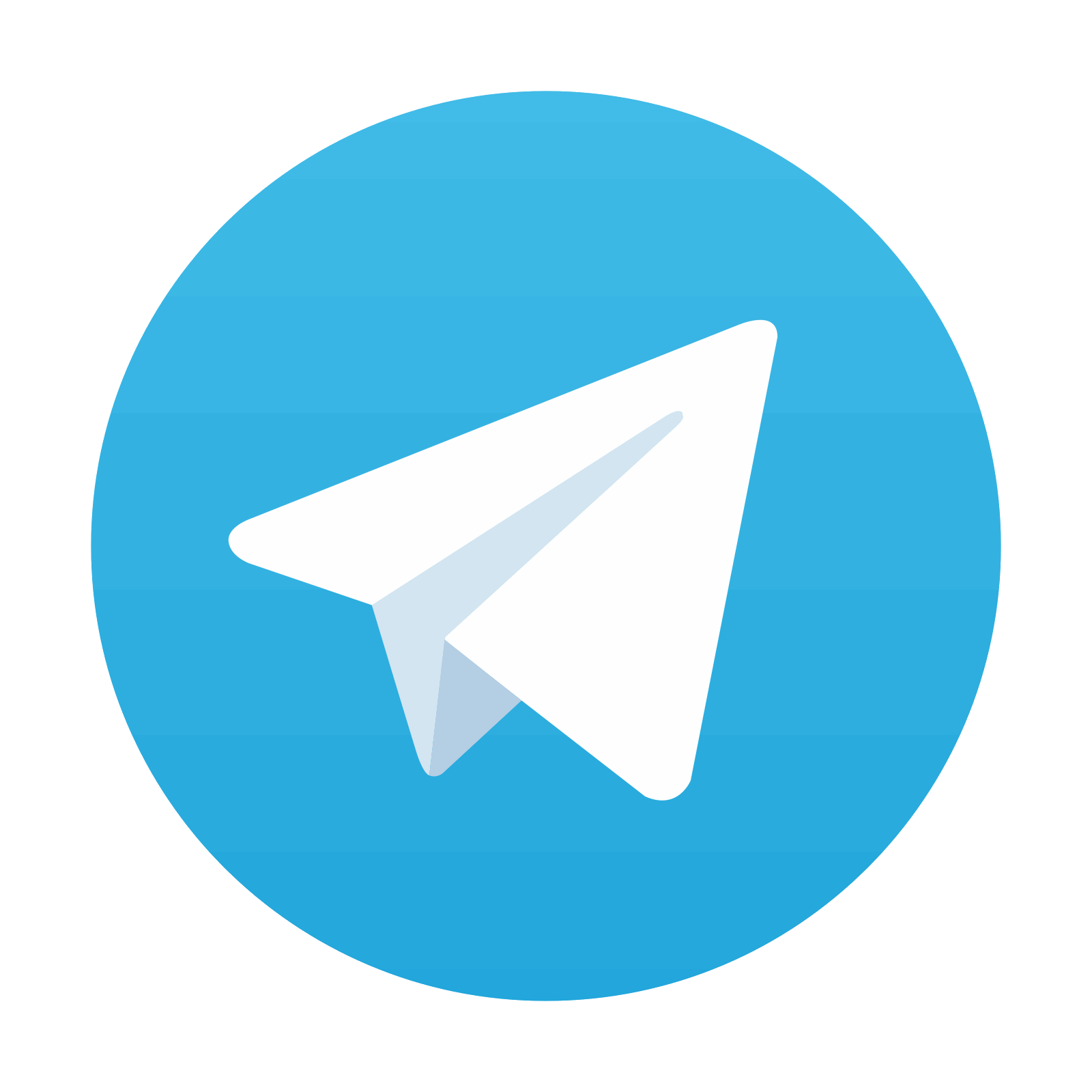
Stay updated, free dental videos. Join our Telegram channel

VIDEdental - Online dental courses
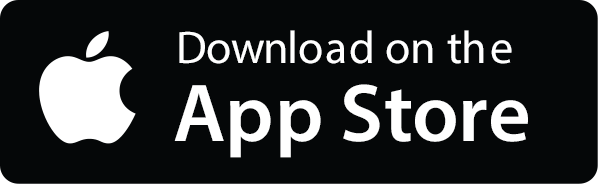
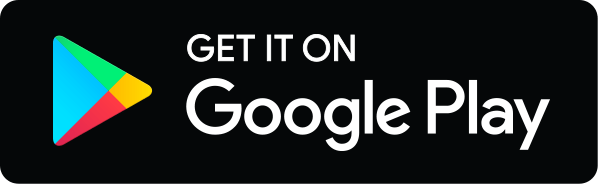