Abstract
Objective
One of the problems encountered clinically when using mineral trioxide aggregate (MTA) as a root-end filling material is washout immediately after placement. A novel MTA is supplied with an anti-washout gel that replaces the mixing water. The aim of this research was to characterize and assess the properties of a novel MTA mixed with an anti-washout liquid.
Methods
MTA Plus mixed with either water (MTA-W) or an anti-washout gel (MTA-AW) was investigated. Un-hydrated and set materials were characterized by scanning electron microscopy (SEM), energy X-ray dispersive analysis (EDX), X-ray diffraction analysis (XRD) and Fourier transform infrared spectroscopy (FT-IR) after being stored dry or immersed in Hank’s balanced salt solution (HBSS). The chemical and physical properties of the set materials were then investigated.
Results
The MTA Plus was composed of tricalcium silicate, dicalcium silicate and bismuth oxide. The anti-washout gel used was water-based and FT-IR plots showed the presence of an organic additive. Both materials immersed in HBSS displayed the presence of reaction by-product with MTA-W exhibiting a high-intensity calcium hydroxide peak on X-ray diffraction. The X-ray diffractograms of all materials following hydration demonstrated the reduction in peak intensity of the tri- and dicalcium silicate. Hydroxyapatite deposits were evident on the surfaces of both materials in contact with HBSS. The pH of the leachate was similar for both materials. MTA-AW exhibited lower levels of calcium ions in solution and reduced fluid uptake in the early stages of reaction. The anti-washout gel reduced the setting time of the cement and enhanced the compressive strength. The radiopacity of both materials was approximately 8 mm aluminum.
Significance
The use of the water-based anti-washout material instead of the standard water with MTA affects the hydration and properties of the set material.
1
Introduction
White mineral trioxide aggregate (MTA) is a dental cement with numerous applications including pulp-capping, apexification, repair of root perforations, root-end filling and others . One of the drawbacks of MTA is washout , which refers to the tendency of freshly prepared cement paste to disintegrate upon contact with blood or other fluids . Washout of a root-end filling material can occur when rinsing an osteotomy site resulting in a compromised root-end seal and its associated repercussions.
New-generation endodontic materials have been investigated as developments of MTA. Prototype cements Generex-A and Capasio have displayed superior washout resistance compared to MTA, whilst having similar compressive strength, radiopacity and alkaline pH , making them potentially suitable replacements. Washout-resistant injectable cements based on different material systems have also been investigated; injectable calcium-phosphate cement with the addition of sodium hyaluronate , sodium alginate chitosan or modified starch , were found to have good washout resistance. Whilst calcium phosphate is primarily indicated for use as a synthetic bone substitute, it has also shown potential as a dental cement .
MTA is composed primarily of Portland cement . Portland cement is used as a binder in concrete. Cements based on calcium silicates (the basic components of the Portland cement in MTA) have been shown to exhibit an increase in washout resistance by the addition of carboxymethyl chitosan or gelatin . In the construction industry, concrete employed in building underwater structures has historically had an anti-washout admixture (based on a water-soluble polymer) added to modify its rheological properties and make it more resistant to washout . Since both concrete and MTA use Portland cement as a binder , a similar admixture could be expected to impart the same enhanced washout resistance to MTA. A novel mineral trioxide aggregate (MTA Plus™, Prevest Denpro, Jammu City, India) which is claimed to have a finer particle size than the MTAs currently available for clinical use is provided with either water or an anti-washout gel . The gel is intended to improve its washout resistance , but little information about the properties of this new material is available in the literature.
An ideal anti-washout agent should (i) inhibit the decay of cement paste in liquid; (ii) not interfere with the hydration reaction or significantly reduce the bioactivity of the cement; (iii) not decrease the mechanical strength of the cement once set; (iv) not worsen the handling properties of the cement paste; (v) not significantly extend the setting time; and (vi) not reduce the radiopacity .
The purpose of this study was to characterize and investigate the chemical and physical properties of un-hydrated MTA Plus as well as set cements composed of MTA Plus mixed with the anti-washout gel (MTA-AW), and to compare these properties to MTA Plus mixed with only distilled water, as control (MTA-W).
2
Methodology
The materials used in this study included MTA Plus (compounded by Prevest Denpro, Jammu, India for Avalon Biomed Inc., Bradenton, FL, USA) lot #2011022801, mixed with either distilled water at a water to cement ratio of 0.35 or anti-washout gel (compounded by Prevest Denpro, Jammu, India for Avalon Biomed Inc., Bradenton, FL, USA). The gel was dosed by weight (0.350 g gel per gram MTA Plus powder).
The testing was performed at 1 day and at 28 days and the materials were stored either dry or immersed in Hank’s balanced salt solution (HBSS; H6648, Sigma Aldrich, St. Louis, MO, USA).
2.1
Characterization of un-hydrated cements, washout gel and set materials
2.1.1
X-ray diffraction analysis
Phase analysis was carried out on un-hydrated MTA Plus, the anti-washout gel and set cements cured in a sealed, dry container or immersed in HBSS for either 1 or 28 days using X-ray diffraction (XRD). The diffractometer (Rigaku Ultima IV, Rigaku Corporation Tokyo, Japan) used Cu Kα radiation at 40 mA and 45 kV. Samples were presented in powder form and the detector was rotated between 5° and 45°, with a sampling width of 0.05° and 2°/min scan speed. Phase identification was accomplished by use of search-match software utilizing the ICDD database (International Center for Diffraction Data, Newtown Square, PA, USA).
2.1.2
Fourier transform infra-red spectroscopy
Un-hydrated MTA Plus powder and the set cements were analysed using a Fourier transform infrared spectrophotometer (Shimadzu IRAffinity-1; Shimadzu Corp., Kyoto, Japan) using and ATR window diameter of 7 mm, spectral resolution of 4 cm −1 and 45 scans per spectrum. The anti-washout gel was first dried for 48 h in an incubator at 90 ± 1 °C to produce a thin uniform sheet of dry polymer. A portion of the sheet was then cut out with scissors, loaded into the spectrophotometer and analysed in a similar way to the un-hydrated MTA powder.
2.1.3
Chemical analysis of anti-washout gel
The chemical analysis of the anti-washout gel was performed using Energy Dispersive X-ray Fluorescence (EDXRF; Bruker S2 Ranger, Bruker Corporation, Madison USA) using water as the matrix and 4 μm liquid prolene film.
2.1.4
Scanning electron microscopy of hydrated cements
Cement cube specimens of side 7 mm, stored dry or immersed in HBSS for 28 days at 37 ± 1 °C, were analysed using scanning electron microscopy (SEM). Microstructural analysis was performed with X-ray energy dispersive analysis (EDX). At the end of the curing period the materials were desiccated and embedded in epoxy resin (Struers Epofix, Struers, Ballerup, Denmark). This was followed by grinding them with progressively finer grits of abrasive paper, polished with diamond paste, washed with isopropanol alcohol, dried and carbon coated (Agar auto carbon coater; Agar Scientific, Essex, England). The materials were viewed under the scanning electron microscope (SEM; Zeiss MERLIN Field Emission SEM, Carl Zeiss NTS GmbH, Oberkochen, Germany) and cement microstructure was assessed in backscatter electron mode. EDX analysis was performed on each phase identified. In addition, plots of calcium–silicon ratios of the cement particle center, the margin of the cement particle and the cement matrix were plotted. The bismuth to calcium ratios of these areas in relation to the bismuth particle were also plotted. This was repeated for cubes stored in HBSS.
In addition, cement discs 15 mm in diameter and 1 mm thick were cured for 24 h as described, immersed in HBSS for 28 days, dried and finally carbon coated without polishing the surfaces. The unpolished surfaces were then observed under the scanning electron microscope.
2.2
Chemical properties
2.2.1
pH and calcium ion release in physiological solution
Discs of diameter 15 ± 0.1 mm and height 2 ± 0.5 mm were cast and allowed to cure for 24 h in a dry incubator at a temperature of 37 ± 1 °C. They were then immersed in individual sealed polycarbonate containers containing 20 ± 0.01 ml of HBSS. Three replicate samples of each material were made.
Using a pH/mV/ISE meter (Hanna HI 3221, Hanna Instruments, Woonsocket, RI, USA) with a single-junction (Ag/AgCl) ceramic pH electrode (Hanna HI 1131, Hanna Instruments, Woonsocket, RI, USA) the pH of each solution was measured after 1, 7, 14, 21 and 28 days had elapsed from the moment of immersion. Temperature compensation was accomplished by simultaneously immersing a temperature probe (HI 7662, Hanna Instruments, Woonsocket, RI, USA) in the measurement solution. The pH meter was calibrated using three standard calibrating solutions (pH 4.01, 7.01 and 10.00) prior to each set of measurements.
The calcium ion concentration in solution was measured at 1, 7, 14, 21 and 28 days using an ISE Calcium Electrode (consisting of Hanna HI 4000-50 Sensor handle and Hanna HI 4004-51 calcium module) and a reference electrode (Hanna HI 5315). Temperature compensation was accomplished by immersing a temperature probe (HI 7662, Hanna Instruments, Woonsocket, RI, USA) in the measurement solution simultaneously. The meter was calibrated using two standard calibrating solutions (100 ppm and 1000 ppm) prior to each set of measurements.
2.3
Physical properties
2.3.1
Radiopacity
Cement discs of diameter 10 ± 0.1 mm and height 2 ± 0.5 mm were prepared and incubated for 24 h at 37 ± 1 °C to allow the cement to cure, after which they were either stored in a dry sealed container, or immersed in HBSS inside a sealed container, in each case at 37 ± 1 °C. Radiographs were taken after one day and after 28 days under these conditions. Three replicates of each test were made.
To take the radiographs, the specimens were taped to a plastic sleeve containing a photostimulable phosphor (PSP) plate of the same size. An aluminum step-wedge (Everything X-ray, High Wycombe, UK) with steps of incremental thickness 3 mm, was placed beside the discs covering part of the sleeve such that it appeared in the radiographs alongside the specimens. The samples and the wedge were then irradiated with X-rays using a dental X-ray machine (GEC Medical Equipment Ltd., Middlesex, UK) using an exposure time of 0.4 s, tube current and voltage of 10 mA and 65 kV respectively, and a cathode-to-target film distance of 400 ± 10 mm.
The radiographs were processed in an automatic processing machine (Clarimat 300, Gendex Dental Systems, Medivance Instruments Ltd., London, UK) and a digital image of the radiograph was obtained. The radiograph was in the form of a greyscale image file. The radiopacity (measured in millimeters of aluminum), denoting the thickness of aluminum required to block X-rays to the extent of one millimeter thickness of the cement under test, was calculated using the method described in a previous publication . Briefly, an image manipulation program (Microsoft Paint; Microsoft Corp., Redmond, WA, USA) was used to determine the gray pixel values (a measure of the “shade of gray” or “color”) of the steps of the step wedge. A spreadsheet program (Microsoft Office Excel 2003; Microsoft Corp., Redmond, WA, USA) was used to plot a chart of gray pixel value vs. thickness of aluminum, and the best-fit exponential trendline was drawn through the points. The equation of this trendline was then used to calculate the radiopacity of the cement discs from their gray-pixel values on the same radiograph.
2.3.2
Setting time
Setting time was evaluated using the procedure set out in ISO 9917-1:2007 . The cement pastes were packed into stainless steel moulds with internal cross-section 10 mm by 8 mm and depth 5 mm. A stopwatch was started and the moulds were immediately placed in an incubator at 37 ± 1 °C, either in air, or immersed in HBSS.
Testing for setting was done using a modified Vicat apparatus, consisting of a weighted needle of square cross-section of side 1 ± 0.01 mm with a total mass of 400 ± 5 g. The cement was considered to have set when the needle was lowered gently onto the cement surface and did not leave a complete square indentation on it. The cement was tested for setting initially at 15 min time intervals, gradually shortening to around 1 min intervals as time progressed and the cement was visibly close to being completely set.
2.3.3
Compressive strength
Two-part cylindrical brass moulds with internal diameter 4 ± 0.1 mm and length 6 ± 0.1 mm were used. The moulds were placed on a glass plate and lubricated with mould release agent (Sika Separol, Switzerland). The cement was then compacted into each mould using a spatula, after which it was further compacted using a dental plugger to ensure a dense uniform sample with minimal porosity. Once filled, the excess was scraped off with the edge of a glass microscope slide to leave a flat uniform surface.
The filled moulds were incubated for 24 h at 37 ± 1 °C, after which they were removed from the incubator and the screws holding the two halves together were loosened to allow the set cement cylinder to be gently pushed out without damaging it. The cylinders were then left for 28 days in either a sealed, dry container, or immersed in HBSS contained in a sealed container, in each case in an incubator kept at 37 ± 1 °C. 10 replicates of each test were made.
After 28 days had elapsed, the specimens were taken out of the solution and dried using filter paper. The top and bottom were lightly sanded using moistened 600-grit sandpaper and each sample’s diameter was measured with a digital micrometer (Draper PM025D, Draper Tools Ltd., Hants, UK). They were then individually loaded with a compressive strength testing machine (Controls 50-C0050/CAL, Controls spa, Milan, Italy) at a loading rate of 50 ± 10 N/min as per ISO 9917-1:2007 , until failure of the cylinder occurred. The compressive loading before failure was recorded and the compressive strength of each cylinder calculated using Eq. (1) , which assumes specimens of perfectly circular cross-section:
σ = Maximum force applied before specimen failure Cross sectional area of specimen = F × 4 π d 2
where σ , compressive strength (MPa); F , maximum force applied before failure (N); d , diameter of specimen cylinder (mm).
2.3.4
Micro-hardness
Specimens 15 ± 1 mm diameter and 1 ± 0.1 mm thick were prepared and allowed to cure for 24 h at 37 ± 1 °C. The specimens were then removed from the moulds and immersed in 20 ± 0.001 ml of HBSS. After immersion in HBSS for 28 days the specimens were dried in a desiccator. The surface of each disc was ground using progressively finer grits of silicon carbide paper, from 180-grit to 1200-grit, and finally polished using 3-micron polycrystalline diamond paste. The polished discs were sprayed with a thin layer of graphite spray (Graphit 33, CRC Industries, Iffezheim, Germany) to aid visibility under the indenter.
Measurement of micro-hardness was performed with a Micro-Vickers hardness-testing machine (MVK-H2, Mitutoyo Asia Pacific Ltd., Kanagawa, Japan) using a Vickers diamond indenter with 100 gf load (equivalent to 0.9807 N). The machine automatically applied the load, held it for 10 s and released it, with the loading and unloading rates being automatically controlled. The length of the two diagonals of the impression left by the indenter were measured, and Vickers hardness was calculated using Eq. (2) :
where HV , Vickers hardness number; 0.1891, Vickers constant (from indenter geometry); F , force in Newtons (=0.9807 N for the 100 g weight used); d , arithmetic mean of the two diagonals, in mm.
2.3.5
Fluid uptake, sorption, solubility and estimated porosity by mass measurement
Specimens 15 ± 0.1 mm diameter and 1 ± 0.1 mm thick were cast by placing the pastes in the moulds and allowing them to cure for 24 h at 37 ± 1 °C. The specimens were then removed from the moulds and weighed to an accuracy of ±0.0001 g. This initial dry mass was recorded as m 1 . The mean diameter of each specimen and the thickness of each specimen were measured to an accuracy of ±0.01 mm used to calculate the volume V of each disc. The specimens were then immersed in 20 ± 0.001 ml of HBSS.
After 1 day of immersion, the specimens were removed, dried using filter paper and weighed (while they were still fully saturated with fluid). This mass was recorded as m . The fluid uptake, F up of each specimen (a measure of the amount of fluid taken up by the specimens, expressed in μg/mm 3 ) was calculated using Eq. (3) and repeated to measure the fluid uptake after 1, 7, 14, 21 and 28 days of immersion
where m is the mass, in micrograms, of the fluid-saturated specimen at 1, 7, 14, 21 or 28 days; m 1 is the mass, in micrograms, of the specimen prior to immersion in fluid (i.e. at day zero); V is the volume of the specimen, in cubic millimeters.
After 28 days, the mass of the specimens (fully saturated with fluid) was measured and recorded as m 2 . The specimens were then dried by placing in a desiccator under vacuum for 24 h, using silica gel as desiccant, to constant mass as defined in the standard . This final dry mass was recorded as m 3 .
The sorption, F sp , in micrograms per cubic millimeter, for each sample was calculated using Eq. (4) .
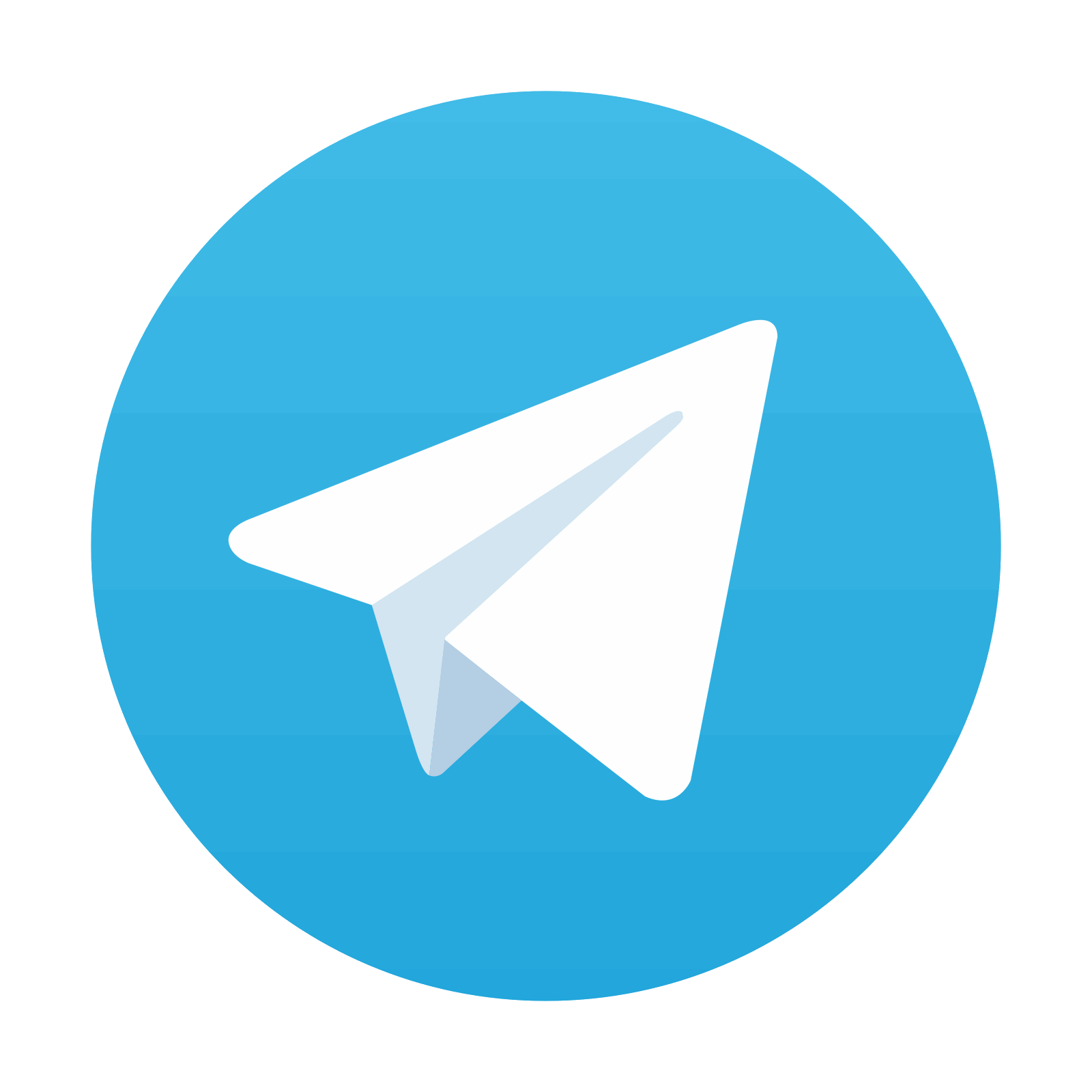
Stay updated, free dental videos. Join our Telegram channel

VIDEdental - Online dental courses
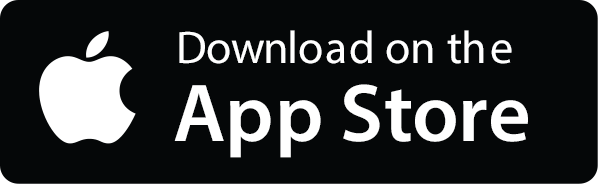
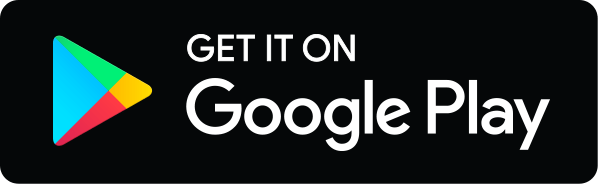