3
Microbial Biofilms in Root Canal Systems
Luis E. Chávez de Paz
Summary
Endodontic infections are caused by microbial biofilm structures that are established on the surfaces of the root canals of teeth. Biofilm removal from the root canal systems is considered essential to successfully achieve healing of inflamed periapical tissues. One of the main characteristics of root canal biofilms is the occurrence of recalcitrant phenotypes among biofilm bacteria that have the potential avoid clearance by mechanical removal and antimicrobial treatments. Although most of these phenotypic adaptive characteristics are known to reduce the effectiveness of clinical treatments, most of them are not very well known. Thus, there is an urgent need to understand the microbial processes that benefit microbial persistence in infection-associated diseases. By understanding the methods of phenotypic adaptation, new clinical strategies to increase the effectiveness of current antimicrobial methods can be achieved, as well new antimicrobial strategies developed for use in Endodontics.
3.1 Introduction
Microbial biofilms are sessile communities of microorganisms that are well-organised and attached to coated surfaces [1, 2]. Commonly, biofilms are organised in multiple layers of cells embedded in a matrix of self-produced extracellular polymeric substances (EPSs) [1, 2]. In biofilms, microorganisms have a greater resistance to antimicrobials compared to cells floating in liquid (planktonic form) [3, 4]. This enhanced resistance to antimicrobials is one of the most clinically relevant features of biofilms and has been the matter of intense research for decades [3, 4].
Two main factors have been found to be of utmost importance underlying the antimicrobial resistance of biofilms: the physical protection provided by the polymeric matrix [5, 6], and the increased phenotypic plasticity of biofilm microorganisms [7]. In relation to the latter factor, plasticity is mainly characterised by the generation of biofilm cells with suspended or delayed growth patterns and/or cells that efficiently regulate the expression of stress-induced proteins [8]. Phenotypic plasticity is considered critical to the adaptation and survival of biofilm cells and plays a role in the recalcitrance (resistance) of biofilms to common antimicrobial therapy.
Microbial biofilms formed in root canals of teeth share basically the same structural characteristics of biofilms formed in different parts of the human body as observed in microscopy studies [9–11]. Biofilm structures have been observed on root canal walls, within dentinal tubules and apical deltas, and attached to the periapical cementum [9–11]. Research in this area has determined that microbial biofilms formed in root canals play a role in persistent apical periodontitis [9–11]. These refractory endodontic infections are caused by biofilm communities that harbour microbial phenotypes that can, for example, adapt to harsh environmental conditions in root canals [9]. In root canal biofilms, microorganisms can also survive for long periods of time by regulating their metabolism and significantly reducing their energy consumption [12]. Although these regulatory pathways have not been clarified completely, it is now clear that root canal biofilm bacteria will use these mechanisms to resist and survive [2, 12, 13]. This chapter addresses the main characteristics of microbial biofilms, formation of biofilms in root canals of teeth, and the ecological parameters that are key to understand phenotypic adaptation of biofilms and their resistance to antimicrobials.
3.2 General Characteristics of Microbial Biofilms
Biofilms are complex biological structures where bacteria are embedded and attached to natural or artificial surfaces. The nature of biofilms relies on the affinity of bacteria to adhere to surfaces and to live in multispecies communities. Biofilm microbial communities form on every surface that is exposed to liquids containing microorganisms [1]. The most common example of biofilm communities continuously formed on human surfaces is dental plaque. In biofilms, bacteria are organised heterogeneously and are encased in a self-produced matrix [5]. The heterogenous nature of biofilms provide bacteria with a wide range of advantages and possibilities; for example, internal regulatory processes are triggered in biofilm bacteria when exposed to host immunological defenses and antibiotics [14]. These wide range of regulatory processes in stressed biofilm bacteria have been widely studied and have determined the plasticity of biofilm bacteria to adapt their physiology to resist stress [4, 6, 14].
3.2.1 How do Bacteria Build Biofilms?
Commonly, the formation of any biofilm follows the same five steps:
- Free-floating cells come in contact with a surface pre-coated with substances from the environment.
- Bacterial cells establish an initial association with the pre-coated surface, usually by structures such as fimbriae and/or flagella [15].
- The initial association between the initial colonisers and the surface becomes firmer with time and enables co-adhesion of secondary colonisers leading to the formation of microcolonies [16].
- Maturation of microcolonies in biofilms are sustained by the production of an extra-cellular matrix that is mainly composed by self-expressed EPSs, polysaccharides, proteins, nucleic acids, and phospholipids [17, 18].
- Finally, micro-colonies develop into three-dimensional structures covering the entire surface.
The general steps in biofilm formation are shown in Figure 3.1.

Figure 3.1 Depiction of the stages of biofilm formation. (a) Surfaces are coated with environmental molecules. (b) Initial colonizers adhere to the coated surface. (c) Secondary colonizers co-adhere to adhered pioneers using different cell-surface adhesins. (d) Adhered bacteria form micro-colonies that grow mature biofilms by producing extracellular polymeric substances (matrix) leading to the development of complex biofilm communities.
3.2.2 Formation of Biofilms in Root Canals
When they become infected, microorganisms form biofilms in all extensions of root canals. Biofilm structures have been found in the main root canal and accessory canals [10, 11]. Root canal biofilm formation has been hypothesized to follow the same steps as their general biofilm counterparts, e.g. bacterial cells in a planktonic phase initially become attached to pre-coated dentinal walls and subsequently form microcolonies [19]. It is possible that root canal biofilm formation begins shortly after oral organisms invade the pulp chamber using the pulp tissue inflammatory breakdown as a fluid vehicle to colonise deeper areas of the root canals [19]. Light microscopy studies have confirmed this hypothesis where micro-organisms have been observed embedded in the pulpal inflammatory masses in necrotic pulps. These observations have shed light on the significance of the inflammatory lesions, which act as vehicles in bacterial colonisation of apical areas of root canals.
3.2.3 Planktonic Versus Biofilm Lifestyles
Based on current knowledge, bacteria inhabit natural environments in two different states: a planktonic phase (free-floating in a liquid substrate) and in colonies adhered to surfaces (biofilms state). The planktonic phase is mainly considered artificial. It has been profoundly studied under highly controlled laboratory conditions, e.g. microbial cultures growing in test tubes filled with specific growth medium [20]. However, in nature, the planktonic phase is considered transient as it poses highly variable environmental conditions that trigger bacteria to quickly adapt their phenotype for survival [20]. These rapid phenotypic adaptations in planktonic cells are often achieved through intense metabolic adaptations, which most of the bacteria cannot cope with and perish upon exposure to environmental stressors [21]. With the advent of current microbiological technologies, it has been revealed that the phenotype of bacteria in the planktonic phase is significantly different from that of the same organism attached to surfaces in biofilms [21, 22].
In biofilms, bacteria experience phenotypic changes from the moment they come in contact with surfaces. In a regulatory process called ‘surface signal,’ several cellular mechanisms are triggered that help bacteria attach to surfaces, e.g. expression of cell membrane receptors and the development of binding structures such as flagella [23, 24]. Flagella expressed in newly attached bacterial cells have a mechanosensory role that is critical in the initial stages of biofilm formation [23, 24]. This mechanosensory mechanism of flagella initiates a cascade of intracellular events leading to the biofilm phenotype (Figure 3.2).

Figure 3.2 Depiction of the phenotypic differences between the planktonic and the biofilm lifestyles in bacteria. Phenotypic changes occur in bacteria in the (a) planktonic phase in response to approximation to a coated surface. In the process of (b) surface sensing, bacterial appendices, e.g. flagellum, trigger the expression of several intracellular factors that will facilitate the attachment to coated surfaces. The phenotypic changes in surface-attached bacteria will provide specific characteristics to bacteria leading to the (c) biofilm phenotype.
3.2.4 The Biofilm Phenotype
The biofilm phenotype is characterised by the regulation of several mechanisms in biofilm cells that adjust their physiology to tolerate environmental changes [13]. These phenotypic regulatory pathways allow biofilm bacteria to survive for long periods of time, a trait that may shed light on the maintenance of chronic infection such as those provoked in the periapical tissues. One of these mechanisms in the biofilm phenotype is the one regulating the amount of energy required for survival. In a process called dormancy [25], biofilm cells enter a ‘lag phase’ that restricts their nutritional demands and adapts their physiology to low nutritional needs. Studies on biofilm have shown that by entering these low-metabolic states, biofilm cells become more tolerant to antimicrobials.
Among bacteria isolated from infected root canals, these nutrient-adaptive capabilities have been shown in ex vivo experiments [12, 26, 27]. In the strict saccharolytic organism Streptococcus oralis, an essential number of proteolytic enzymes have been found to be up-regulated upon depletion of carbohydrates in the media. This nutritional plasticity of biofilm cultures of S. oralis to metabolise proteins in the depletion of carbohydrates is a clear example of phenotypic adaptation of biofilm cells at the times of substrate depletion. Of interest are the complementary patterns exerted by similar saccharolytic oral bacteria in biofilms that have the ability to degrade glycoproteins in salivary mucins when present as the only source of nutrients [27]. In a similar way, plasminogen from serum is degraded by oral bacteria in biofilms. The latter is a clear example of how bacteria in root canal biofilms can adapt their nutritional demands upon nutrient depletion with environmental changes provoked after pulp inflammation and necrosis [26].
Another interesting trait of biofilm phenotypes and that has been found to have a strong correlation among bacteria isolated from infected root canals is the ‘stringent response’ [28, 29]. The stringent response is a stress-regulator mechanism that encloses a switch in the transcriptional profiles of biofilm bacteria [28, 29]. This nutritional regulation is controlled by an alarmone system composed by guanosine tetraphosphate (ppGpp) and guanosine pentaphosphate (pppGpp) [30, 31]. In Enterococcus faecalis strains isolated from infected root canals, the alarmone system has been found to play a role in low-nutrient survival and biofilm maturation [30]. An improved understanding of the alarmone system (p)ppGpp in root canal biofilm bacteria may shed light as to how bacteria can withstand long periods of famine in treated root canals and lead to alternative mechanisms of treatment for such chronic infections [32].
3.3 Ecological Factors Affecting Biofilms in Root Canals
The root canal microbiota has been widely described in terms of the frequency that certain species are found in different clinical scenarios. However, we still lack a clear understanding of the fundamental ecological processes that microorganisms endure while invading and establishing in root canals of teeth. As proposed by Chavez de Paz and Marsh [33], three main ecological processes occur in root canals after microbial invasion. The first process encloses two ecological scenarios that act as a habitat filter selecting for specific microorganisms: a scenario of pulpal inflammation and pulp necrosis. The second process is triggered after root canal treatment, where the use of antibacterials and medicaments cause stressful environmental conditions that selects for more resistant microorganisms. The third ecological process comprises the resilience of microorganisms surviving root canal treatment. This last process is characterised by the multiple factors used by microorganisms in order to adapt and survive in the limiting post-disturbance ecological scenario.
3.3.1 The Inflammatory and Necrotic Environments
In healthy conditions, dental pulp tissue is located within a sterile environment and encased in hard tissue barriers that protect it from the oral microflora. Upon bacterial invasion into the pulp chamber, a well-organised first line of defence system effectively responds by signalling, activation, and subsequent migration of neutrophils to the site of invasion [10, 34, 35
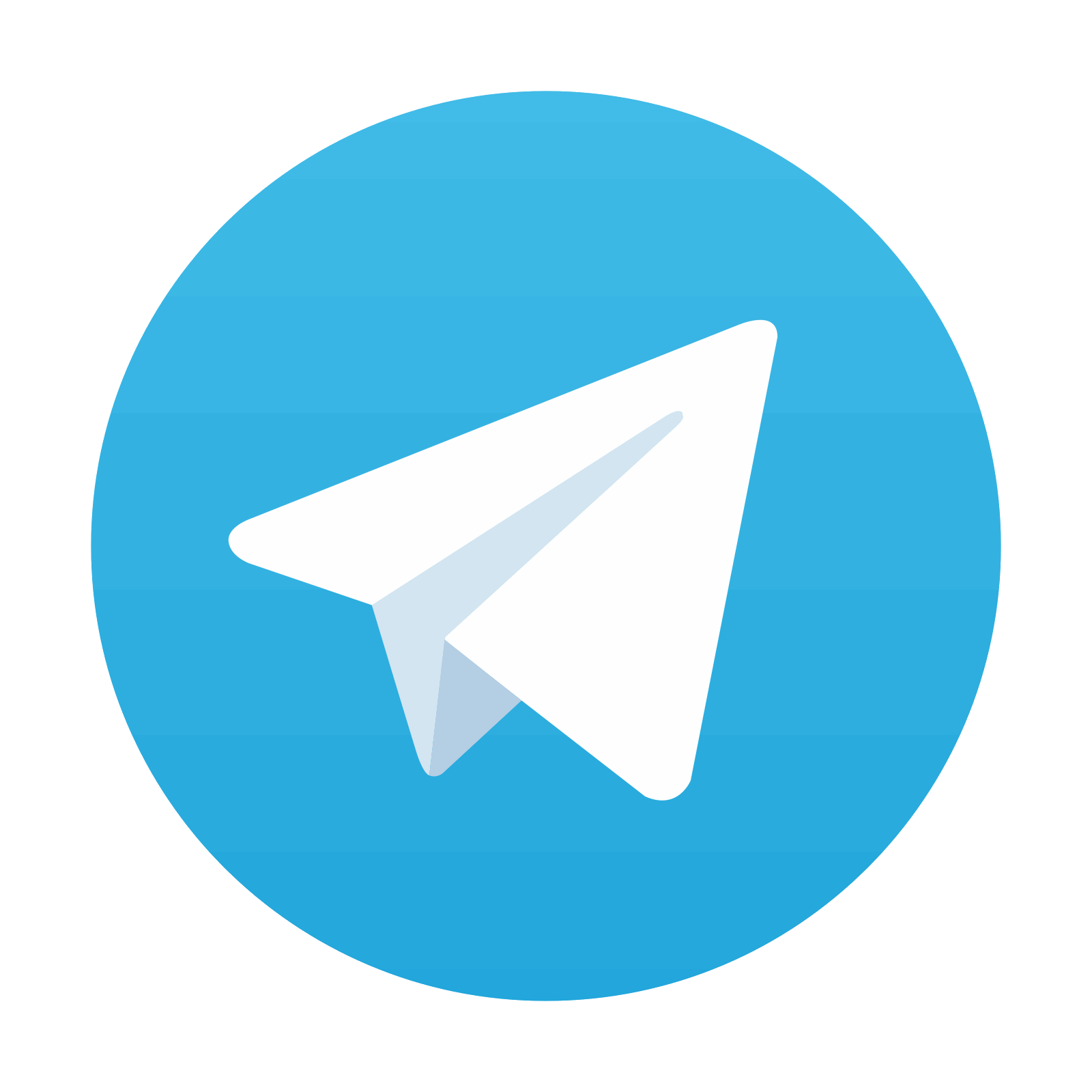
Stay updated, free dental videos. Join our Telegram channel

VIDEdental - Online dental courses
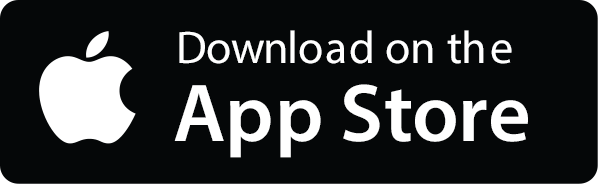
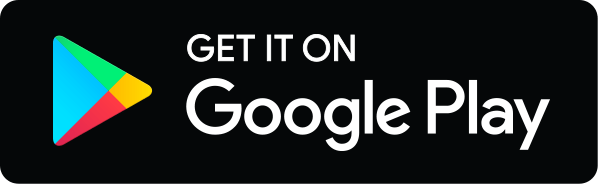