Abstract
The primary aims of orbital floor reconstruction are to prevent enophthalmos and herniation of the orbital contents in order to achieve correct globe position. Theoretically, the mechanical load of the orbital floor is approximately 0.0005 N/mm 2 (30 g orbital content onto 600 mm 2 of orbital floor area). Therefore, low mechanical stress from orbital floor reconstruction materials is expected. The periorbita and orbital floor complex (bony orbital floor with periorbita) of 12 human cadavers were investigated for their mechanical resistance to distortion and compared to different absorbable pliable reconstruction materials after modification with pores (Bio-Gide, Creos, and PDS). The human periorbita resistance (approximately 1.4 N/mm 2 ) was comparable to that of the absorbable membranes (Creos, Bio-Gide), and the resistance of PDS (approximately 2.3 N/mm 2 ) was comparable to that of the orbital floor complex. The periorbita has a higher stability than the bony orbital floor. Therefore, in isolated orbital floor fractures with a traumatized bony orbital floor and periorbita, reconstruction of the soft tissue as a periorbita equivalent with a resorbable membrane appears to be adequate to prevent enophthalmos and herniation of the orbital contents.
Isolated orbital floor fractures are the result of forces on the orbital globe, on the infraorbital rim, or on a combination of both. These clinical fractures result in both soft tissue (periorbita) and hard tissue (bony orbital floor) trauma. The periorbita and the bony orbital floor together comprise the orbital floor complex. Herniation of the peri-orbital tissue, fat, the inferior rectus muscle, and the periorbita leads to the clinical signs of motility disorders, diplopia, and enophthalmos. Paresthesia of the cheek and the teeth depends on the trauma to the infraorbital nerve, which runs along the lateral part of the orbital floor. Revision of the orbital floor and repositioning of the herniated soft tissues are the most common treatments.
However, consensus is lacking in the literature regarding adequate reconstruction materials. Several materials have been tested and described in clinical use. The most important issues in reconstruction are optimizing the globe position in relation to that before the fracture and achieving unrestricted function of the inferior rectus muscle, especially after healing. Moreover, complications triggered by reconstruction materials should be minimized. The most common reconstruction materials are titanium meshes, polydioxanone, and polyglactin. The use of these materials may result in complications, migrations of the material, inflammation, adherence of the orbital contents, or retrobulbar haematoma. Retrobulbar haematoma may be reduced easily with the use of perforated materials to increase drainage into the sinus. Other complications may be reduced by the use of adequate degradable reconstruction materials.
There is still a lack of data regarding the mechanical stress of incorporated reconstruction materials. Theoretically, the mechanical load of the orbital floor is approximately 0.0005 N/mm 2 (30 g orbital content onto 600 mm 2 of orbital floor area), as indicated by several cadaver investigations. Therefore, low mechanical stress is expected from orbital floor reconstruction materials. With a better understanding of mechanical stress and anatomical prerequisites, new materials could be developed, or existing materials could be customized based on biomechanical requirements. Therefore, the mechanical resistance to distortion of human cadaver periorbita and the orbital floor complex (assessment of the anatomical orbital floor complex) were investigated in this study and the data compared to those of absorbable reconstruction materials.
Materials and methods
Twelve sagittally split human cadaver heads (fixed in alcohol solution) prepared for academic courses in the Department of Anatomy at Christian-Albrechts University Kiel were used for this investigation. Within 24 h after death, the cadavers were fixed in alcoholic solution by perfusion. The cadavers were used for 2 years in anatomy courses and were stored in alcoholic solution during this period. Unfortunately, the ages and sexes of the heads used in this study could not be determined. The heads were first freed of soft tissue, with the exception of the periorbita and the infraorbital nerve. Specimens of periorbita measuring approximately 15 mm × 15 mm were harvested from the lateral orbit ( Fig. 1 ). Then, the orbit and the maxilla were separated from the remaining bony parts of the skull and the orbital roof was removed.

Two collagen membranes, Bio-Gide (Geistlich, Wolhusen, Switzerland) and Creos (Nobel Biocare, Cologne, Germany), were modified by the introduction of pores of 2.0 mm in diameter, with 3.0 mm between pores. For this purpose, the pore pattern of the PDS foil (ZX8, 0.15 mm; Ethicon, Norderstedt, Germany) was used as a matrix and was copied with a felt pen onto the collagen membranes. A biopsy punch was used to produce the pores in the membranes (BP-20F, diameter 2.0 mm; Kai Medical Europe GmbH, Solingen, Germany) ( Fig. 2 ).

To evaluate the puncture strength of the periorbita, collagen membranes, PDS foil, and orbital floor complex, a Zwick Z010 TN1 universal testing machine was used (Zwick GmbH, Ulm, Germany) ( Fig. 3 A, B ). A height adjustable punch (diameter 5.5 mm; 23.758 mm 2 ) was connected to a high-definition force transducer. The initial position (200.0 mm above the tested material), first testing position (approximately 2.0 mm above the specimen), and final position of the punch (10.0 mm behind the surface of the material) were set by software (testXpert II; Zwick GmbH). The force transducer was calibrated prior to each experiment. The punch had a continuous speed of 2.0 mm/min after starting the test procedure. The periorbita specimens and the membranes were fixed between two metal plates with a hole for the testing punch ( Fig. 3 C). The bony specimens of the orbital floor complex were fixed with kneadable silicone (Optosil Comfort Putty; M + W Dental, Büdingen, Germany) to ensure stability ( Fig. 3 D). After the membranes/tissues were centred under the punch, the testing procedure was started. Twelve specimens were tested for each group. The measurements were recorded in a series of graphics. In addition, the maximum value of mechanical resistance to distortion was calculated.

Statistical analysis
First, the mechanical resistance data were tested for standard deviation with the Kolmogorov–Smirnov test. Data were normally distributed ( P = 0.209), thus a t -test was used in the statistical analysis. The statistical analyses were performed with WinSTAT software (R. Fitch Software, Bad Krozingen, Germany) as an add-in to Microsoft Excel.
Results
The values of the mechanical resistance to distortion of the tested materials are summarized in Table 1 . There was no statistically significant difference between the mechanical resistance of the periorbita and that of the absorbable membranes (Bio-Gide, P = 0.91; Creos, P = 0.70). The values were all close to approximately 1.4 N/mm 2 . There was also no statistically significant difference between the puncture strength of PDS foil and that of the orbital floor complex (bony orbital floor with periorbita) ( P = 0.54). Both values were approximately 2.3 N/mm 2 . However, there were significant differences in resistance between the periorbita and the orbital floor complex ( P = 0.03), between PDS foil and the periorbita ( P = 0.02), between the orbital floor complex and Creos ( P = 0.003), and between the orbital floor complex and Bio-Gide ( P = 0.008).
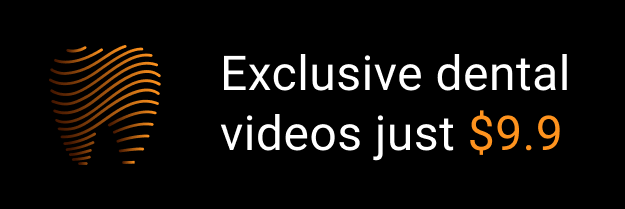