Abstract
Objectives
To determine and identify correlations between flexural strength, strain at failure, elastic modulus and hardness versus ceramic network densities of a range of novel polymer-infiltrated-ceramic-network (PICN) materials.
Methods
Four ceramic network densities ranging from 59% to 72% of theoretical density, resin infiltrated PICN as well as pure polymer and dense ceramic cross-sections were subjected to Vickers Indentations (HV 5) for hardness evaluation. The flexural strength and elastic modulus were measured using three-point-bending. The fracture response of PICNs was determined for cracks induced by Vickers-indentation. Optical and scanning electron microscopy (SEM) was employed to observe the indented areas.
Results
Depending on the density of the porous ceramic the flexural strength of PICNs ranged from 131 to 160 MPa, the hardness values ranged between 1.05 and 2.10 GPa and the elastic modulus between 16.4 and 28.1 GPa. SEM observations of the indentation induced cracks indicate that the polymer network causes greater crack deflection than the dense ceramic material. The results were compared with simple analytical expressions for property variation of two phase composite materials.
Significance
This study points out the correlation between ceramic network density, elastic modulus and hardness of PICNs. These materials are considered to more closely imitate natural tooth properties compared with existing dental restorative materials.
1
Introduction
The use of dental CAD/CAM-systems in combination with CAD/CAM-machinable materials enables the esthetic demands of prosthetic restorations to be achieved. Besides esthetic outcomes, complementary superior physical properties are needed for permanent dental restorations. Regarding non-metal CAD/CAM materials for permanent dental restorations there are currently two main groups, ceramics and composites . The ceramic group is subdivided into polycrystalline and glass ceramics. The composites are subdivided in macro-, micro-, hybrid-filled- or nano-composites . Ceramics tend to be more rigid and brittle, while composites are more compliant, soft and experience high wear. The ideal goal for restorative dentistry would be to replace lost tooth substance by a restorative material with tooth like structure and matching physical properties. Toward this objective a novel material that attempts to emulate the properties of natural teeth in its structure and physical properties was developed and named polymer-infiltrated-ceramic-network material (PICN). The goal is to achieve a material with enhanced mechanical characteristics, compared to conventional restorative materials like ceramics and composites.
PICNs can be classified as interpenetrating phase composites (IPC). IPCs have a three-dimensional interconnected geometry. A ceramic interpenetrating material was introduced to dentistry more than two decades ago, known as the In-Ceram system (Vita Zahnfabrik, Bad Saeckingen, Germany). In-Ceram materials are based on a porous ceramic structure with a glass interpenetrating phase . The combination of ceramic-metal IPC has been reported by Horvitz et al. , who outlined the advantages of the ceramic matrix (Al 2 O 3 ) as, for example, enhanced wear resistance, high temperature strength etc., and the fact that the metallic phase improves toughness by crack bridging.
Due to the presence of two connected phases within IPCs, crack propagation is generally limited because of interfacial crack deflection. The phase with the higher strain to failure enhances the fracture resistance by bridging the cracks introduced to the other phase .
Compared to In-Ceram the brittle glass phase is replaced by a polymer to form the PICN. In contrast to traditional composites which consist of one continuous phase filled with inorganic particles, PICN consists of two continuous interpenetrating networks. One network is a ceramic material (feldspar, light gray areas in Fig. 1 ) and the other a polymer (commonly used methacrylates for dental applications, dark gray areas in Fig. 1 ).

The anticipated improvements of the novel PICN CAD/CAM materials are reduced brittleness, rigidity and hardness coupled with improved flexibility, fracture toughness and better machinability compared to ceramics. Further improvements would be to maintain the wear “kindness” toward opposing teeth such as occurs with composites along with enamellike material attrition. The long-term aim is to imitate the mechanical behavior of a natural tooth. A literature summary of the physical properties of human dentin and enamel is listed in Table 1 .
Elastic modulus [GPa] | Hardness [GPa] | Fracture toughness [MPa √m] | Density [g/cm 2 ] | |
---|---|---|---|---|
Dentin | 16–20.3 | 0.6–0.92 | 2.2–3.1 | 1.96–2.4 |
Enamel | 48–105.5 | 3–5.3 | 0.6–1.5 | 3.02 |
The main aim within the scope of this paper was the comparison of elastic modulus and hardness of PICNs, dense ceramic and polymer, employing three-point-bending and Vickers indentation. Also, the flexural strength of the mentioned materials above was compared. Additionally, the microstructure deformation and cracking behavior around indented areas of PICNs were observed by SEM.
2
Materials and methods
2.1
Manufacturing of PICN
In the first step a porous pre-sintered feldspar ceramic with adjustable densities was produced. In the second step the porous ceramic network structure was filled with resin. The ceramic powder was initially compressed into blocks then sintered to a porous network. Different porosities of the ceramic could be achieved by manipulating the initial ceramic particle size and utilizing different firing temperatures. The ceramic network had to be conditioned by a coupling agent prior to resin infiltration. Thus, the polymer network is chemically cross linked to the ceramic network to form an interpenetrating network system. The chemically conditioned porous inorganic network was infiltrated with a cross-linking polymer (resin mixture and initiator namely, Urethandimethacrylate, Triethylenglycoldimethacrylate, Dibenzoylperoxide, Evonik Industries AG, Essen, Germany) by capillary action. Subsequently heat induced polymerization, to form a polymer network, led to the polymer-infiltrated-ceramic-network material.
2.2
Materials
Ten materials were tested in this study. They included the pure components of PICN that is a polymer (mixture of two methacrylates), a densely sintered feldspar ceramic, four feldspar ceramic networks with varying porosities ( Table 2 ) and additionally four PICNs based on the porous ceramics mentioned before. The density in g/cm 2 as well as in percent and the pore volume in cm 2 /g of the porous and dense ceramics, are listed in Table 2 . The density of the dense feldspar ceramic is 2.44 g/cm 2 measured by Archimedes’ principle, and considered as 100% dense. The densities of the porous ceramics were determined based on the dense ceramic.
Material | Density [g/cm 2 ] | Density [%] | Pore volume [cm 2 /g] |
---|---|---|---|
Porous feldspar ceramic | 1.45 | 59 | 0.266 |
Porous feldspar ceramic | 1.54 | 63 | 0.248 |
Porous feldspar ceramic | 1.66 | 68 | 0.208 |
Porous feldspar ceramic | 1.76 | 72 | 0.145 |
Dense feldspar ceramic | 2.44 | 100 | 0 |
2.3
Methods
2.3.1
Flexural strength, elastic modulus and strain at failure
For the flexural strength and static elastic modulus measurement, bending bars ( n = 10) of each material (20 × 4 × 1.2 mm 3 ) were cut out of blocks with a diamond saw. Subsequently bending bars of the materials except porous ceramics were ground and polished with a 3 μm diamond suspension (Struers, Willich, Germany). Porous materials were prepared on silicon carbide paper (grit size 340 and 1200). All bending bar edges were chamfered. The cutting and preparation of bending bars of porous ceramic with initial density of 59% was not possible (insufficient sintering necks and too fragile). The bars were loaded until fracture in a universal testing machine (Zwick, Model Z010, Ulm, Germany) in three-point flexure with a crosshead speed of 0.5 mm/min. The fracture stress, σ f , was calculated by the formula (according ISO 6872 ):
σ f = 3 F l 2 w h 2
where F is the fracture load, l the roller span (here 15 mm), w the width and h the height of the bar. In Tables 3 and 4 the flexural strength mean values and standard deviations were listed.
The static elastic modulus was calculated from the three-point bending results, by the formula :
E f = F l 3 4 w h 3 d
where d is the deflexion corresponding to load F .
For comparison with experimental data and prediction of the elastic modulus of PICNs, the Halpin–Tsai analytical equation was employed :
E PICN = E P ⋅ 1 + ξ η V C 1 − ξ η V C
η = ( E C / E P ) − 1 ( E C / E P ) + ξ
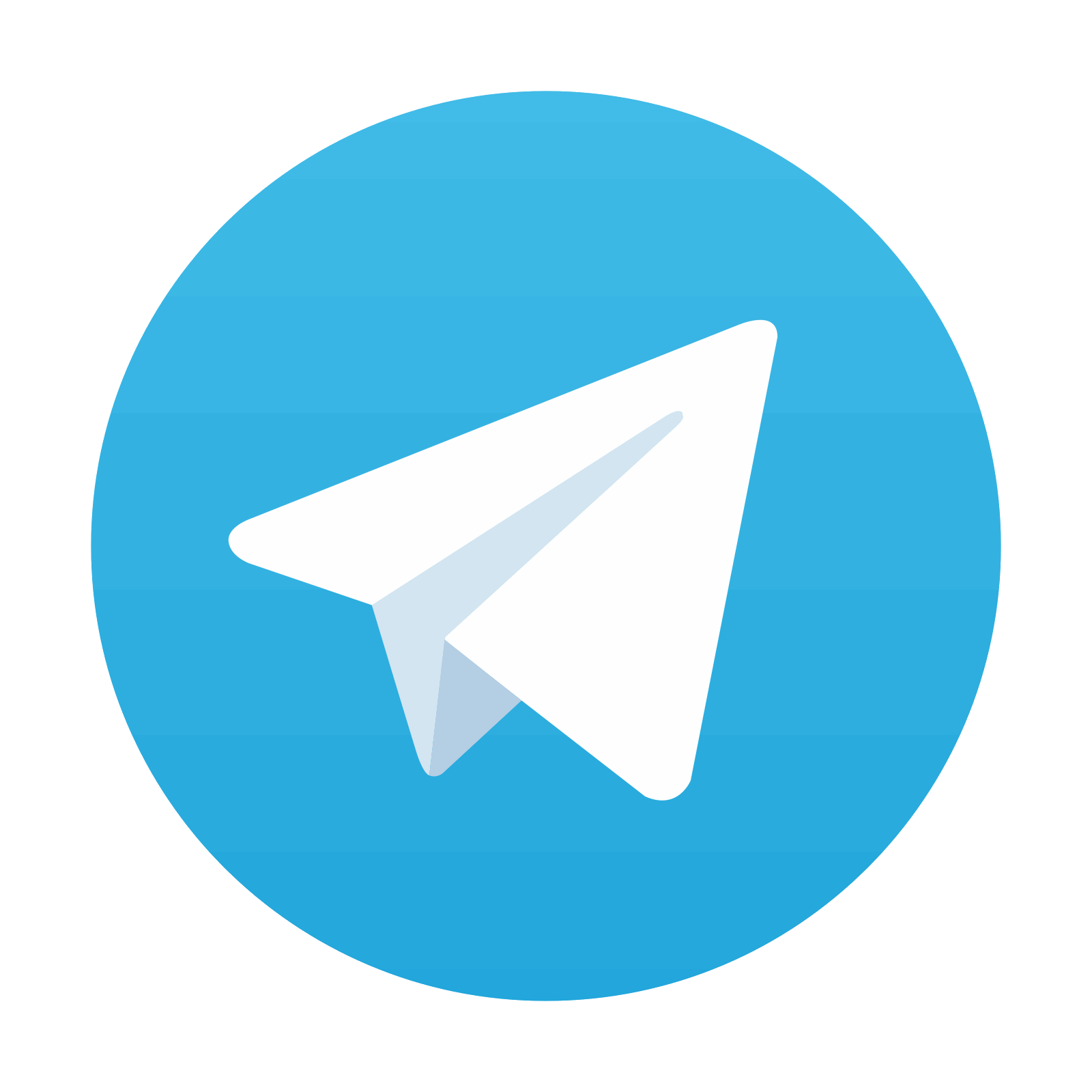
Stay updated, free dental videos. Join our Telegram channel

VIDEdental - Online dental courses
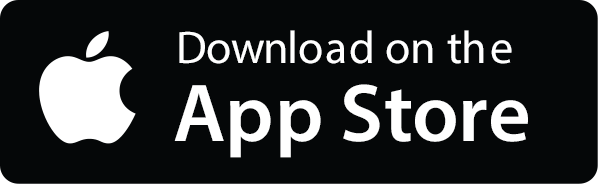
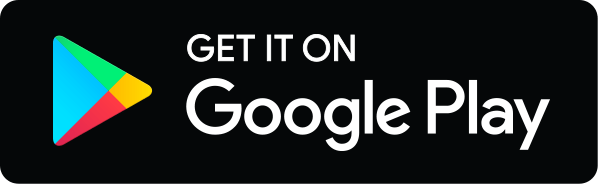