Abstract
Objectives
Maxillofacial silicone elastomers are used to replace facial parts lost through disease or trauma. However, these materials do not possess ideal properties. The purpose of this study was to investigate the mechanical properties of recently and previously introduced maxillofacial silicone elastomers and their bond strength to acrylic resin substrate.
Materials and methods
Specimens of three maxillofacial silicone elastomers (TechSil S25, Cosmesil M511, and Cosmesil Z004) were prepared according to manufacturers’ instructions. Tear and tensile strengths, elongation percentage, modulus of elasticity and hardness were evaluated for each material. Shear bond strengths of these silicone elastomers to primed acrylic resin surfaces were evaluated. Data was analyzed with 1-way ANOVA, Bonferroni, and Dunnett’s T3 post hoc tests ( P < 0.05).
Results
The tensile strength of S25 was higher than M511 and Z004 ( P < 0.05). Also S25 had higher elongation percentage in comparison to the other materials ( P < 0.05). Z004 and S25 were harder ( P < 0.05) than M511. All materials had the same tear strength and modulus of elasticity ( P > 0.05). Z004 had higher shear bond strength than S25 ( P < 0.05). There was a positive linear correlation between shear bond strength, and tear strength ( r = 0.974) and shore A hardness ( r = 0.717).
Conclusions
All silicone elastomers tested showed desirable properties. TechSil S25 had more favorable combination of high tensile strength and elongation at break, comparable tear strength, and hardness within the favorable range. However, Cosmesil Z004 was more resistant to shear debonding than other silicone elastomers.
Significance
TechSil S25 silicone elastomer has promising properties that favor its use in fabricating maxillofacial prostheses.
1
Introduction
Facial disfigurements, which result from congenital abnormalities, cancer resection, or trauma, are restored aesthetically and functionally into natural-appearing reproductions of the missing parts, using silicone elastomers . Silicone elastomers have been widely used to fabricate facial prostheses because of their chemical inertness, strength, durability, ease of manipulation, and biocompatibility . However, silicone elastomers are far from ideal. Their polymer chains, silica fillers, and the interactions between these two components affect the overall silicone strength and bonding .
High tear resistance, high tensile strength, and adequate hardness, along with sufficient bonding to the underlying retentive substrate are essential characteristics of maxillofacial silicone elastomers . Tear resistance of silicone elastomers is clinically important especially at the thin margins surrounding facial prostheses, which blends the prosthesis with the surrounding facial tissue. Margins are usually glued with medical adhesive, and are highly susceptible to tear especially when the facial prosthesis is removed at nighttime or for cleaning . The tensile strength of the silicone elastomer indicates the overall strength and how far the material stretches before it breaks . Skin changes in elasticity among individuals and during aging, whereas more wrinkling and pigment lesions are evident . Hardness of the maxillofacial silicone elastomer determines its flexibility, and it is desirable to have a material with similar softness to facial tissues surrounding the defect site. However, there is a lack of desirable values of the physiomechanical properties of maxillofacial silicone elastomers, as they were only mentioned two times in the literature . On the other hand, bonding of silicone elastomers to retentive substrates whether made from acrylic, polyurethane , titanium , or FRC , is an equally important criterion of functional facial prosthesis.
Various studies on maxillofacial silicone elastomers have investigated their mechanical properties , and bonding characteristics . They used a wide variety of testing methods and standards, making direct comparisons between them impossible. For example, the tear resistance of maxillofacial materials is a complex phenomenon and dependent on the shape of the test specimens (i.e. trouser, angle, or crescent) and also on the cross-head speed of mechanical testing. The bond integrity of silicone elastomers was investigated using peel , shear , and tensile bond tests . All these tests have been subjected to constructive critique. Peel test, despite its simulation to clinical setting, its results are influenced by compliance and thickness of materials; along with bond failures being predominantly cohesive within peeled materials . Shear bond test is claimed to concentrate stresses at the edges, and it is affected by the deformation rate chosen , materials tested and their testing arrangements . Tensile test is a sensitive method, and represents the tensile strength of the materials rather than their bonding to the substrate .
Bond primers were also utilized to increase bond strength , and indicated that primers and silicones should be compatible in order to achieve strong bond strength .
Nevertheless, all studies reached to the conclusion that further improvements are required in the mechanical and physical properties and bonding ability of poly(dimethylsiloxane) maxillofacial silicone elastomers.
Cosmesil (Principality Medical Ltd, Newport, UK), and A-2186 (Factor II, Lakeside, Arizona) are popular silicone elastomers which have been widely reported as better serviceable materials . Furthermore, they provided a baseline with which newer silicone elastomers can be compared . New silicone elastomers are used today but their properties and bonding integrity to denture base resins remain elusive. Therefore the aim of this study was to investigate mechanical properties of recently and previously introduced maxillofacial silicone elastomers and their bond strength to acrylic substrate. Our null hypothesis is that all silicone elastomers tested are not different from each other in all tested properties and bonding abilities.
2
Materials and methods
Materials used in this study are presented in Table 1 . For mechanical properties testing, the silicone elastomers were processed in dental stone molds using a dry heat oven according to manufacturer instructions. Hard wax patterns (Associated dental products Ltd, Swinton, UK) were invested in type 1 dental stone (Dentsply, Surrey, UK) in conventional denture flasks to construct the molds. After the stone had set, the flasks were opened and the patterns removed, and molds were ready to construct the specimens. The silicone elastomers were mixed manually for 5 min followed by mechanical mixing under vacuum for 5 min (Multi Vac 4, Degussa, Frankfurt, Germany) . The molds were isolated with a thin layer of sodium alginate (Hillier dental, Kent, UK), and then the silicone was poured into them, with the aid of vibration. The molds were cured in a dry oven ( Table 1 ). After curing, the specimens were removed from the molds, excess silicone was trimmed, and specimens were incubated at 23 ± 1 °C for 24 h, and then the mechanical properties testing was performed. For tensile and tear tests eight specimens for each material were fabricated, and five specimens for hardness test. Specimens were tested after 24 h of processing, and at 23 ± 1 °C room temperature and 50 ± 5% relative humidity.
Materials | Batch number | Rubber:catalyst ratio (g) | Processing parameters | Manufacturer |
---|---|---|---|---|
TechSil (S25) | 08/02 | 9:1 | Heat cured for 2 h at 100 °C | Technovent Ltd, Leeds, UK |
Cosmesil series materials (M511) | 08/01 | 10:1 | Heat cured for 1 h at 100 °C | Principality Medical Ltd, Newport, UK |
Cosmesil series materials (Z004) | 08/02 | 1:1 | Heat cured for 1 h at 100 °C | Principality Medical Ltd, Newport, UK |
Type 2 dumbbell-shaped tensile test specimens were fabricated and tested according to ISO specification number 37 ( Fig. 1 ) . Tensile grips were installed on a universal testing machine (Zwick/Roell Z020, Leominster, UK), with a separation of 20 mm between them. Tensile tests were performed at 500 mm/min cross-head speed. Prior to testing, the thickness of each specimen was measured using a digital caliper (Mitutoyo, Tokyo, Japan.) at the center and at each end of the test specimen, and the median thickness was used for cross-sectional area calculations. Tensile strength (TS) and elongation percentage (EP) were calculated automatically by the software using Eqs. (1) and (2) , respectively.
T s = F b W t
EP = L b − L o L o × 100 %
where F b is force recorded at break (N); W is width of the narrow portion of the specimen (mm); t is thickness of the test length (mm); L o is initial test length (mm); and L b is test length at break (mm). The tensile modulus was obtained from the plotted stress–strain curve when specimen is subjected to a 100% strain , using SigmaPlot software (release 11, SPSS Inc., Chicago, IL).
Trouser-shaped tear test specimens were fabricated (100 mm × 15 mm × 2 mm) and tested according to the ISO specification number 34 ( Fig. 2 ) . A cut in the test specimen was made at the center of the width of the test piece (40 mm long). The tensile grips were used in performing the tear tests. Test specimens trouser legs were inserted symmetrically and in axial alignment with the direction of the pull in each grip. The depth of insertion was 30 mm, ensuring that the specimen is adequately gripped. Specimens were tested at a strain rate of 100 mm/min with the Zwick/Roell Z020 machine (Leominster, UK). Tear strength ( T s ), expressed in kilo Newton per meter of thickness (kN/m) was calculated according to Eq. (3) .
T s = F d
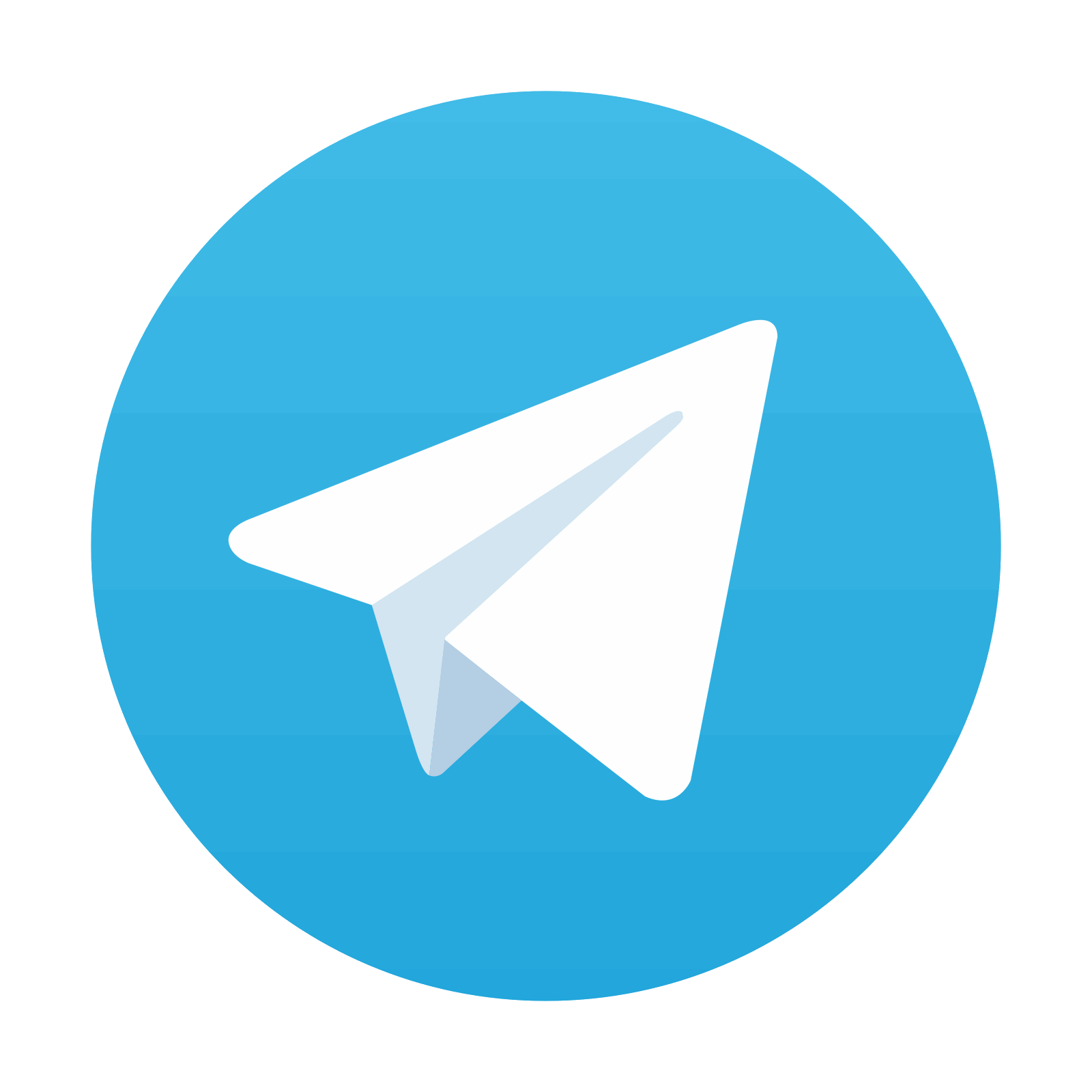
Stay updated, free dental videos. Join our Telegram channel

VIDEdental - Online dental courses
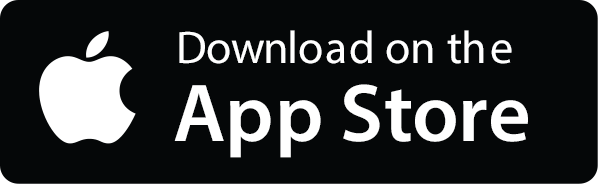
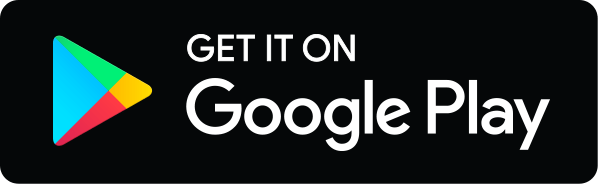