Abstract
Objective
The aim of this study was to evaluate the microstructure and mechanical behavior of polymer-infiltrated zirconia ceramics as a function of pre-sintering temperature (1000–1150 °C).
Methods
Polymer-infiltrated zirconia ceramics were prepared by combining the porous zirconia networks and polymer through infiltration and polymerization. XRD was employed to determine phase structure. The microstructure and fracture mechanism were observed by SEM. Flexural strength and fracture toughness were measured by three-point bending method and single-edge-notched beam method, respectively. A nanoindentation system was employed to determine elastic modulus and hardness.
Results
Different porosities and polymer contents can be obtained by tuning the pre-sintered temperature of zirconia ceramic precursors. Zirconia network porosity varies from 46.3% to 34.7% and the relevant polymer content ranges from 18.4 wt.% to 12.3 wt.% when the pre-sintered temperature is set from 1000 °C to 1150 °C. The flexural strength, fracture toughness, hardness, and elastic modulus values of the specimen pre-sintered at 1150 °C are 240.9 MPa, 3.69 MPa m 1/2 , 3.1 GPa, and 58.8 GPa, respectively.
Conclusion
The pre-sintering temperature has a significant effect on the microstructure and mechanical properties of polymer-infiltrated zirconia ceramics and the optimal pre-sintering temperature is 1150 °C.
Clinical significance
Specimen pre-sintered at 1150 °C shows tooth-like mechanical properties, suggesting a promising restorative material in dental clinic. Moreover, the synthesis process is simple and can be easily performed in a prosthesis laboratory.
1
Introduction
Ceramic and composite materials, engineered with CAD/CAM technique, have been widely applied in esthetic dentistry in recent years . There are mainly two types of dental ceramics: glass-ceramics and polycrystalline ceramics . Glass-ceramics, like lithium disilicate, enjoy good biocompatible and esthetic properties, but suffer from unsatisfying brittleness . The tougher and stronger polycrystalline ceramics, such as alumina-based ceramics , and zirconia-based ceramics , have their own shortcomings, for instance, opaqueness, unusually high hardness and excessive elastic modulus . For esthetic considerations, the polycrystalline ceramics are usually covered with a layer of veneering porcelain in clinical practices . However, the restoration with porcelain veneering is characterized by high failure rate, which is typical for all-ceramic restorations due to too fast firing and cooling . Furthermore, the hardness of polycrystalline ceramics is two to four times higher than that of enamel. On the other hand, the Young’s modulus of human dentine lies between 20 and 25 GPa , which is far lower than 200 GPa for zirconia and 340–390 GPa for zirconia toughened alumina . A favorite hardness and elastic modulus should mimic those of enamel and dentin as much as possible . Due to a good combination of mechanical behaviors and esthetic effects, resin composites have been extensively applied to dental restoration, and many efforts have been made to tailor their performances . However, marginal failures have been caused by the degradation of the dentin side of the composite-dentin interface by matrix metalloproteinase (MMPs) .
Driven by the demands to design restorations with real tooth-like function, a novel composite, polymer-infiltrated-ceramic-network material (PICN), has emerged in recent years inspired by the glass-infiltrated ceramic structure . Different from conventional filler-reinforced resin composites, the polymer-infiltrated-ceramic-network material is composed primarily of a porous ceramic structure with an interpenetrating resin phase, which overcomes the shrinkage problem . But, commercial products like Vita Enamic ® have mainly focused on the polymer-infiltrated feldspar ceramics for esthetic effects, and it suffers from a relatively low strength and toughness, for example, 103.36–159.88 MPa for flexural strength and 0.44–1.72 MPa m 1/2 for fracture toughness .
As discussed above, mechanical properties resembling natural teeth, including flexural strength, fracture toughness, hardness, elastic modulus and brittleness, can hardly be achieved with a single type of material. Zirconia-based ceramic plays a vital role in the all-ceramic system in clinic due to its excellent mechanical behaviors and outstanding esthetic property. Its application in the polymer-infiltrated-ceramic-network structure coupled with Bis-GMA (Bisphenol A glycerolate dimethacrylate) and TEGDMA (Tri(ethylenglycol) dimethacrylate) is expected to exhibit biomimetic mechanical performances, including an increase in fracture toughness and flexural strength as well as a reduction in hardness and elastic modulus. The aim of this study is to tailor a material to simulate the mechanical properties of natural teeth. The influence of the pre-sintering temperature, porosity, polymer content and crack bridging on the microstructure and mechanical behaviors of polymer-infiltrated zirconia ceramics is addressed.
2
Experimental procedure
2.1
Materials
3Y-TZP (Tosoh Co., Tokyo, Japan) with an average particle size of 90 nm was used to prepare porous zirconia ceramic matrix. Bis-GMA (Bisphenol A glycidyl dimethacrylate), TEGDMA (Tri(ethylenglycol) dimethacrylate), and BPO (benzoyl peroxide) were purchased from Aldrich Chemical Co., China.
2.2
Synthesis of polymer-infiltrated zirconia ceramics
The porous zirconia ceramic matrixes were prepared by a solid-state ceramic process. The starting powder, 3Y-TZP, was poured into a stainless steel mold and pressed at 4 MPa, followed by a cold isostatic pressing at 200 MPa. In order to obtain different porosities, the initial blocks were partially sintered in air at the following temperatures, 1000 °C, 1050 °C, 1100 °C and 1150 °C. The pre-sintered zirconia networks were conditioned with silicone coupling agent prior to polymer infiltration as an attempt to stimulate the formation of chemical bonds between the two materials. γ-MPS (Aldrich Chemical Co., China) dissolved in ethanol was employed as the silicon coupling agent. TEGDMA with a mass ratio of 49.5 wt.% and BPO with a mass ratio of 1 wt.% were added to Bis-GMA to be uniformly blended for infiltration. Zirconia networks were immersed in the liquid polymer, resulting in two interpenetrating phases by capillary action in −0.1 MPa vacuum. And then, the polymer-infiltrated zirconia ceramics were obtained after polymerization under the atmospheric pressure by heat treatment at 70 °C for 10 h.
2.3
Characterization
2.3.1
Phase structure and microstructural analysis
X-ray diffraction spectroscopy (Rigaku D/MAX-2550 V, Japan) was employed to analyze the phase composition. The morphology of surface sections before and after infiltration was examined by scanning election microscopy (Hitachi, S-2500N, Japan). The semi-quantitative analysis of elements was conducted by energy dispersive spectrometer (Hitachi, S-2500N, Japan).
The relative density was measured by the Archimedes method and estimated by using the theoretical density of 6.05 g cm −3 for ZrO 2 . Thus, the total porosities (П) were calculated by the equation,
Where ρ 0 is the relative density of the as-prepared ceramic. In order to analyze the resin content, TGA of specimens was performed at temperatures up to 650 °C at 20 °C/min of heating rate by using Mettler Toledo STAR system.
The number of specimens per group is 6 for these tests, respectively.
2.3.2
Mechanical behaviors analysis
A universal test instrument (Shimadzu, EZ-100, Japan) was employed to measure the flexural strength and the fracture toughness of specimens by three-point bending method with a loading rate of 0.5 mm/min and single-edge-notched beam method with a loading rate of 0.05 mm/min, respectively. For flexural strength test, polished bending bars of 2 × 3 × 16 mm 3 ( n = 21) of each specimen were cut out from blocks according to ISO 4049. For fracture toughness test, bending bars ( n = 21) per specimen were cut into 2 × 4 × 16 mm 3 with diamond blade and the notch depth was approximately 2 mm. The flexural strength was calculated by the equation
Where P 0 is the load, l is the span of the sample, h is the height of the bar, and b is the width of the bar. The fracture toughness was calculated using formula:
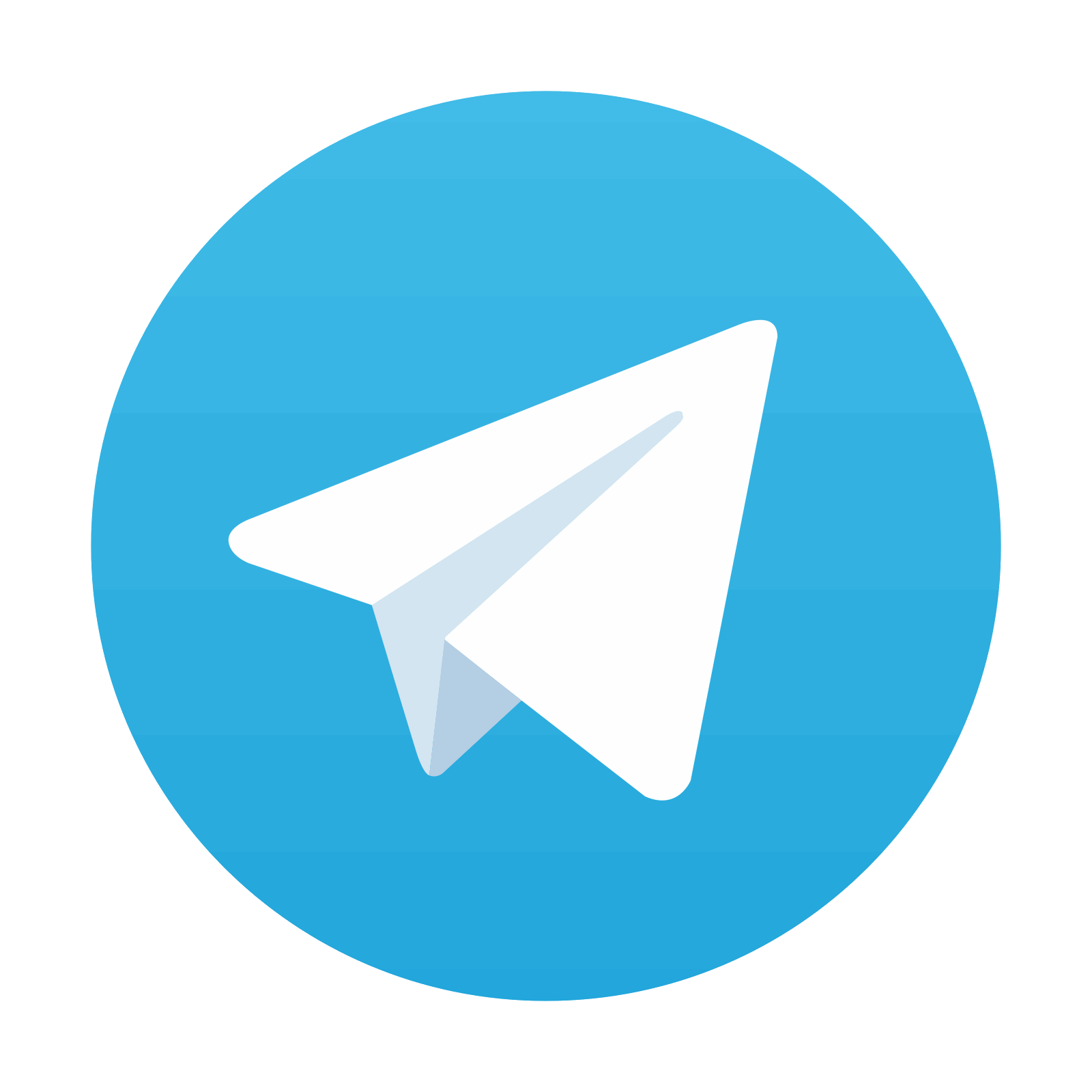
Stay updated, free dental videos. Join our Telegram channel

VIDEdental - Online dental courses
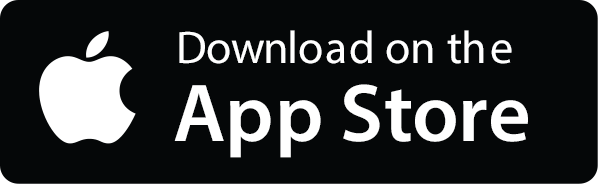
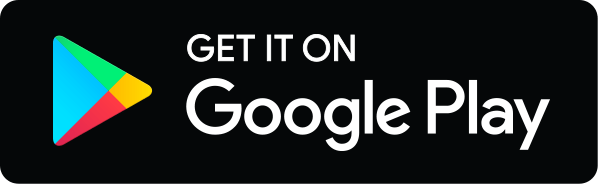