For successful outcomes in bone grafting, it is important to have a clear and detailed understanding of the fundamentals and basics in regenerative science. This article summarize the grafting materials and growth factors that are now in use to provide an improved understanding of the properties of each material and indications for subsequent use. The article gives an overview of the fundamentals of bone healing, including the physiology of regeneration. It is hoped that clinicians can make improved decisions that are based in literature when considering treatment options for restoring patients’ functional dentition.
Key points
- •
This article provides a summary of the basic knowledge and fundamental principals of bone grafting in the setting of Oral and Maxillofacial Surgery.
- •
Autogenous bone graft is currently the gold standard in regards to grafting success.
- •
Available grafting materials include autograft, allograft, xenograft and alloplast.
- •
Bone morphogenic protein is predictable method for inducing bone formation.
Introduction
Successful outcomes in the use of bone grafting and reconstructive outcomes heavily depend on understanding the fundamentals and material properties. The purpose of grafting is to achieve regeneration of hard tissues. The regenerated bone must have the capacity to provide the same physiologic support as the original dentition. Such goals can be achieved through regenerating well-vascularized bone that will undergo normal remodeling and healing. Prosthetically, a variety of restorative options, including fixed, hybrid, and removable restorative options, have become available. These options restore functionality and quality of life to patients who may have both esthetic and functional disabilities. Regenerated bone must have histologically and physiologically identical characteristics to native bone. Implant integration and function depend on the composite characteristics and structural stability of native bone to function. This article provides an overview of the currently available bone regenerative materials, their advantages, and their uses in the current literature.
Biology of bone
Bone is a composite structure composed of a macrostructure of type I collagen with calcified components. Bone is a dynamic structure undergoing constant resorption and deposition by osteoclasts and osteoblasts. To maintain balance, the body orchestrates cell activity by secreting parathyroid hormone (PTH), vitamin D, and calcitonin. This dynamic system plays an important role in growth, adaptation to biomechanical stresses, and repair of macrofractures and microfractures.
Bone deposition, resorption, and maintenance are achieved through the actions of osteoblasts, osteoclasts, and osteocytes. Osteoblasts are derived from local differentiated osteoprogenitor cells in reaction to stimuli. Such osteoprogenitor cells are present in the periosteal and endosteal layers of the bone. These critical parenchymal tissues serve as a vital reservoir for undifferentiated stem cells in addition to serving as a blood supply to the native tissue. Minimizing the reflection of periosteum during dissection preserve vascularity to the local tissues, which leads to improved outcomes of bone regeneration. Osteoclasts are resorptive cells that, through the actions of alkaline phosphatase, resorb bone and release calcium. Through the coordinated actions of PTH, calcitonin, and vitamin D, bone turnover and calcium levels are maintained. Diseases that affect this balance can be detrimental to the body. Diseases such as Paget, rheumatoid arthritis, and osteopenia can compromise the integrity of the bony framework. Conditions such as release of parathyroid hormone–related proteins in squamous cell carcinoma and renal osteodystrophy can result in osteolytic lesions defects that make bone susceptible to fracture.
Bone is composed of 2 major components: cortical and trabecular bone. Cortical bone is a dense housing that forms the outer layers that encase the trabecular bone marrow. Harvesting and grafting each type affects the quality of the regenerated bone. Cortical bone is a dense structure made of haversian canal systems. Examination of cortical bone reveals bone resembling tubes with a central core containing osteocytes. Cortical bone is composed of multiple units of tubules, referred to as haversian canals. Trabecular bone is formed by an aggregate of bone loosely arranged in a meshlike fashion. Within this lattice, erythron and leukopoiesis takes place.
During bone formation, mesenchymal cells are stimulated by growth factors during development and differentiate into osteoblasts. Osteoblasts deposit bone in their immediate surrounding, encasing themselves within a mineralized matrix termed lacunae. As the bone grows, these osteoblasts mature into osteocytes. Groups of lacunae form into conical structures previously referred to as haversian canals. The individual osteocytes communicate with one another through the canals, which allow resorption and deposition in response to the needs of the body. Trabecular bone forms within the core of the developing cortical bone. A thin, fibrous tissue subsequently forms around the maturing bone. Periosteum is the layer that envelopes cortical bone. In comparison, endosteum envelopes trabecular bone. The tissue surrounding these bony structures provides vascular supply and nutrition ( Table 1 ).
Type of Bone | Histologic Features |
---|---|
Organized matrix | 40% of the dry weight of bone. Composed of 90% type 1 collagen, noncollagenous proteins, ground substance, water, proteoglycans, cytokines, and growth factors |
Cells | Osteoprogenitor cells: osteoblasts osteocytes osteoclasts |
Vascular and nutrient | Bone receives 5%–10% of cardiac output via arterial supply through the periosteum and endosteum. |
Distribution | Microcirculation, lymphatics, and venous return |
Neurologic | Bone is supplied by autonomic and neurosensory networks |
Marrow | Serves hematopoietic and osteogenic functions |
Periosteum | A source of osteoprogenitor cells, neurovascular distribution, and blood supply |
Endosteum | A source of osteoprogenitor cells |
Communication systems | A network including haversian and Volkmann canaliculi lacunae and extracellular fluid |
Bone is classified into several categories depending on the thickness and volume of cortical and cancellous bone. The composition changes depending on the load and demands on the bone. Branemark described and classified bone based on the composition of cortical and trabecular bone. Type 1 bone is composed mainly of cortical bone with minimal amounts of trabecular bone. In contrast, type 4 bone is composed mainly of trabecular bone and little cortical bone. Types 2 and 3 bridge the gap between types 1 and 4, with more trabeculae present in type 3 bone. The mandible is typically characterized by a composition of type 1 and 2 bone. Type 3 bone primarily makes up the maxillary alveolus, with sections of type 4 bone posteriorly toward the tuberosity.
Implant placement and primary stability are affected by the type of bone in which it is placed. Implant placed in type 2 bone is likely to have good primary stability with good bone/implant interface, and such bone is typically found in the mandible. Type 3 bone may need an implant with a more aggressive thread design, and such bone is typically found in the maxilla. Both types 1 and 4 bone have their own respective disadvantages and are not favorable for implant placement. Type 1 bone is dense with minimal trabecular bone present. Such bone has reduced vascularity and subsequently is more susceptible to necrosis and implant failure. Type 4 bone has minimal cortical bone present, and implants placed in such bone, although integrated, may not be able to support functional loads. The end result is implant failure and compromise in the foundation of the prosthesis.
Bone healing biology
The mechanism of bone healing closely follows that of other tissues. Bone must proceed through the 3 phases of healing: inflammation, proliferation, and remodeling. Inflammation leads to blood coagulation and hematoma formation. Damage to endothelial cells leads to platelet aggregation and degranulation. Critical growth factors are released to promote angiogenesis and formation of a hematoma. Growth factors include fibroblastic growth factor and vascular endothelial growth factors. In the proliferative phase, fibroblasts are recruited and migrate into the surgical site. Fibrin deposition and angiogenesis result in organized tissue development, referred to as granulation tissues. The fibrin and collagen network paves the way for osteoblast migration and collagen deposition within 48 hours.
Initial collagen meshwork in bone is composed of type I and III collagen. The fibers are laid in a haphazard manner and the process of ossification begins. Calcification begins in what are referred to as ossification or maturation centers. Immature osteoid forms around these foci of calcified tissues, slowly growing in a concentric manner. Additional osteoblasts are recruited and surround the osteoid, further expanding the bone until ossification centers come into contact with one another and merge. Large blocks of osteoid are referred to as a soft callus, forming 4 to 7 days following the initial insult. As the soft callus matures and bone consolidates in the remodeling phase, osteoid begins to reorganize into organized matrices of cortical and trabecular bone. The remodeling phase starts at 8 weeks and forms what is known as a hard callus. The remodeling phase is orchestrated by the actions of osteoblasts and osteoclasts, which are responsible for bone deposition and resorption respectively. Type III collagen is replaced by the composite structure made of type I collagen. At the time of remodeling, the bone is most brittle and susceptible to fracture. Sixteen weeks following the insult is when bone maturation and remodeling is completed. The bone is considered to have the structural integrity for load-bearing forces.
Effects of bone grafting on healing
The purpose of bone grafting and reconstruction is to regenerate tissues that are histologically identical to the native bone tissues. Aside from autogenous tissue, grafting materials are limited to those that are acellular. The remaining types of graft material are processed to maintain the macrostructure of bone or synthesized to mimic local scaffolding of bone to facilitate the movement and migration of local cells into the graft. This property of grafting materials is referred to as being osteoconductive. Techniques have been developed to preserve or add growth factors that add osteoinductive potential to the graft. The ability to facilitate and recruit cells into the graft material is referred to as being osteoinductive. Synthetic grafts such as calcium carbonate or even xenografts have been shown to have a slower rate of resorption and can act to resist compressive forces from wound contraction or masticator forces that may cause volume loss. Autografts have osteoinductive and osteoconductive properties. In addition, autografts transplant live osteoclasts, osteoblasts, and the pluripotent osteoprogenitor cells. Such tissues are considered to have osteogenic potential. The osteogenic potential of autografts has a significant positive impact on tissue healing and bone integration. Autografts are considered the gold standard of bone grafting materials.
Impacts on bone healing
Minimizing factors that can negatively affect bone healing leads to reduced risk of graft failure and increased volume of regenerated bone. Immobility and vascular perfusion are essential in bone healing. Micromotion in healing is considered an important contributing factor for nonunion. For example, fractured bone segments that are not immobilized result in micromotion and severance of the newly formed blood vessels. Compromises in the vascular supply lead to nonunion. In a similar fashion, grafted bone that receives pressure and is not immobilized has an increased incidence of failure, with greatly reduced bone volume regenerated. Pressure on grafted bone when placed under function can result in graft failure during the healing period due to compromised vascular supply.
Patients who have received radiation have drastically reduced angiogenic and cellular capacity for healing. Free radicals formed through radiation damage cell structure and cause sclerosis of vital vessels. The result is referred to as the 3 Hs: hypocellular, hypo-oxygenated, and hypovascular tissue. In such circumstances, patients must receive treatment to improve tissue oxygen perfusion in the form of hyperbaric oxygen. Hyperbaric oxygen treatment increases the oxygen tension of the tissues, subsequently stimulating angiogenesis with the goal of improving the chances of bone healing.
Systemic conditions that affect the vascular supply of tissues also have a negative effect on bone healing. Conditions such as diabetes and atherosclerosis reduce the flow of blood to the healing bone, subsequently reducing the nutritional supply to the healing tissues. In addition, modifiable risk factors such as smoking have devastating effects on the vascular supply to healing bone. The effects of nicotine on the vasculature last 5 hours (5 half-lives of 60 minutes) and compromise the blood flow to tissues. In addition, smokers have reduced oxygen carrying capacity through the formation of carboxyhemoglobin, which shifts the hemoglobin dissociation curve to the left. The result is a reduced proportion of hemoglobin molecules available for carrying oxygen. Patients that have stopped smoking have been found to have impaired macrophage and antimicrobial resistance for up to 6 months.
Basics of bone grafting
Clinical success in bone regeneration depends on good clinical practice and understanding the basics. Bone must have adequate blood supply and fixation. Several technical basics must be kept in mind when conducting patient care. Obtaining a good vascular supply is largely influenced by the clinician’s ability to design and handle soft tissue gently. Mucosal and attached gingiva must be handled with care, and crush injuries must be avoided when using the tissue forceps. When designing a flap, the base of the flap must be wider than the free margin of the flap. Folding or kinking flaps must be avoided to optimize blood flow and perfusion pressure to the flap. The clinician must conduct appropriate release of the soft tissue and periosteum. Without primary closure, blood supply is compromised and the bone is exposed to the oral flora, giving rise to potential infections. However, it is important to maintain tension-free closure to avoid ischemic necrosis in the soft tissue. It is prudent to pay attention to detail in flap design and not create incisions that will result in closure over root prominences (eg, over the canine root). Such flaps conveniently place incisions over points of tension.
When grafting, primary stability of the grafted material is critical. Micromotion in bone grafting or any pressure applied to the graft can compromise the vascular supply to the grafted material. Often, fibrous nonunion and subsequent graft failure result. Grafts can be fixated with the use of fixation screws or pins. A minimum of 2 screws must be placed to prevent rotational forces. Titanium mesh is a reliable medium to secure and house particulate grafts. The material is able to maintain its shape, resist functional forces of deformation, and minimize motion. Materials such as polytetrafluoroethylene (PTFE) have been developed to provide protection in cases where primary closure is difficult or not possible. These membranes have the capacity to remain exposed in the oral cavity and can be stabilized with the use of titanium screws or pins. Both PTFE and titanium mesh are nonresorbable membranes and must be removed before additional fixture placement. The PTFE membrane is typically removed 4 weeks following placement once the soft tissue callus has formed.
Bone grafting materials
Four basic types of bone grafting materials are available for use clinically to augment and reconstruct the maxillofacial skeleton ( Tables 2 and 3 ).
Bone Type | Description |
---|---|
Autograft (autogenous) | Transplant of viable cortical or cancellous bone from one location of the body to another within the same patient |
Xenograft | Cross-species transplant of tissue: the use of organic bovine bone or porcine collagen |
Alloplast | Implantation of synthetic material, such as apatite or tricalcium phosphate, bioactive glass, or polymers |
Bone Graft | Structural Strength | Osteoconduction | Osteoinduction | Osteogenesis |
---|---|---|---|---|
Autograft | — | — | — | — |
Cancellous | No | +++ | +++ | +++ |
Cortical | +++ | ++ | ++ | ++ |
Allograft | — | — | — | — |
Cancellous | — | — | — | — |
Frozen | No | ++ | + | No |
Freeze dried | No | ++ | + | No |
Cortical | — | — | — | — |
Frozen | +++ | + | No | No |
Freeze dried | + | + | No | No |
Autologous bone
Autologous bone refers to bone that is harvested form the individual’s own tissue. Examples of harvest sites used in the oral surgery setting include the anterior and posterior hip, calvarium, tibia, and mandibular ramus and symphysis. Use of autologous bone and its popularity in the routine oral surgical setting has decreased because of the predictability and availability of allogenic bone. However, autogenous bone is still maintained as the gold standard for bone grafting. This particular source of bone is the only graft material that boasts osteoconductive, osteoinductive, and osteogenic properties. In the contemporary setting, autogenous bone is typically reserved for large defects or situations where predictable results are hard to obtain; for example, in continuity defects and alveolar clefts. In patients with craniofacial dysplasia and oculoauriculovertebral spectrum disorders, rib grafts, with their respective cartilaginous components, have been used in the reconstruction of temporomandibular joints. Depending on the harvest site, different volumes and compositions of grafts can be harvested ( Table 4 ).
Noncompressed Corticocancellous | Cortical Block | |
---|---|---|
Tibia | 25–40 cm 3 | 1 <SPAN role=presentation tabIndex=0 id=MathJax-Element-1-Frame class=MathJax style="POSITION: relative" data-mathml='×’>×× × 2 cm |
Anterior Ilium | 50 cm 3 | 3 <SPAN role=presentation tabIndex=0 id=MathJax-Element-2-Frame class=MathJax style="POSITION: relative" data-mathml='×’>×× × 5 cm |
Posterior Ilium | 100–125 cm 3 | 5 <SPAN role=presentation tabIndex=0 id=MathJax-Element-3-Frame class=MathJax style="POSITION: relative" data-mathml='×’>×× × 5 cm |
Calvarium | Variable, minimal | Abundant |
As discussed previously, adequate vascular supply to the grafted bone is critical in the survival and integration of the graft. Defects ranging more than 6 to 8 cm are considered the limit for nonvascularized bone grafting. Reconstruction in defects large than 6 to 8 cm becomes challenging when using nonvascularized grafts because the nutritional and oxygen supply is inadequate. Nonvascularized grafts obtain their nutritional supply through a process known as imbibition. Subsequent inosculation of blood vessels to the graft provides new anastomosis and vascular network for the grafted tissues. The limitations of this process are reached when defects become bigger than 6 to 8 cm. When reconstructing large defects as a result of resections from ameloblastoma and squamous cell carcinoma or loss from traumatic injuries, vascularized grafts are indicated. A vascularized graft is needed to maintain nutrition and oxygen tension to the grafted tissue. Examples of vascularized bone grafts include free fibula, iliac crest, and scapular tip grafts.
In planning for reconstruction, it is important to consider the volume and composition of the graft that can be harvested. It is generally accepted that a 1-cm defect require 10 cm 3 of bone for reconstruction. Depending on the harvest site, large volumes of cortical or trabecular bone may be harvested. For example, the symphysis of the mandible yields primarily cortical bone, which is useful in reconstructing alveolar defects in the area of lateral incisors or in gaining structural support (vertical and horizontal). In contrast, harvesting graft from the ilium (anterior or posterior) yields a large volume of trabecular bone, which is useful in guided tissue regeneration and reconstruction of alveolar clefts. Cortical blocks are often used for their structural integrity in reconstruction. Periosteum often constricts during the healing process. Cortical grafts resist compression forces placed on the graft material during the healing process. Such resistance is important to maintain the volume of regenerated tissue and achieve the appropriate dimensions for fixture placement. Trabecular bone is often favorable for filling defects. The handling characteristics make marrow bone ideal for filling walled defects and contouring the graft sites.
Autogenous graft is advantageous for the key reason that it is the host’s own tissue. As mentioned previously, it has osteoinductive, osteoconductive, and osteogenic properties. Osteoconduction and osteoinduction refer to the graft’s ability to facilitate movement of cells into the graft and induce differentiation of host monocytes into osteoblasts, respectively. When harvested, the graft maintains the original macrostructure and microstructure of the tissue, which includes the cytokines, growth factors, and cells of the tissue. The transplant of vital host cells is referred to as osteogenic grafting. Components of the graft give autograft distinct advantages of efficient signaling and differentiation of osteoprogenitor cells present within the periosteum and endosteum within the host site and the graft. These properties facilitate efficient new osteoid formation and graft integration.
Because the tissue is harvested from the host, there is no risk of immune rejection and disease transmission. Autogenous grafts eliminate risks in transmission of diseases, including human immunodeficiency virus and hepatitis B and C. There are still reported cases of disease transmission despite the manufacturers’ efforts to appropriately screen, test, and process tissue in a disease-free condition.
The need for a secondary surgical site is the main disadvantage of autologous grafts. In addition, limited volume can be harvested and the process is associated with its own morbidity. For example, anterior and posterior iliac crest grafts have an associated risk of gait disturbance, which can result in difficulty ambulating. Complication rates have been reported to be 8.5% to 20%. Other types of complications include hematoma formation, gastric ileus, blood loss, neurosensory disturbances, hernia formation, pelvic fracture, and chronic pain. Harvest sites such as the symphysis can result in cosmetic defects, droopy chin, and sensory disturbances in the distribution of the mental nerve. The invasive nature and risk of morbidity restrict its access to those clinicians that are appropriately trained in the harvest of autogenous graft. In addition, its use must be carefully planned so the risks of harvest are justified by the benefits of the graft. However, with the use of trephine burs and bone scrapers, local harvesting techniques have made obtaining grafts easier. In summary, autogenous bone is a superior graft material with numerous advantages, such as improved healing time and predictable results compared with other types of available grafting material. However, its use must be carefully considered and the risks must be weighed against the benefits.
Cleft lip and palate is a good example of an indication for the necessary use of autogenous bone grafting. Carlini and colleagues report a case series of 16 patients with bilateral transincisive foraminal defects of the premaxilla. Following a period of orthodontic optimization and stabilization using a transpalatal appliance, the premaxillary segment was surgically repositioned and secured using an acrylic splint. The surgery involved invasive reflection and repositioning of soft and hard tissues ( Fig. 1 ). Iliac crest bone (cortical and trabecular) was harvested and the cortical plates were used to fixate the premaxilla to the posterior alveolar segments with titanium screws to achieve primary stability. Medullary bone was subsequently used to fill the remaining void and contour the bone. In such cases where incisions and osteotomies compromise the vascular supply to the tissues, autogenous graft provides the appropriate stability, tissue volume, and growth potential to obtain a predictable result. Precious and colleagues outlined repair of the cleft palate with the use of iliac crest bone graft. Repair and excellent results were achieved with the use of careful surgical planning, technique, and autogenous bone ( Fig. 2 ).
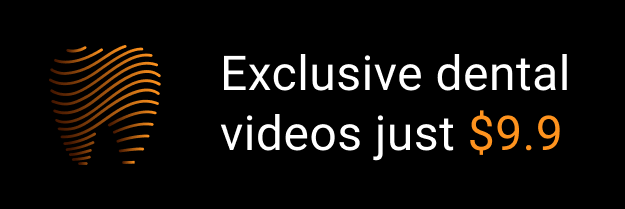