Abstract
Introduction
Gestational hyperthyroidism is an important cause of bone modifications in offspring, resulting from changes in endochondral growth. However, its effect on the craniodental development in offspring is unknown.
Objective
The objective of this study was to demonstrate the effect of maternal hyperthyroidism on the craniodental development of offspring.
Methods
Five pregnant Wistar rats with hyperthyroidism and five euthyroid rats were used in this study. At weaning, three pups per mother were selected from both groups. Blood was collected from the mothers on the day of birth of their offspring and from pups at weaning to measure plasma-free thyroxine levels.
Results
The influence of maternal hormones on offspring was confirmed by thyroid histomorphometry. The size of the rostro-caudal and latero-lateral axes of the skull, frontal bone width, and the thicknesses of the sutures and the bones were measured. In the molar teeth, the thicknesses of the dentin, pre-dentin, and odontoblast layers, as well as the thickness of the periodontal ligament, were evaluated. The concentration of free T4 was higher in hyperthyroid rats. The height of the thyroid follicular epithelium was lower in offspring of hyperthyroid mothers. Additionally, these offsprings showed a reduction in the width of the frontal bones and an increase in suture thickness. The molars in the hyperthyroid mothers showed a reduction in the thickness of the odontoblast and pre-dentin layers and an increase in the thickness of the periodontal ligament.
Conclusion
We conclude that maternal hyperthyroidism in rats causes significant changes in the cranialdental development of offspring.
1
Introducation
Hyperthyroidism is an important endocrine dysfunction characterized by elevated serum triiodothyronine (T3) and thyroxine (T4) levels. The disease affects 0.2%–1.3% of the general population [ ], and its main causes include hormonally active neoplasms, toxic multinodular goiters, and Graves’ disease [ ].
The proportion of people with hyperthyroidism during pregnancy is approximately 2% [ ]. In this population, the specific cause of hyperthyroidism is attributed to increased circulating human chorionic gonadotropin (hCG), which has a structure similar to that of thyroid-stimulating hormone (TSH) and can, therefore, bind to its receptors in follicular cells and induce the production of T3 and T4 [ , ]. As the fetal thyroid is not functionally mature until the 18th-20th week of gestation in humans and until the 17th day of gestation in rats, maternal thyroid dysfunction can affect the development of organs and tissues, such as teeth and bones, as the fetus is dependent on the placental transfer of maternal thyroid hormones [ , ].
Gestational hyperthyroidism is a significant cause of intrauterine growth restriction (IUGR), which involves the pathological reduction of fetal growth potential resulting from placental dysfunction [ , ]. Between 5 and 7% of pregnancies are affected by IUGR [ , ], and this alteration is present in approximately 20% of babies admitted to Neonatal Intensive Care Units [ ]. Placental dysfunction resulting from gestational hyperthyroidism is caused by reduced endovascular trophoblastic migration [ ], reduced vascularization [ ], and placental hormonal and inflammatory imbalances [ ].
Individuals born to pregnant women with hyperthyroidism exhibit remarkable bone changes, such as accelerated endochondral ossification and advanced bone age [ , ]. Long bones shorten [ ] owing to reduced chondrocyte proliferation, lower amounts of proteoglycans in the extracellular matrix, increased hypertrophic differentiation, and reduced vascular invasion [ , ].
Craniosynostosis, resulting from intramembranous ossification, has been reported in infants and children with juvenile hyperthyroidism [ ]. In mice, gestational hyperthyroidism causes premature fusion of sutures resulting from increased osteogenic differentiation, which results in skull growth arrest [ ]. In rats, these changes are not yet fully understood. A recent in vitro study conducted by our team demonstrated an increase in the osteogenic differentiation of osteoblasts extracted from the calvaria of neonatal rats exposed to gestational hyperthyroidism [ ]. However, the in vivo effects of this disease on intramembranous ossification in rats remain unknown.
Congenital or acquired thyroid dysfunction can alter tooth morphology, eruption speed, and predisposition to caries and periodontal diseases [ , ]. In children, there is an association between the development of dental enamel defects and hypothyroidism, whether acquired [ ] or gestational [ ]. The effects of maternal hyperthyroidism on the formation and development of offspring’s teeth and periodontium are unknown. Therefore, this is the first study to evaluate the effect of maternal hyperthyroidism on offspring’s teeth.
There are similarities between teeth and bone that extend beyond their mineralized composition. For example, dentin and cementum are variants of bone tissue that respond to several systemic factors acting on the bone. However, the question arises whether these components of the tooth would present changes similar to those described in the skeletons of growing animals subjected to maternal hyperthyroidism in the pre- and post-natal periods [ , ].
The hypothesis is that maternal hyperthyroidism accelerates the fusion of cranial sutures, resulting in a reduction in skull size. In addition, we believe that rats born to hyperthyroid mothers present reduced tooth growth. Therefore, the objective of the present study was to demonstrate, using histomorphometry, the effects of maternal hyperthyroidism on the craniodental development of rat offspring at weaning.
2
Material and methods
All the experiments were performed in accordance with the accepted standards of the Guide for the Care and Use of Laboratory Animals and the Brazilian Guidelines for the Care and Use of Animals for Scientific and Didactic Purposes (DBCA), from the National Council for the Control of Animal Experimentation (CONCEA). The present study was approved by the Animal Use Ethics Committee of the institution, under protocol no. 216/2019.
2.1
Copulation and induction of hyperthyroidism
Ten two-month-old female Wistar rats (>200 g) were housed in collective plastic boxes in a controlled environment (12 h of light and 12 h of dark, 18–22 °C). Commercial food and water were provided ad libitum for two weeks. After adaptation, the estrous cycle was monitored daily using vaginal cytology. Rats in estrus and proestrus were housed with adult males at a density of up to three females per male for 12 h. Copulation was confirmed by observing sperm in vaginal cytology, and this was considered day 0 of pregnancy. From day 0 of gestation, the rats were allocated to individual boxes and randomly placed in the control (n = 5) and hyperthyroid (n = 5) groups. Hyperthyroidism was induced by the daily administration of 50 μg of l -thyroxine (Sigma-Aldrich, St. Louis, MO, USA) diluted in 5 mL of water, through an orogastric tube [ , , ]. The treatment was administered from day 0 of pregnancy until weaning. At 21 days postpartum, that is, at weaning, the mothers and offspring were euthanized by an anesthetic overdose of ketamine (300 mg/kg) and xylazine (15 mg/kg) intraperitoneally.
2.2
Confirmation of hyperthyroidism and thyroid histomorphometry of offspring
Blood was collected from mothers on the day of giving birth and from offspring at weaning, for plasma-free T4 measurements. Collection was carried out in tubes containing heparin, with subsequent centrifugation to obtain plasma and storage at −20 °C. Free T4 concentration was measured using the ELISA technique (sensitivity: 0.4 ng/dL), with commercial kits (IMMULITE, Siemens Medical Solutions Diagnostics, Malvern, PA, USA).
In the offspring, the effect of excess maternal thyroid hormones was confirmed by morphometric analysis of the thyroid gland. Thyroid glands were dissected, fixed in 10% neutral formalin, buffered with monobasic and dibasic sodium phosphate, and processed using the routine paraffin embedding technique. Histological sections of 4 μm were stained using the hematoxylin-eosin technique for morphometric evaluation. The largest and smallest diameters of 30 follicles were measured randomly in each thyroid to obtain the average of these measurements. This measurement was performed on one histological section per animal using an eyepiece with a ruler attached to the microscope and a 40 × objective. The epithelial height was measured in 20 follicles. Four distinct and equidistant points were measured in each follicle to obtain the average values of the four measurements. This measurement was performed using a micrometric eyepiece with a ruler attached to the microscope and 100 × objective. The values obtained for the epithelial height and follicle diameter were transformed into micrometers using a micrometer blade [ ].
2.3
Skull histomorphometric analysis
After euthanasia, the skulls and jaws were disarticulated, dissected, and fixed in 10% buffered formalin for at least 24 h.
The rostro-caudal and latero-lateral axes of the skull were measured according to a protocol previously established by Silva et al. [ ]. The measurements were carried out with the aid of a digital caliper, with the rostro-caudal axis of the skull measured from the end of the snout to the external occipital crest, while the latero-lateral axis obtained as the distance between the two most mesial and superior insertion points of each ear. The width of the frontal bone was measured at the height of the zygomatic malar suture in the most constricted region of the frontal bone [ ] ( Fig. 1 A).

The skulls were decalcified with 10% EDTA for 14 days and sectioned before histological processing. The first fragment was obtained in a sagittal section parallel to the sagittal suture, in which the coronal, lambdoid, and occipital sutures and frontal, parietal, interparietal, and occipital bones were evaluated. The second fragment was obtained caudal to the coronal suture to evaluate the sagittal and squamous sutures and temporal bone ( Fig. 1 A). The fragments were then processed using the routine paraffin embedding technique to obtain 4 μm histological sections, which were stained with hematoxylin and eosin.
To evaluate the sutures, five measurements of the distance between the ossified edges were obtained and bone thickness was evaluated at five equidistant points. Analysis was performed under an optical microscope at 40 × magnification using an eyepiece with an attached ruler. The values obtained were transformed into micrometers using a micrometric blade.
2.4
Histomorphometric analysis of the molar tooth and periodontal ligament
The jaws were preserved in 10% buffered formalin, decalcified, and histologically processed in the same manner as the skulls. After complete decalcification, transverse sections were obtained below the ocular region, corresponding to the region of the molar teeth. The molar tooth was selected for its limited growth similar to that of the human tooth, making it possible to extrapolate the results to humans [ ].
The thicknesses of the dentin, pre-dentin, and odontoblast layers were measured at 25 points along each layer using a ruler and a 40 × objective. Finally, a correction factor obtained using the scale of the micrometric blade was applied to the averages, and the results were expressed in micrometers.
The morphology and thickness of the periodontal ligaments were evaluated. The thickness was measured at 50 points along the ligament using a ruler and a 40 × objective. Finally, a correction factor obtained using the scale of the micrometric blade was applied to the averages, and the results were expressed in micrometers.
2.5
Statistical analysis
Data were assessed for normality and homoscedasticity using Shapiro-Wilk and Brown-Forsythe tests. For variables that were within normal limits, the Student’s t-test was performed. For variables that did not meet the assumptions, even after logarithmic transformation, a non-parametric Mann-Whitney test was performed. GraphPad Prism 8.0.2 software was used, and the differences were considered significant at P < 0.05.
3
Results
3.1
Confirmation of hyperthyroidism
Thyroxine treatment significantly increased the plasma concentration of free T4 in treated rats compared with that in the control group. However, there was no significant difference between groups concerning the free T4 concentrations in the offspring ( Fig. 2 A).

Regardless of the group, the thyroids of newborns and rats at weaning showed follicles ranging from round to oval with varying diameters. In the control group, the follicles were covered predominantly by cuboidal epithelium and filled with dense and sometimes vacuolated colloids, whereas, in the offspring of rats treated with l -thyroxine, several follicles were covered predominantly by flattened epithelium. Although there was no significant difference in follicular diameter between the groups, the offspring of rats treated with l -thyroxine presented thyroids with a significant reduction in the height of the follicular epithelium compared to the control ( Fig. 2 B).
3.2
Skull histomorphometry
Maternal hyperthyroidism significantly reduced the width of the frontal bones in rats at 21 days of age ( Fig. 3 ). No significant differences were observed between the groups in skull length or latero-lateral axis width.

In offspring of the hyperthyroid mothers, a significant increase in the thickness of the coronal and occipital sutures was observed ( Fig. 4 A). The thicknesses of the lambdoid, sagittal, and squamous sutures did not differ significantly between the groups. All sutures exhibited similar microscopic morphologies ( Fig. 4 B–G). The sutures contained a large number of undifferentiated osteoprogenitor cells with scant cytoplasm and fusiform nuclei immersed in an abundant collagenous matrix ( Fig. 4 C). At the bone edge, there was a single layer of osteoblasts with abundant and weakly basophilic cytoplasm, polygonal in shape, and round-to-oval nuclei with condensed chromatin. The osteoid matrix formed a thick, slightly eosinophilic layer and housed osteocytes, with large nuclei retained in gaps of the usual width. In the mineralized bone matrix, osteocytes were abundant, with small round to fusiform nuclei and condensed chromatin. Regardless of the group, the coronal and squamous sutures were narrower than the others but had a larger bone surface, with fusiform osteoblasts and a smaller amount of osteoid matrix.

The frontal, parietal, interparietal, occipital, and temporal bones exhibited similar morphologies in all the groups. They had two outer leaflets of compact tissue covered by endosteum and periosteum, which housed the medullary cavity with a discrete amount of hematopoietic tissue. Occasionally, the inner and outer leaflets were fused, and the medullary cavity was non-existent. The thicknesses of the cranial bones did not differ significantly between the groups ( Fig. 5 ).

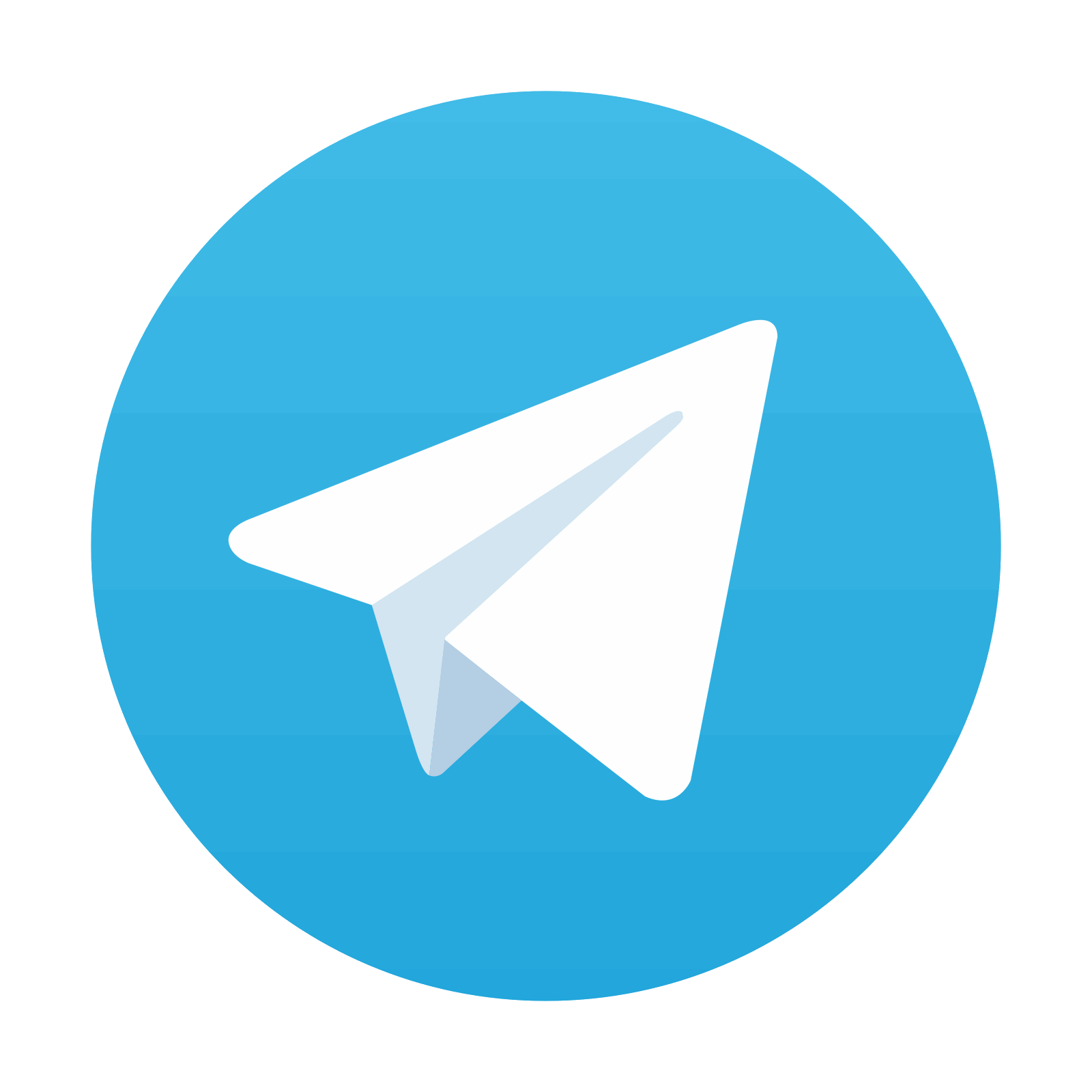
Stay updated, free dental videos. Join our Telegram channel

VIDEdental - Online dental courses
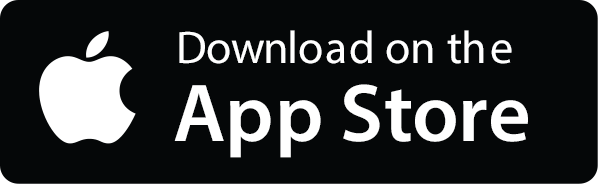
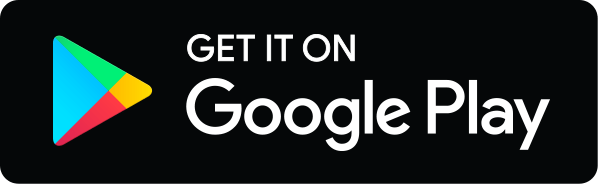
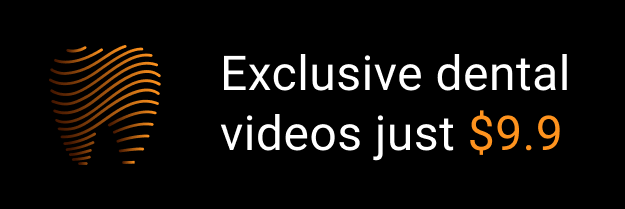