Introduction
The aim of this study was to evaluate the bone density of mandibular condyles in adolescents with varying skeletal patterns using cone-beam computed tomography. The null hypothesis was that there is no difference in the bone density of mandibular condyles in adolescents across various facial height ratios, ANB angle classifications, sexes, and age categories.
Methods
We divided 120 adolescent patients, 56 boys and 64 girls, into 3 groups according to 3 criteria: (1) age (early, 10 to <14 years; middle, 14 to <17 years; late, 17 to <20 years); (2) facial height ratio or Jarabak quotient (hyperdivergent: facial height ratio, <62%; normovergent: facial height ratio, 62% to ≤65%; and hypodivergent: facial height ratio, >65%); and (3) ANB angle classification (Class I, 1° to ≤4°; Class II, (>4°); and Class III, <1°). The total, cortical, and cancellous bone densities were measured and compared on the axial slice with the largest mediolateral diameter of the mandibular condyle using C-mode cone-beam computed tomography.
Results
Cortical bone density increased as age increased and showed statistically significant differences between the early and middle ( P = 0.041) and the early and late adolescent groups ( P = 0.031). Condylar bone density increased as facial height ratio decreased, and cancellous bone density showed statistically significant differences between the hyperdivergent and hypodivergent groups ( P = 0.038). The cortical, cancellous, and total bone densities increased as ANB angle increased and showed statistically significant differences between the Class II and Class III groups ( P = 0.022, P = 0.006, and P = 0.003, respectively).
Conclusions
The null hypothesis was rejected. Condylar bone density increased as facial height ratio decreased and ANB angle increased. These findings may be useful in predicting the vertical and horizontal skeletal growth patterns of growing adolescents.
Highlights
- •
We used CBCT to evaluate bone density of mandibular condyles in adolescents.
- •
Condylar bone density increased as facial height ratio decreased and ANB angle increased.
- •
ANB was a significant factor in predicting bone density.
- •
These findings may help predict vertical and horizontal skeletal growth patterns.
Skeletal patterns are analyzed and classified by vertical disproportions (hyperdivergent, normovergent, and hypodivergent) and by anteroposterior disproportions (skeletal Class I, Class II, and Class III) for orthodontic diagnosis. Understanding the various factors that influence mandibular growth and their subsequent effects on skeletal pattern can greatly enhance an orthodontist’s ability to provide an ideal treatment for a patient. This understanding is also critical for mandibular growth prediction and can provide essential information for treatment planning and posttreatment evaluation of occlusal stability.
Genetic and environmental factors have been shown to influence maxillofacial growth, including symphyseal morphology, masticatory function, skeletal and facial patterns, condylar growth, and condylar bone density.
The mandibular condyle’s role in the growth of the mandible has been a controversial topic in the literature. Previous views posited that the condyle was a critical component for mandibular growth and even was once believed to be a primary growth center that determined the growth rate and the overall size of the mandible. However, Enlow maintained that condylar growth is regulated and affected by extrinsic factors, and contributes little to mandibular growth. The point of intersection is that the mandibular condyle does not control the growth of the entire mandible, but it is essential for normal mandibular growth, particularly the enlargement of the ramus.
The bone density of the mandibular condyle is affected by the functional pressure generated by the occlusion and the mandibular movements that are, in turn, influenced by the properties of the masticatory muscles and age. For example, functional adaptation to the tension of the lateral pterygoid muscle may lead to a stronger and denser cortical layer of the condyle.
Skeletal pattern is thought to be closely related to occlusal force. Varrela reported that muscle activity during maximal clenching is correlated with mandibular prognathism, anterior inclination of the mandible, and a small gonial angle. Braun et al reported that maximum bite force increases with an increasing ratio of posterior facial height to anterior facial height. Taken together, patients with a retrognathic mandible and a dolichocephalic pattern tend to have lower levels of occlusal force.
Many methods have been used to predict mandibular growth and skeletal growth patterns. Björk discussed 3 methods for mandibular growth prediction: a longitudinal method (following development by annual cephalograms), a metric method (based on a metric description of facial morphology at 1 stage of development), and a structural method (by which it may be possible to predict from 1 cephalogram). Hägg and Taranger analyzed hand-wrist radiographs to evaluate mandibular growth and assess the pubertal growth spurt. Mito et al and Chen et al derived an equation to predict mandibular growth potential. Although these methods have been shown to be successful at predicting mandibular length, it is still difficult to accurately predict mandibular growth. Although previous studies have included jaw bone density, there is little understanding of mandibular condyle bone density in adolescents and how this information could be used to predict mandibular growth.
Therefore, the aims of this study were to measure and compare the bone density of mandibular condyles in adolescent patients with varying skeletal patterns using cone-beam computed tomography (CBCT). The null hypothesis was that there is no difference in the bone density of the mandibular condyles in adolescents across various facial height ratios, ANB angle classifications, sexes, and age categories.
Material and methods
A power analysis using G*Power (version 3.19.2; Franz Faul, Christian-Albrechts-Universitat, Kiel, Germany) was performed to estimate the sample size required for this study. To detect a pairwise difference between group means, Cohen d =.65, 40 participants per category would be required to achieve a power exceeding .80, P = 0.05, 2-tailed.
Adolescent patients (10 to <20 years) who had initial CBCT images taken in C-mode at the Department of Orthodontics, Wonkwang University Daejeon Hospital, in Iksan, Korea, were selected as participants. Images were taken by the same radiographer) using 3 guidance pins to align the heads when the patients were sitting in an upright position to obtain the Frankfort horizontal plane, which was parallel to the floor. Exclusion criteria were patients with previous orthodontic treatment, history of trauma to the dentofacial region, skeletal asymmetry, temporomandibular joint disorders, and general diseases that could influence bone tissue metabolism.
This study was conducted with 120 adolescents, 56 boys and 64 girls. They were classified into 3 groups according to age: early adolescence (10 to <14 years), middle adolescence (14 to <17 years), and late adolescence (17 to <20 years). The subjects were divided into vertical skeletal groups based on facial height ratio or Jarabak quotient, a ratio of posterior (S-Go) to anterior facial height (N-Me). These 3 groups were hypodivergent (facial height ratio, >65%), normovergent (facial height ratio, 62% to ≤65%), and hyperdivergent (facial height ratio, <62%). Patients were also categorized into 1 of 3 horizontal skeletal groups based on ANB angle: Class I (1° to 4°), Class II (>4°), and Class III (<1°) ( Table I ).
Variable | Sex | Age | FHR | ANB | |||||||
---|---|---|---|---|---|---|---|---|---|---|---|
M | F | Early | Middle | Late | Hyperdivergent | Normovergent | Hypodivergent | Class III | Class I | Class II | |
10 to <14 y | 14 to <17 y | 17 to <20 y | <62% | 62% to ≤65% | >65% | <1° | 1° to 4° | >4° | |||
Sex | |||||||||||
Male | 56 | 16 | 20 | 20 | 12 | 19 | 25 | 19 | 19 | 18 | |
Female | 64 | 24 | 20 | 20 | 27 | 16 | 21 | 12 | 18 | 34 | |
Age | |||||||||||
Early | 16 | 24 | 19 | 16 | 5 | 7 | 11 | 22 | |||
Middle | 20 | 20 | 10 | 10 | 20 | 7 | 14 | 19 | |||
Late | 20 | 20 | 10 | 9 | 21 | 17 | 12 | 11 | |||
FHR | |||||||||||
Hyperdivergent | 12 | 27 | 19 | 10 | 10 | 5 | 8 | 26 | |||
Normovergent | 19 | 16 | 16 | 10 | 9 | 10 | 11 | 14 | |||
Hypodivergent | 25 | 21 | 5 | 20 | 21 | 16 | 18 | 12 | |||
ANB | |||||||||||
Class III | 19 | 12 | 7 | 7 | 17 | 5 | 10 | 16 | |||
Class I | 19 | 18 | 11 | 14 | 12 | 8 | 11 | 18 | |||
Class II | 18 | 34 | 22 | 19 | 11 | 26 | 14 | 12 | |||
Total (each) | 120 | 40 | 40 | 40 | 39 | 35 | 46 | 31 | 37 | 52 |
The CBCT (PSR 9000N; Asahi Alphard Vega, Kyoto, Japan) images were taken in C-mode (scan size, 200 × 179 mm; voxel size, 0.39 mm; field of view, 19.97 cm). The radiologic parameters used were 80 kV(p), 60 mAs, and 17-second scan time. The CBCT data were saved as DICOM files, and Simplant Pro 2011 pack software (version 13; Materialise, Leuven, Belgium) was used to analyze the DICOM data to generate the quantitative measurements.
Institutional review board approval was granted by Wonkwang University Dental Hospital (number WKD IRB W1610/001-001) in Daejeon, Korea, to conduct this study.
Bone density of the condyles was determined on the axial view of CBCT images magnified up to 400% to clarify the morphology and boundary between the cortical and cancellous bones of the condyle. The axial slice with the largest mediolateral diameter of the mandibular condyle was selected ( Figs 1 and 2 , A ). The measurements of total, cortical, and cancellous bone densities of the condyles were performed in that axial slice bilaterally one at a time. First, total bone density was calculated by setting the extent of total condyle area using the “draw a volume” function in the 3-dimensitonal (3D) software ( Fig 2 , B ). Second, cortical bone density was calculated by removing the cancellous area from the total condyle area using the “erase a volume” function in the 3D software ( Fig 2 , C ). Third, cancellous bone density was calculated by removing the cortical area from the total condyle area using the “erase a volume” function in the 3D software ( Fig 2 , D ). The bone densities were measured with Hounsfield units (HU).


Statistical analysis
One investigator (K.J.K.) performed all measurements on the 120 subjects. To test the reliability of the measurements, 30 subjects were randomly selected for remeasurement 2 weeks after the initial measurement. The intraclass correlation coefficient showed excellent test-retest reliability of bone density measurements (0.98). The standard errors of measurement were total (0.49 HU), cortical (2.20 HU), and cancellous (0.69 HU).
SPSS software (version 23; IBM, Armonk, NY) was used for statistical analyses. Independent-samples t tests were performed to compare bone density between the 2 sex groups. Dependent-samples t tests were used to compare bone density between the right and left sides of the condyles. One-way analysis of variance with post-hoc adjustment using the Tukey honestly significant difference (HSD) test was used to compare bone density among groups in each criterion: age, facial height ratio, and ANB angle. A 2-factor analysis of variance, along with the Tukey HSD test, was used to examine differences in total mandibular condyle bone density among facial height ratios and ANB angle classifications. Chi-square analyses and follow-up Fisher exact tests were used to evaluate the relationship between facial height ratio and ANB angle. Significance was established at P <0.05, 2-tailed.
Results
The cortical ( P <0.001), cancellous ( P = 0.012), and total ( P <0.001) bone densities were significantly higher in the girls than in the boys. The cortical bone density increased as age increased. Statistically significant differences were seen between the early and middle ( P = 0.041) and early and late groups ( P = 0.031). Cortical ( P <0.001) and total ( P = 0.012) bone densities were significantly higher on the left side than on the right side ( Table II ).
Means (SD) and P values | ||||||||||
---|---|---|---|---|---|---|---|---|---|---|
Boys (n = 56) | Girls (n = 64) | P value | Early: 10 to <14 y (n = 40) |
Middle: 14 to <17 y (n = 40) |
Late: 17 to <20 y (n = 40) |
P value | Right (n = 120) | Left (n = 120) | P value | |
Cortical | 438.23 (61.13) | 490.93 (66.53) | <0.001 | 441.15 (52.27) | 478.09 (63.04) | 479.76 (82.85) | 0.017 ∗ | 449.69 (72.44) | 482.98 (79.03) | <0.001 |
Cancellous | 328.58 (49.95) | 353.01 (54.49) | 0.012 | 349.95 (43.24) | 340.33 (55.02) | 334.54 (61.24) | 0.434 | 343.31 (55.64) | 339.90 (57.40) | 0.298 |
Total | 370.18 (52.72) | 407.48 (52.88) | <0.001 | 385.39 (45.29) | 395.37 (52.09) | 389.47 (68.45) | 0.727 | 385.73 (59.13) | 394.41 (58.53) | 0.012 |
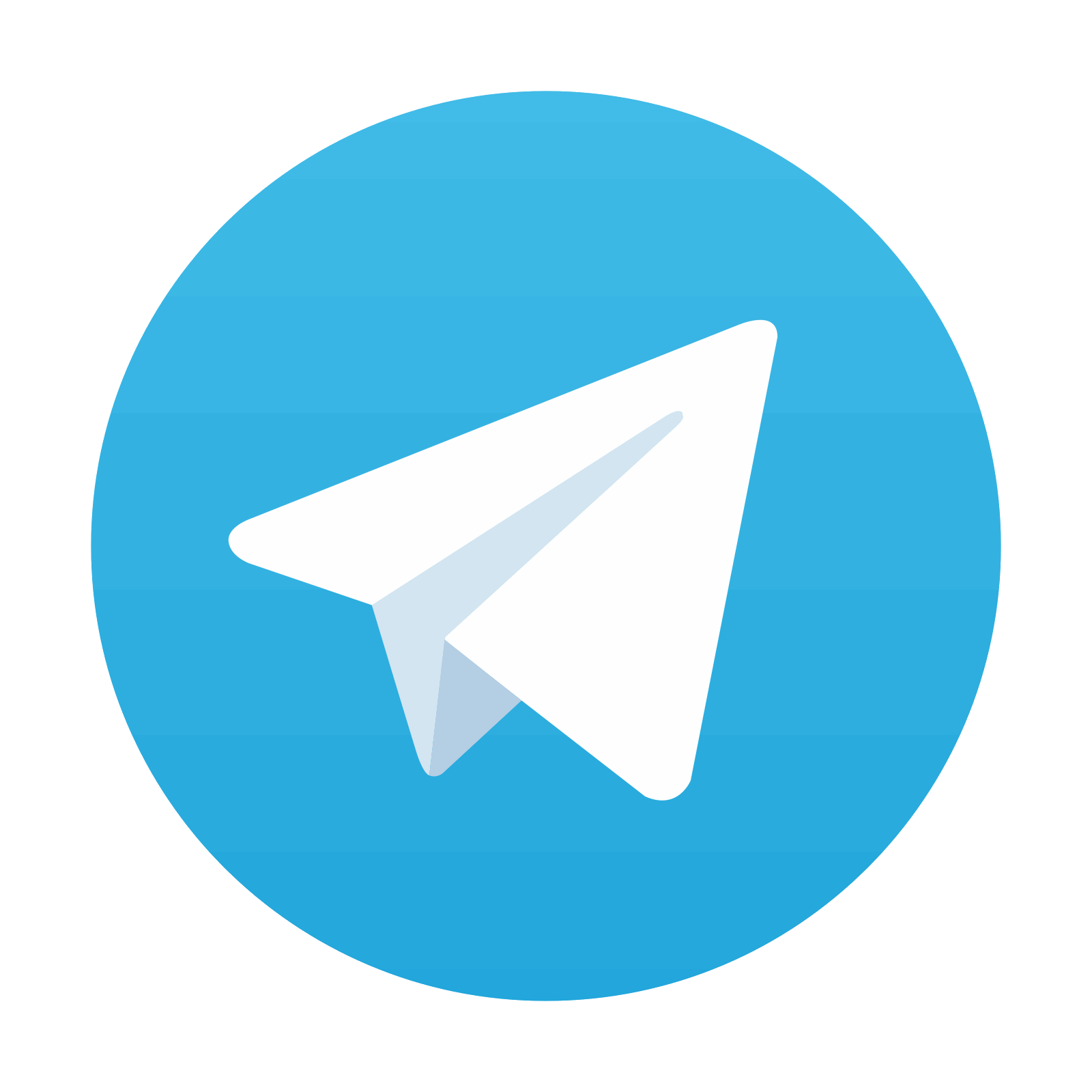
Stay updated, free dental videos. Join our Telegram channel

VIDEdental - Online dental courses
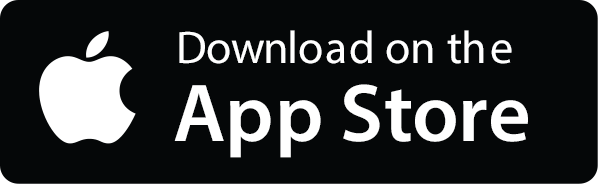
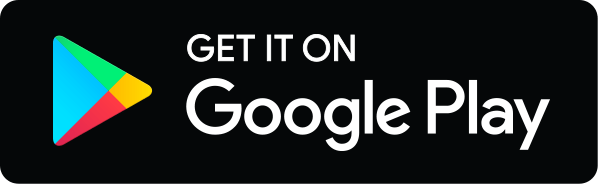
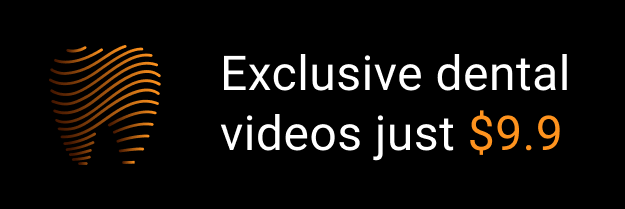