Introduction
Deepbites can be corrected by intrusion of mandibular anterior teeth. Direct anchorage with miniscrews simplifies complex tooth movements; however, few studies have reported their use for mandibular anterior intrusion. The purpose of this study was to evaluate, by means of the finite element method, initial tooth displacement and periodontal stress distribution using various mandibular anterior intrusion mechanics. Miniscrews were used as skeletal anchorage devices.
Methods
Cone-beam computed tomography scans were used for 3-dimensional reconstruction of the mandible and the mandibular anterior dentition. Models included the 4 incisors with or without the canines. After all surrounding periodontal and bony structures were determined brackets, segmental archwires, and miniscrews were added. Finite element studies were performed to assess initial tooth displacement and periodontal stress distribution with multiple intrusion force vectors. Changes in the location of the miniscrews and loading points on the archwire created 14 scenarios.
Results
Minimum buccolingual displacements, a uniform distribution of periodontal stress, and overall group intrusion for both 4-tooth and 6-tooth scenarios were best achieved when applying distointrusive vectors. The highest peaks of periodontal stress were observed when the force was directed at the corners of the segmental archwire. It was found that, in addition to distointrusive vectors, 4 loading points on the archwire were necessary for pure intrusion and uniform distribution of periodontal stress in the 6-tooth scenarios.
Conclusions
The simulations in this study suggest that group intrusion of all 6 mandibular anterior teeth might be achieved by applying distointrusive vectors. Inserting a pair of miniscrews distal to the canine roots, 1 screw per side, and directing 4 loading points on the archwire generates uniform periodontal stress distribution and minimum buccolingual displacements. Local conditions, such as narrow bone width and attached gingiva level, play significant roles in the clinical viability of the proposed virtual scenarios.
Highlights
- •
Simultaneous intrusion of mandibular teeth is possible by applying distointrusive vectors.
- •
This can be done by placing miniscrews distal to canine roots and directing 4 load points.
- •
This method was analyzed with the finite element method.
- •
Uniform periodontal tension and minimum buccolingual displacement were found.
Deepbite treatment depends on its etiology. The decision to intrude anterior teeth for overbite correction is based on the patient’s skeletal convexity, vertical dimension, and interocclusal space, among other factors. Burstone developed appliances for maxillary anterior intrusion. His mechanics delivered light, continuous, and single-point forces.
The introduction of skeletal anchorage has simplified complex tooth movements. Miniscrews offer the advantages of immediate loading, multiple insertion sites, simple mechanics, and cost-effectiveness. When compared with utility arches, anchorage with minsicrews prevents secondary movements of molars, and the force passes closer to the center of resistance, resulting in pure maxillary incisor intrusion.
Maxillary incisor intrusion might flatten the smile arc, alter tooth-lip exposure, and reduce smile attractiveness. Mandibular anterior intrusion, on the other hand, could prevent such a compromise in dental esthetics and focus treatment on the predominant mandibular nature of a deepbite.
Current use of miniscrews in orthodontics is widespread. However, research on temporary anchorage devices has not achieved standardization, and rates of success or failure in clinical practice are still undetermined. There are currently few reports describing mandibular anterior intrusion mechanics with skeletal anchorage.
Approximately 50% of patients with deepbite have extruded mandibular canines. A single manipulation of these teeth is suggested to prevent posterior anchorage loss. It is still unclear whether the use of miniscrews allows for simultaneous intrusion of mandibular incisors and canines; moreover, adding mandibular canines could distally displace the center of resistance.
Finite element analysis is a helpful resource for assessing the virtual biomechanics of the possible clinical outcomes. Its reliability depends on model design and selected mechanical properties of tissues and appliances. There are substantial differences between computerized simulations and biologic reality; however, finite element methods have been proven to be helpful in creating an accurate virtual panorama, which could be reproduced in clinical practice.
The purpose of this study was to assess, with 3-dimensional modeling and finite element analysis, initial periodontal stress distribution and displacement of mandibular anterior teeth under various intrusion mechanics using miniscrews as direct anchorage devices. The tested hypothesis was that specific arrangements between miniscrew insertion sites and loading points on the archwire create force vectors that pass closer to a distally displaced center of resistance and allow for pure group intrusion of 6 mandibular anterior teeth.
Material and methods
Cone-beam computed tomography mandibular scans of a 25-year-old woman were available to us. The scans had been previously obtained for restorative purposes. They were selected because they complied with skeletal and dental Class 1 occlusion, proper overbite and overjet, and incisors positioned at standard cephalometric values. A total of 128 layered slices with an approximate voxel size of 0.4 × 0.4 × 0.4 mm were saved in DICOM format. All images were imported into InVesaluis software (CTI Renato Archer, Campinas, Brazil). Bone and teeth were isolated from other structures. Segmentation operations were performed to reduce noise and artifacts ( Fig 1 ).

A 3-dimensional mandibular model, including the incisors, with or without the canines, was imported into SolidWorks software (Dassault Systèmes, Suresenes, France). A cloud of points was performed. Manual design of the miniscrew was based on a conical, self-drilling device with a length of 6 mm and a thread diameter of 1.6 mm (Ortho Easy; Forestadent, Pforzheim, Germany).
Manually designed brackets with a 0.021 × 0.025-in stainless steel segmental archwire were attached to the teeth forming a rigid unit; no clearance between slot and archwire was assumed.
Finite element models were constructed by importing solid models into Ansys software (Swanson Analysis Systems, Canonsburg Pa). A shell 0.25-mm thick surrounding every root of all 6 teeth was designed to act as a periodontal ligament (PDL) ( Fig 2 , A and B ). Four-noded tetrahedron elements were used. Each model included a total of 1,036,000 elements and 3,442,000 nodes, approximately.

Mechanical properties of tissues and orthodontic appliances were selected based on published data ( Table I ). All materials in this study were assumed to be linearly elastic, homogeneous, and isotropic.
Material | Elastic modulus E (GPa) |
Poisson’s ratio v |
---|---|---|
Trabecular bone | 1.5 | 0.30 |
Cortical bone | 14.7 | 0.30 |
Tooth | 20.7 | 0.30 |
Miniscrew (Ti 6 Al 4 V) | 114 | 0.34 |
PDL (linear model) | 6.89 × 10 −5 | 0.45 |
Stainless steel | 193 | 0.29 |
Boolean subtraction operations were performed to simulate accurate drilling holes necessary for miniscrew insertion ( Fig 2 , C ). To simplify modeling, 6 miniscrews were inserted at once, distal to the roots of the mandibular incisors and canines.
To obtain easier isolation of the implant bone block, the miniscrews were inserted at a 90° angle with respect to the bone surface. Insertion depth stopped at the transmucosal transition of the screw body ( Fig 3 , A ). Implants were placed approximately 10 mm below the alveolar ridge. This distance allowed compliance with safety parameters regarding the amount of bony structure surrounding a miniscrew inserted between 2 roots ( Fig 3 , B ). Vestibular interdental bone was measured at each side of the miniscrew, from its surface to the PDL. The following values were obtained at each side of the implant: 0.5 mm for both central-lateral incisors and lateral incisor-canine, and 0.7 mm for canine-first premolar. The cortical bone width was also measured at insertion sites: 2.1 mm for central-lateral incisors, 2.0 mm for lateral incisor-canine, and 2.16 mm for canine-first premolar.

Light, continuous intrusion forces of 80 and 100 g were applied on the segmental archwires, according to classical guidelines. Virtual force vectors travelled from the miniscrew head to the different points on the archwire.
Fourteen scenarios were produced by selecting a pair of miniscrews used for anchorage and changing loading points on the archwire. Six scenarios included only incisors, with 2 or 4 loading points on the segmental archwire ( Fig 4 ). Another set of 8 scenarios, including incisors and canines, is shown in Figure 5 . Force was applied bilaterally and symmetrically. Periodontal stress distributions and initial tooth displacements were assessed.


Results
In 4-tooth mechanics, it was found that maximum peaks of stress appeared when the loading was applied at the corners of the archwire (scenarios b and d; Table II ). The PDL of teeth far from the loading source showed lower stress values. When a distointrusive vector was applied, as in case f, stress distribution improved for all teeth involved ( Fig 6 ).
Tooth group | Scenario | Maximum Von Mises values in PDL (MPa) |
---|---|---|
Mandibular incisors | a | 0.223 |
b | 0.408 | |
c | 0.305 | |
d | 0.442 | |
e | 0.303 | |
f | 0.185 | |
Mandibular incisors and canines | g | 0.292 |
h | 0.533 | |
i | 0.589 | |
j | 0.518 | |
k | 0.452 | |
l | 0.213 | |
m | 0.315 | |
n | 0.256 |
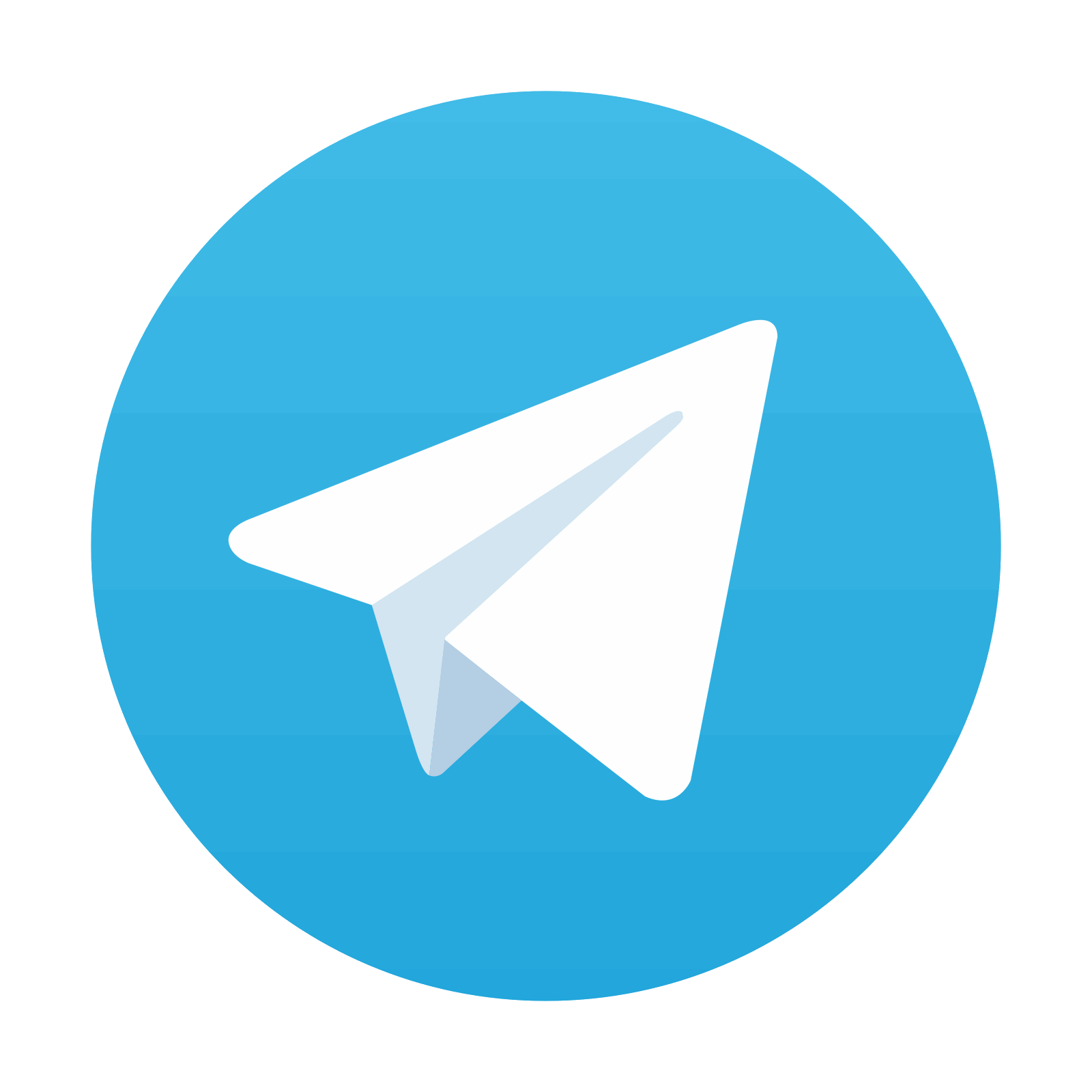
Stay updated, free dental videos. Join our Telegram channel

VIDEdental - Online dental courses
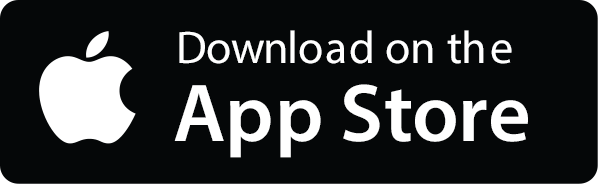
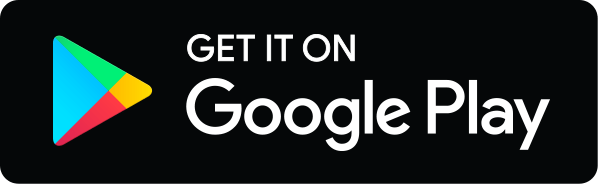
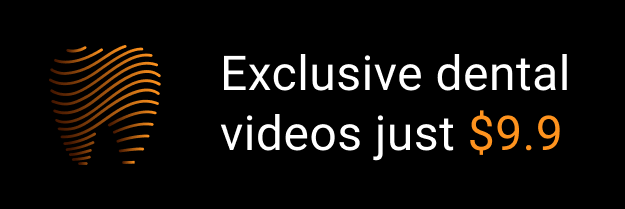