Low level light therapy (LLLT) with light-emitting diodes (LEDs) is emerging from the mists of black magic as a solid medico-scientific modality, with a substantial buildup of corroborative bodies of evidence for its efficacy and elucidation of the modes of action. Reports are appearing from many different specialties; however, of particular interest to plastic surgeons treating the aging face is the proven action of LED-LLLT on skin cells in both the epidermis and dermis and enhanced blood flow. Thus, LED-LLLT is a safe and effective stand-alone therapy for patients who are prepared to wait until the final effect is perceived.
Key points
- •
Light-emitting diodes–low level laser therapy (LED-LLLT) does not deliver coherent energy as do lasers and laser diodes, but it does deliver highly narrow-band quasimonochromatic light with more than 95% of the photons at the rated wavelength allowing for precise chromophore targeting.
- •
LED-LLLT need not be delivered by a clinician, but can be given by a trained nurse or therapist under clinician supervision thereby freeing up the clinician for other duties.
- •
LED-LLLT is easy to deliver, is safe, effective, pain and side-effect free, and is well tolerated by patients of all ages from infants to centenarians.
Low level light therapy
The acronym LLLT originally stood for low level laser therapy , which was first coined by Ohshiro and Calderhead in 1988. It is a term that has come in for a fair amount of misinterpretation and abuse, with investigators often talking about “low level lasers,” for example. The original concept of LLLT was focused on the therapeutic effect induced in the target tissue by the incident photons, irrespective of the system generating these photons.
Photons and Photon Intensity
Photons are discrete particles of pure light energy without mass. The first law of photobiology states that without absorption, there can be no reaction, so the incident photon must be absorbed by the target cells for any reaction to occur. When a photon is absorbed by a cell, it passes on its energy to the cell. The degree of the photon density determines what sort of energy transfer occurs. In parallel with the Ohshiro-Calderhead Arndt-Schultz curve, which illustrates the level of biological activity depending on the strength of the stimulus ( Fig. 1 ), low photon intensities excite the cell, moderate ones sustain the cell, strong intensities will damage the cell through the generation of a photothermal reaction, retarding cellular activities, and very strong ones will kill the cell.

Photoactivation Versus Photodestruction
If we think of a cell, as shown schematically in Fig. 2 , we can assign to each cell 2 thresholds based on the aforementioned concept: the damage threshold and the survival threshold. As the level of stimulus increases, the level of the reaction in the cell increases. If the level of reaction is below the damage threshold of the cell (see Fig. 2 A), the thermal reaction, if any, is negligible and the photo-biomodulation or photoactivation of the cell and its activity occurs. This activity takes 3 forms: if photoactivated cells are damaged or compromised in some way, they will be repaired; if they have a function to do, for example, fibroblast synthesis of collagen and elastin, they will perform that function better and faster; if there are not enough cells, then more will be recruited or the existing cells will proliferate. These actions can happen singly or in combination. The above concepts explain the level of low level laser therapy: it refers to the level of the reaction induced in the cell by the incident light energy. This level of reaction can be classed as phototherapy, whereby some form of clinical effect is achieved through photo-biomodulation of the cell actions but without heat or damage.

What Systems Can Deliver Low Level Light Therapy?
In the late 1980s, the only clinically appropriate sources available for true phototherapy were low-powered laser diodes or defocused surgical lasers. Light-emitting diodes (LEDs) were available; but they were totally inappropriate for clinical indications, having low and unstable output powers, large angles of divergence, and wide wavebands. It may come as somewhat of a surprise to see that surgical lasers could, and still can, be used for phototherapy. As Table 1 illustrates, a high-level laser can be used for LLLT and a low-level laser can be used to deliver high-level light therapy with powerful thermal damage. Terminology, therefore, matters because it is the level of the tissue reaction that is important and not the level of the laser or light source delivering the beam.
Laser Type | Output Power (Units as Shown) | Spot Size at Tissue (Units as Shown) | Power Density | Level of Tissue Reaction |
---|---|---|---|---|
CO 2 | 60 W | 15 cm | 0.8 W/cm 2 | Therapeutic (LLLT) |
Diode | 60 mW | 50 μm | 3000 W/cm 2 | Destructive (HLLT) |
Low level light therapy
The acronym LLLT originally stood for low level laser therapy , which was first coined by Ohshiro and Calderhead in 1988. It is a term that has come in for a fair amount of misinterpretation and abuse, with investigators often talking about “low level lasers,” for example. The original concept of LLLT was focused on the therapeutic effect induced in the target tissue by the incident photons, irrespective of the system generating these photons.
Photons and Photon Intensity
Photons are discrete particles of pure light energy without mass. The first law of photobiology states that without absorption, there can be no reaction, so the incident photon must be absorbed by the target cells for any reaction to occur. When a photon is absorbed by a cell, it passes on its energy to the cell. The degree of the photon density determines what sort of energy transfer occurs. In parallel with the Ohshiro-Calderhead Arndt-Schultz curve, which illustrates the level of biological activity depending on the strength of the stimulus ( Fig. 1 ), low photon intensities excite the cell, moderate ones sustain the cell, strong intensities will damage the cell through the generation of a photothermal reaction, retarding cellular activities, and very strong ones will kill the cell.
Photoactivation Versus Photodestruction
If we think of a cell, as shown schematically in Fig. 2 , we can assign to each cell 2 thresholds based on the aforementioned concept: the damage threshold and the survival threshold. As the level of stimulus increases, the level of the reaction in the cell increases. If the level of reaction is below the damage threshold of the cell (see Fig. 2 A), the thermal reaction, if any, is negligible and the photo-biomodulation or photoactivation of the cell and its activity occurs. This activity takes 3 forms: if photoactivated cells are damaged or compromised in some way, they will be repaired; if they have a function to do, for example, fibroblast synthesis of collagen and elastin, they will perform that function better and faster; if there are not enough cells, then more will be recruited or the existing cells will proliferate. These actions can happen singly or in combination. The above concepts explain the level of low level laser therapy: it refers to the level of the reaction induced in the cell by the incident light energy. This level of reaction can be classed as phototherapy, whereby some form of clinical effect is achieved through photo-biomodulation of the cell actions but without heat or damage.
What Systems Can Deliver Low Level Light Therapy?
In the late 1980s, the only clinically appropriate sources available for true phototherapy were low-powered laser diodes or defocused surgical lasers. Light-emitting diodes (LEDs) were available; but they were totally inappropriate for clinical indications, having low and unstable output powers, large angles of divergence, and wide wavebands. It may come as somewhat of a surprise to see that surgical lasers could, and still can, be used for phototherapy. As Table 1 illustrates, a high-level laser can be used for LLLT and a low-level laser can be used to deliver high-level light therapy with powerful thermal damage. Terminology, therefore, matters because it is the level of the tissue reaction that is important and not the level of the laser or light source delivering the beam.
Laser Type | Output Power (Units as Shown) | Spot Size at Tissue (Units as Shown) | Power Density | Level of Tissue Reaction |
---|---|---|---|---|
CO 2 | 60 W | 15 cm | 0.8 W/cm 2 | Therapeutic (LLLT) |
Diode | 60 mW | 50 μm | 3000 W/cm 2 | Destructive (HLLT) |
The light-emitting diode
In the past decade and a half, LEDs have become accepted sources for therapeutic systems. The year 1988 was the pivotal year for LEDs, when Professor Harry Whelan and his team at the Space Medicine Laboratory of the National Aeronautic and Space Administration (NASA) developed the first of a new generation of LEDs, the so-called NASA LED. The same group quickly went on to demonstrate the clinical efficacy of near-infrared LEDs for wound healing, and the new-generation LED was available to researchers and clinicians as a valid therapeutic light source. In fact, at the time of writing, LEDs are now supplanting laser diode-based systems for many applications; it was suggested some 10 years ago by Kendric C Smith, Emeritus Professor at Stanford University and a leading photobiologist, that low level laser therapy should become low level light therapy but share the same acronym of LLLT. That is now the case.
What Is a Light-Emitting Diode?
At the heart of LEDs are tiny semiconductor chips. When a DC current is applied to an LED, light (photons) is emitted in an uninterrupted elliptical cone with divergence usually in the range of 60° to 110° across the larger diameter of the beam. Fig. 3 illustrates the construction of the older and current form of LEDs. The light from LEDs is completely noncoherent, but in high-grade LEDs a very high percentage of the photons are at the rated wavelength. The higher the grade of the LED, the narrower the bandwidth of the emitted photons and is defined as quasimonochromaticity , allowing LEDs to emit the rated wavelength plus or minus a very few nanometers.
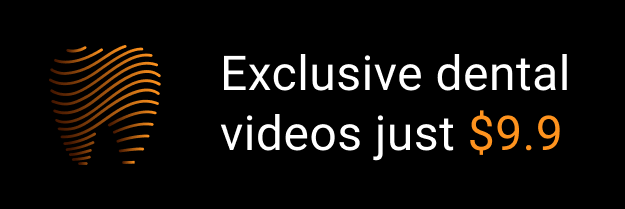