Introduction
In orthodontic treatment, teeth can relapse after tooth movement without retention. The aim of this study was to evaluate the inhibition effects of local osteoprotegerin (OPG) gene transfer on orthodontic relapse.
Methods
Eighteen male Wistar rats were divided into 3 groups. The maxillary right first molars of all animals were subjected to orthodontic force and moved mesially. Three weeks later, the force was removed, and the teeth relapsed. During the 2-week relapse period, the 3 groups of rats received local OPG gene transfer (experimental group), mock vector transfer (mock group), and no injections (control group). Tooth movement and relapse were measured by using palatal superimpositions of 3-dimensional digital models. Histomorphometric analysis was used to quantify osteoclasts, and microcomputed tomography analysis was done to quantify the alveolar bone and the tibia.
Results
Relapse was significantly inhibited and the number of osteoclasts was reduced in the experimental group. On the other hand, bone mineral density and bone volume fraction of alveolar bone were significantly increased. Bone mineral density and bone volume fraction of the tibia showed no significant difference between the groups.
Conclusions
Local OPG gene transfer to periodontal tissues could inhibit relapse after orthodontic tooth movement, through the inhibition of osteoclastogenesis.
Achallenging problem in orthodontics is tooth relapse. After orthodontic tooth movement, a retainer is needed to maintain teeth in their corrected positions until the treatment result becomes more stable. Without a phase of retention, there is a tendency for teeth to return toward their initial positions. The reasons behind relapse are unclear, although several factors have been suggested, including the recoil of gingival and periodontal tissues, the surrounding soft tissues, growth of skeletal bases, and other dental factors. In this study, we focused on the periodontal supporting tissues, especially the remodeling of surrounding alveolar bone.
During active orthodontic tooth movement, stresses and strains are thought to be built up and stored in the periodontal and transseptal fiber system. After removal of the orthodontic appliance, these stresses are released, and the teeth begin to relapse to their original positions. However, even though the periodontal fiber is primarily responsible for the generation of forces on moved teeth, osteoclastic resorption and osteoblastic formation of surrounding alveolar bone are necessary for relapse to occur. It has been reported that relapse pressure persisted until the alveolar bone resorption was completed, and relapse of moved teeth can be prevented by properly manipulating alveolar bone remodeling after orthodontic tooth movement. Administration of bisphosphonate has been reported to inhibit bone resorptive functions of osteoclasts and reduce relapse. Simvastatin can prevent relapse through inhibition of the bone-resorbing activity of osteoclasts and by stimulating bone formation. Bone morphogenetic proteins have been used to prevent relapse, and the results showed that they can promote new bone and cementum formation. These data suggest that relapse could be inhibited by manipulating alveolar bone remodeling.
The axis consisting of receptor activator of nuclear factor kappa B (RANK)/RANK ligand (RANKL)/osteoprotegerin (OPG) has recently been shown to play an important role in orthodontic bone remodeling. RANKL, a member of the tumor necrosis factor superfamily, is produced by osteoblast lineage cells, periodontal ligament cells, and T lymphocytes. RANKL binds to its receptor RANK, which is located on the surface of osteoclasts and osteoclast precursors. RANK-RANKL interactions lead to preosteoclast recruitment, fusion into multinucleated osteoclasts, osteoclast activation, and osteoclast survival. Each of these responses can be fully inhibited by OPG, which is a soluble decoy receptor against RANKL and produced by osteoblasts and other cells.
An increase in exogenous OPG expression can lead to the indirect inhibition of bone resorption and loss. This inhibition has been observed in many bone destruction-related diseases including rheumatoid arthritis, primary osteoprosis, postmenopausal bone loss, periodontal disease, and others. It has also been shown that exogenous OPG has an inhibitory effect on orthodontic tooth movement. When the fusion protein OPG-Fc was locally delivered, inhibition of osteoclastogenesis and active orthodontic tooth movement was observed. Previously, we had reported that local OPG gene transfer to periodontal tissues significantly diminished, whereas RANKL gene transfer significantly enhanced, orthodontic tooth movement. OPG expression in periodontal tissues was observed to increase significantly after local OPG gene transfer; this was followed by RANKL-mediated osteoclastogenesis inhibition. As a result, the experimental orthodontic tooth movement in rats was inhibited without eliciting any systemic effects. Simvastatin can inhibit relapse by enhancing the expression of OPG and reducing the expression of RANKL. These data suggested the possibility of manipulating bone remodeling with local OPG gene transfer to inhibit postorthodontic relapse.
In this study, we hypothesized that local OPG gene transfer to periodontal tissues would inhibit the relapse after orthodontic tooth movement. The specific objectives of this study were to assess the magnitude of relapse after orthodontic tooth movement in rats with or without local OPG gene transfer, and to determine the effects of local OPG gene transfer on the bone mineral density (BMD) and the bone volume fraction (BVF) of the alveolar bone (local effects) and the tibia (systemic effects) through microcomputed tomography analysis.
Material and methods
Eighteen male Wistar rats (age, 6 weeks; approximate weight, 180-190 g) were used in this study. Six-week-old rats were considered as adolescent, since male rats reach sexual maturity at 8 weeks of age. All rats were housed under normal conditions with a 12-hour circadian cycle and fed a standard rat-chow diet and water ad libitum. The experimental procedures were approved by the Ethics Committee of Peking University Health Science Center, Beijing, China.
The 18 rats were randomly divided into 3 equal groups: experimental, mock, and control groups. The maxillary right first molars of all animals were subjected to orthodontic force and moved mesially by a closed-coil nickel-titanium spring (Sentalloy, Tomy, Tokyo, Japan). The spring was ligated to the maxillary right first molar and incisor, exerting a force of approximately 40 g ( Fig 1 , A ). The activation force of the spring was evaluated with a force gauge, and the spring could provide a force of 40 ± 2 g at 3.2 mm of activation. Three weeks later, the springs were removed, and the teeth were left without retainers. This allowed relapse of the mesialized molars to occur by returning toward their distal positions. At the time of spring removal, the 3 groups of rats received different treatments: local OPG gene transfer (experimental group), mock vector transfer (mock group), and no injections (control group). The duration of relapse was 2 weeks.
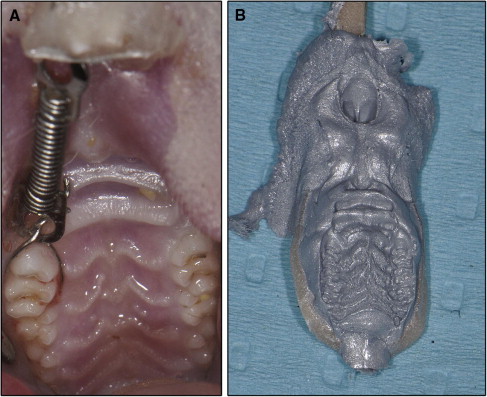
For in-vivo gene transfer, we used an inactivated hemagglutinating virus of Japan (HVJ) envelope vector (GenomONE, Ishiara-sangyo kaisha, Osaka, Japan) and an OPG expression plasmid. The cloning of the mouse OPG gene and details of the OPG expression plasmid (pcDNA3.1(+)-mOPG) have been described previously, and functional in-vitro protein expression has been confirmed. The rats of the experimental group were administered the HVJ envelope vector, which contained the pcDNA-mOPG plasmid, on the initial day of relapse. Under anesthesia, 5 μL of vector solution were injected into the palatal mucosa adjacent to the distal surface of the maxillary right first molar, by using 26s-gauge microneedles (Hamilton, Reno, Nev). All injections were volumetrically equivalent and were administered twice weekly. After 2 weeks, the animals were killed.
The distances of tooth movement and relapse were measured by palatal superimposition of 3-dimensional (3D) digital models. Silicone (Affinis precious light body, Coltene Whaledent, Altstatten, Switzerland) impressions were taken of the rats’ maxillary dentitions at 3 times: baseline (pretreatment impression), the last day of the 3-week tooth movement (posttreatment impression), and the last day of the 2-week relapse (relapse impression; Fig 1 , B ). After the fabrication of precise stone models, 3D scanning of the maxillary dental casts was performed by using a 3D spot laser scanner (LPX-1200, Roland, Shizuoka, Japan). The validity and precision of the scanner were 0.05 mm. The scanned data were used to reconstruct and analyze the 3D images; this was performed by using reverse engineering software (Rapidform 2006; Inus Technology, Seoul, Korea). After reconstruction and analysis, the pretreatment, posttreatment, and relapse models were superimposed on the area of the palatal rugae ( Fig 2 , A-D ). After superimposition, the amounts of tooth movement and relapse were calculated by using the software Rapidform 2006. First, the occlusal plane was chosen as the reference plane ( Fig 2 , E ). Then the midpoints of the mesial marginal ridge of the maxillary right first molars were marked on the pretreatment, posttreatment, and relapse models. The distances between these points were measured on the reference plane ( Fig 2 , F ). All measurements were repeated 3 times by 2 investigators (N.Z. and W.L.).
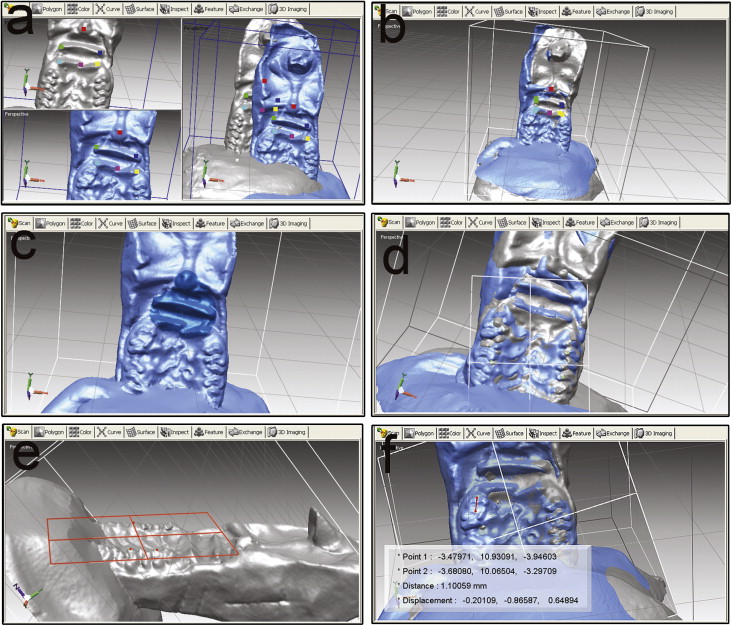
To evaluate the effect of 5-week growth on the stability of the rats’ palatal surfaces, we performed the following preliminary experiment. Three rats received no orthodontic forces. For each rat, the maxilla models at baseline and subsequent growth after 5 weeks were scanned and superimposed on the area of the palatal rugae. The results showed that the 3D positions of landmarks on the palatal surface were stable.
Microcomputed tomography analysis was performed to quantify alveolar bone in the proximity of the first molar roots. It was also used to measure the BMD and the BVF of the tibia ( Fig 3 ). Density and volume fraction are important parameters in describing the status of bone metabolism. BVF is the fraction of solid bone volume to total volume, which is measured to evaluate the trabecular microstructure. The accuracy of BVF and BMD measured by microcomputed tomography has been confirmed in previous studies.
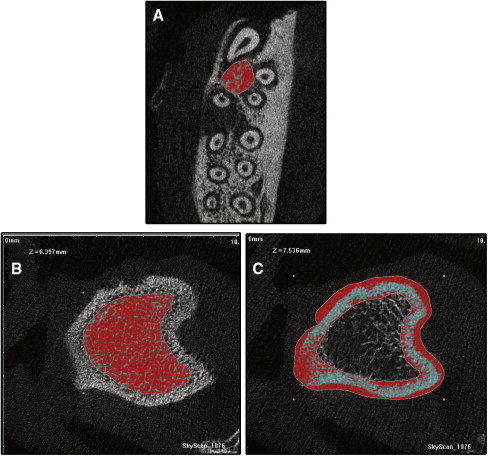
In this experiment, the head and left tibia of each study animal were scanned, under general anesthesia, with an x-ray microtomography system (model 1076; SkyScan, Kontich, Belgium). The BMD and BVF of the alveolar bone and the tibia were analyzed by using the system’s software. The image voxel sizes of alveolar bone and tibia were 9.488 and 18.97 μm, respectively. All measurements were repeated 3 times by 2 investigators.
BMD and BVF of alveolar bone were measured in the first molar furcation area. The furcation area was chosen since it provides reproducible morphologic landmarks.
The animals were killed under pentobarbital anesthesia 2 weeks after spring removal. After final impressions and scanning with microcomputed tomography, block biopsies of the maxillae were harvested, immediately fixed, and stored in 10% neutral-buffered formalin solution for 24 hours at 4°C. The maxillae, including the molars, were dissected and decalcified by using 10% ethylene diamine tetra-acetic acid for 4 weeks at 4°C before being embedded in paraffin. Horizontal specimens were obtained from the root of the maxillary first molar. The sections were stained with hematoxylin and eosin for descriptive histology. The OPG immunohistochemical staining was performed to identify the OPG gene transfection. A minimum of 6 randomly selected slides per animal were deparaffinized and incubated overnight with the anti-OPG antibody (1:200 dilution; sc-8468; Santa Cruz Biotechnology, Santa Cruz, Calif) at 4°C. The anti-OPG antibody can recognize both mouse and rat OPG. The slides were stained with the 2-step plus poly-HRP anti-goat IgG detection system (ZSGB-Bio, Beijing, China), followed by color development with diaminobenzidine. The intensity of immunohistochemistry was measured with the software Image-Pro Plus (version 6.0; Media Cybernetics, Bethesda, Md). Tartrate-resistant acid phosphatase (TRAP) staining of the sections at the bifurcation level was performed with a leukocyte acid-phosphatase kit (387A-1KT; Sigma-Aldrich, St Louis, Mo). Counting was performed of TRAP-positive multinucleated cells that formed resorption lacunae on the alveolar bone surface adjacent to the distopalatal root of the maxillary right first molar.
Statistical analysis
All data were expressed as means ± standard deviations. Statistical significance was calculated by 1-way analysis of variance (ANOVA) and the least-significant difference test. Differences with a P value less than 0.05 were considered significant.
Results
The orthodontic appliance and force only slightly affected the animals’s weights during the first 3 days. There were no significant differences in weight gain among the groups. The local injections did not affect the rats’ growth during the 2-week relapse. Twice-weekly local OPG gene transfer seemed to cause no appreciable macroscopic changes, such as edema, redness, or erosion at the local injection site.
Local OPG gene transfer resulted in substantial relapse of the first molar tooth movement when compared with the animals in the mock and control groups ( Figs 4 and 5 ). The distances of tooth movement and the relapse of the first molars in the 3 groups are shown in Figure 4 . After 3 weeks of force application, tooth movement of the first molars was about 1.57 to 1.59 mm, and there were no significant differences between the groups. After 2 weeks of relapse, the distance of relapse in the experimental group (0.55 ± 0.13 mm) was reduced significantly ( P <0.001) when compared with the control (1.53 ± 0.23 mm) and mock (1.31 ± 0.39 mm) groups. The percentage of relapse (distance of relapse/distance of tooth movement) in the experimental group (35.7% ± 8.9%) was significantly ( P <0.001) less than in the mock group (82.3% ± 10.0%) and the control group (96.3% ± 7.0%). No statistical significance was observed between the control and mock groups. These results suggest that RANKL-dependent osteoclast regulation plays a role not only in orthodontic tooth movement, but also in relapse.
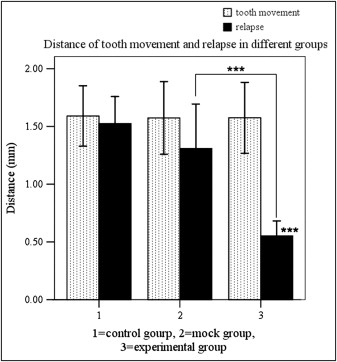
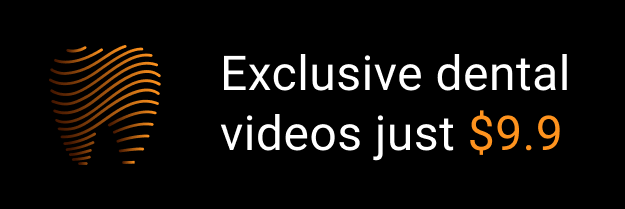