Lipid metabolism
Publisher Summary
This chapter discusses lipid metabolism. The digestion and absorption of fats pose considerable problems because of their insolubility in water. The fat splitting enzymes or lipases are water-soluble, and can only operate at the interface between lipid droplets and the aqueous phase. For this reason, the degree of dispersion or emulsification is very important in ensuring that fat is adequately digested and absorbed. Lipases are secreted both in the stomach and in the pancreatic juice. The value of gastric lipase is doubtful as its optimum pH is 8–0 whereas the pH of the normal adult stomach is 1.0–2.0. The process of emulsification of other fats is probably initiated by the churning motion of the stomach and is greatly facilitated when the chyme passes into the duodenum, and is mixed with the bile and pancreatic juice. These secretions, which are both alkaline, neutralize the acid from the stomach so that conditions become suitable for the action of pancreatic lipase. Bile contains no enzymes but owes its effects to the presence of the bile salts, sodium glycocholate, and sodium taurocholate, which are powerful detergents. The bile salts are derived from cholic acid, a sterol, which is joined by a peptide linkage either to glycine or to taurine. Lipase is relatively unspecific in its action as it is little affected by either the degree of saturation or the chain length of the fatty acids present in the glyceride. It acts preferentially to remove the fatty acid present in the a-position, so that the first product is an α, β-diglyceride.
< ?xml:namespace prefix = "mml" />
Lipase is relatively unspecific in its action since it is little affected by either the degree of saturation or the chain length of the fatty acids present in the glyceride. It acts preferentially to remove the fatty acid present in the α-position, so that the first product is an α,β-diglyceride. The fatty acid in the second α-position is then removed to give a β-monoglyceride. Hydrolysis of the monoglyceride probably only occurs after isomerization, involving transfer of the remaining acyl group to one of the α-positions (Figure 18.1). The first, second and third fatty acids are removed with increasing difficulty, so that monoglycerides are a major product of fat digestion and small amounts of diglyceride also remain. It appears that only about 30−40% of the triglycerides are completely hydrolysed to glycerol and fatty acids.
Absorption of fat
The fatty acids and monoglycerides released from micelles are resynthesized into triglycerides within the epithelial cells, the mechanism of resynthesis being similar to that for triglyceride synthesis in adipose tissue (page 258). It should be noted that triglycerides that accumulate in the intestinal mucosal cells during fat absorption are different from those originally present in the food, both with respect to the arrangement of their fatty acids and the origin of the glycerol part of the molecule. Glycerol in totally resynthesized fat is derived from intermediates of glycolysis and not from the original glyceride.
Body fats
Triglycerides are peculiarly well suited to their role as the main food reserve since:
1. On oxidation they produce more than twice the amount of energy released during the oxidation of an equivalent weight of carbohydrate or protein (page 122).
2. Unlike protein and glycogen, fat can be laid down without any concomitant storage of water so that fat depots are compact highly concentrated energy stores.
3. The metabolism of fat produces more water than either protein or carbohydrate

The oxidation of fat


Once inside the mitochondrion the acyl group is tranferred back to CoA. Thus the extra- and intra-mitochondrial supplies of CoA are kept separate (see Figure 18.5).
Oxidation of fatty acyl-CoA compounds occurs by a well-recognized pattern of reactions similar to those occurring at the four-carbon stages of the citrate cycle. Thus oxidation of the β-carbon atom as shown in Figure 18.2 occurs by: (1) removal of 2H to give an unsaturated derivative; (2) addition of H2O to give a β-hydroxy derivative; (3) removal of 2H to give a β-keto derivative.
The energy yield from fatty acid oxidation
7 β-oxidations each producing 5 molecules of ATP | + 35 |
Oxidation of 8 molecules of acetyl-CoA each producing 12 molecules of ATP | +96 |
Less 2 ATP equivalents expended during the initial fatty acid activation and conversion of ATP to AMP | − 2 |
— | |
Net gain 129 |
If the ΔG of hydrolysis of ATP is taken as −31 kJ (−7·3 kcal) then the energy conserved


Fatty acid synthesis
As a result of these reactions when energy supplies are plentiful, reducing power (NADH), obtained during the conversion of glucose to pyruvate by the glycolytic pathway, instead of being used for further energy production can be transferred to NADP+ and used for fat synthesis and energy storage. One NADPH is generated for every acetyl unit that passes into the cytosol from the mitochondria but the synthesis of 1 mol of palmitate requires 14 mol of NADPH (see below) and the remaining 6 are obtained from the pentose phosphate pathway. The pyruvate passes back into the mitochondria and is used either for regeneration of oxaloacetate or for conversion to acetyl-CoA (Figure 18.5).
In the first reaction of fatty acid synthesis acetyl-CoA is converted to malonyl-CoA by the very important enzyme, acetyl-CoA carboxylase which contains biotin (page 166) as its prosthetic group. ATP is needed to provide energy for the carboxylation and magnesium ions are also required:
Acetyl-CoA carboxylase is an allosteric enzyme and the reaction is the primary regulating step in fatty acid synthesis (page 341). It is also regulated through phosphorylation/dephosphorylation reactions which modify specific serine residues on the protein (page 344).

As in gluconeogenesis, alternate carboxylation and decarboxylation reactions are involved.
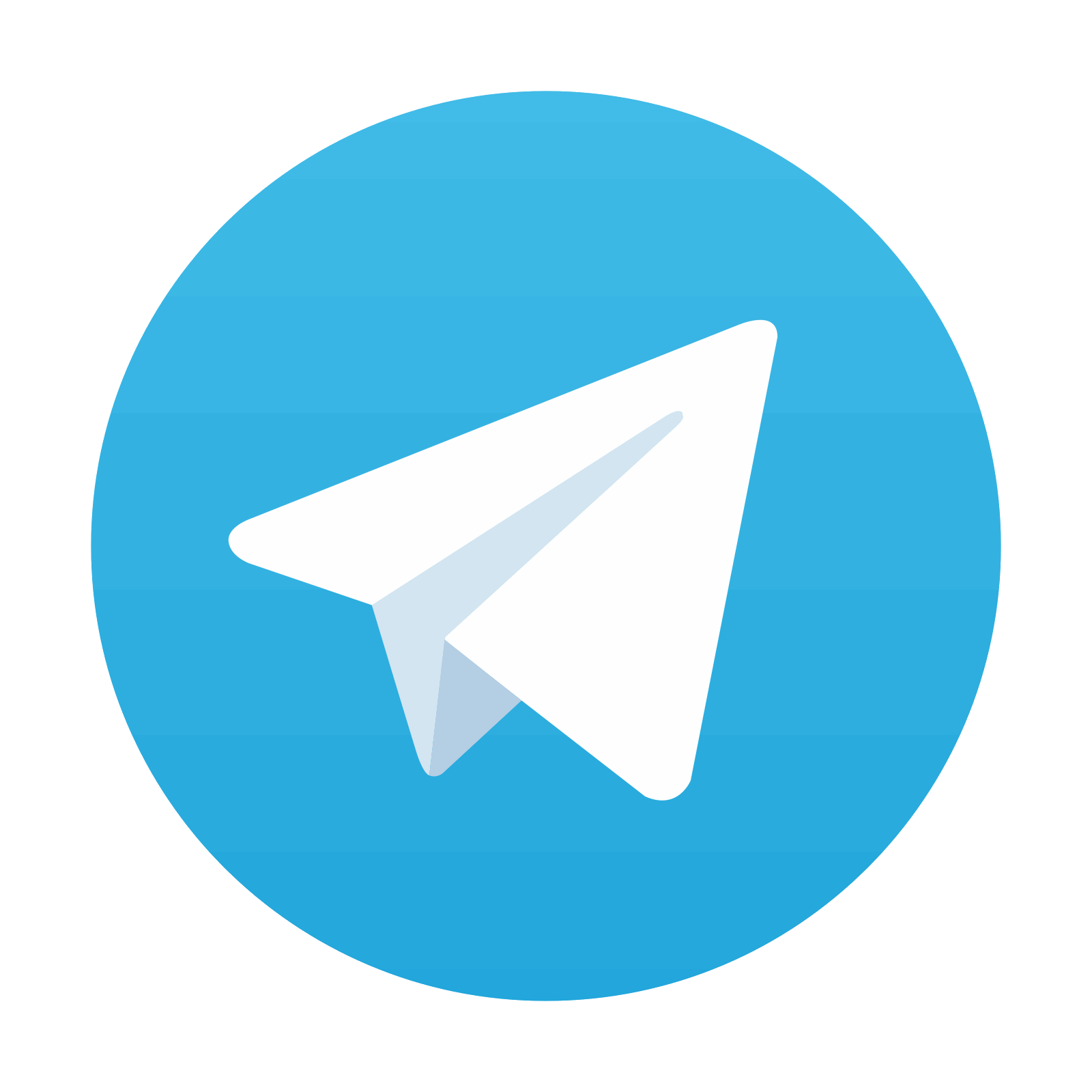
Stay updated, free dental videos. Join our Telegram channel

VIDEdental - Online dental courses
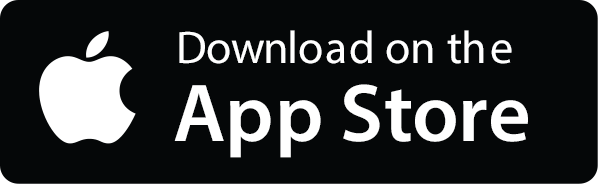
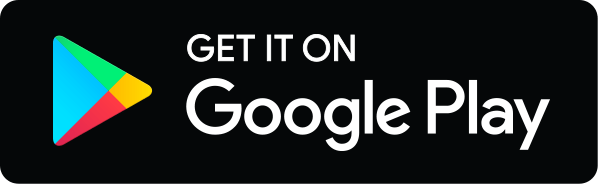