Abstract
Objectives
Light cured materials are increasingly used in orthodontic clinical practice and concurrent with developments in materials have been developments in light curing unit technology. In recent years the irradiances of these units have increased. The aim of this study was to determine the safe exposure times to both direct and reflected light.
Methods
The weighted irradiance and safe exposure times of 11 dental curing lights (1 plasma arc, 2 halogen and 8 LED lights) were determined at 6 distances (2–60 cm) from the light guide tip using a spectroradiometer. In addition, using the single most powerful light, the same two parameters were determined for reflected light. This was done at a distance of 10 cm from the reflected light, but during simulated bonding of 8 different orthodontic brackets of three material types, namely stainless steel, ceramic and composite.
Results
The results indicate that the LED Fusion lamp had the highest weighted irradiance and the shortest safe exposure time. With this light the maximum safe exposure time without additional eye protection for the patient (at 10 cm), the operator (at 30 cm) and the assistant (at 60 cm) ranged from 2.5 min, 22.1 min and 88.8 min respectively. This indicates a relatively low short term risk during normal operation of dental curing lights. For reflected light at a distance of 10 cm the risk was even lower, but was affected by the material and shape of the orthodontic bracket under test.
Significance
The short term risks associated with the use of dental curing lights, halogen, LED or plasma, appear to be low, particularly if as is the case adequate safety precautions are employed. The same is true for reflected light from orthodontic brackets during bonding. What is still unclear is the potential long term ocular effects of prolonged exposure to the blue light generated from dental curing lights.
1
Introduction
The use of light curing as a means of initiating the polymerization of orthodontic adhesives has gained in popularity in recent years, having first been described by Tavas and Watts . Indeed since its introduction over 30 years ago there has been a progressive shift toward the use of light cured materials in dentistry and an increase in the number and types of light curing units available. Light cured materials have a number of advantages over chemically cured adhesives, including single paste application, consistent handling characteristics, easy removal of excess material, extended working time, command set, more accurate bracket positioning and immediate ligation of the archwire .
There are currently three main types of light curing units available, namely: halogen, plasma arc and light emitting diodes (LED). With time the lights available have become ever more powerful, producing higher light intensities (mW/cm 2 ) with obvious advantages and potential disadvantages. The advantages extend to both the operator and the patient and include shorter curing times , saving time and money, less chance of bracket drift, a reduced risk of moisture contamination and overall less discomfort for the patient . However, what is uncertain is whether or not these new more powerful lights also bring with them additional risks to the eyes of the operator, ancillary staff or patient.
In order to cure dental composites and resin modified glass ionomer cements, light with a wavelength of 440–480 nm is required to initiate free radical addition polymerization. This is within the spectrum of blue light. However, some adhesives contain more than one photo initiator and these may have slightly different peak sensitivity wavelengths, down to as low as 370 nm , which is within the UV spectrum. In addition to light of a specific wavelength, irradiance is also important in the cure of resin based dental composites. It has been suggested that a minimum irradiance (power per unit area) of 300 mW/cm 2 is required to produce an adequate degree of polymerization . While doubling the output above this minimum increases the rate of cure by up to 22% , increasing it beyond 1000 mW/cm 2 is said to have no further detectable benefit to the polymerization of resin composites . Nevertheless some of the newer light curing units has been reported to have power outputs of up to 2000 mW/cm 2 .
As a part of normal vision, when light reaches the photoreceptors of the eye, photochemical reactions take place creating electrical neural impulses. As a by product of this process, harmful reactive oxygen species (ROS) are also produced within the retina and in this respect blue light is potentially 100 times more damaging than orange light (590 nm) . However, this oxidative damage is usually prevented by macular pigments based on carotenoids, which work by increasing oxygenation at the arterial end of the retina . With age there is an increased risk of a condition known as Dry Age Related Macular Degeneration, in which there is a gradual deterioration of central vision due to a loss the photoreceptors and their supporting tissue in the macula. Although it is thought to be a multi-factorial disease, one possible cause is thought to be prolonged exposure to blue light .
What then are the exposure risks with the normal use of dental curing lights? The maximum permissible daily exposure times for intense blue light sources can be calculated using criteria published by the American Conference of Governmental Industrial Hygienists (ACGIH) . In the exposure standard, two viewing conditions are described: point source conditions apply when the source is far removed from the eye (greater than 1 m) and extended source conditions when the source is close to the eye (less than 1 m). In normal dental use, extended source conditions apply. For exposure times less than 2.8 h (10 4 s) the limit is given by the formula L B × t ≤ 100 where L B is the radiance of the source weighted by the blue light hazard function and t is the cumulated daily exposure time in seconds. The blue light hazard function at different wavelengths can be obtained from published data . Very recently new guidelines for the calculation of the maximum daily exposures have been produced by the Health and Safety Executive in the UK which has increased the maximal permissible exposure .
To date, a number of studies have described the potential for retinal damage from dental light curing units. Satrom et al. found a variation in the maximum daily exposure photochemical (blue light hazard) to the retina, with different lights ranged from 2.4 min a day up to 16 min a day. Whereas Moseley et al. found the maximum permissible daily exposure ranged from 12 min to 30 min for direct light and between 40 and 100 min for reflected light. More recently Roll et al. estimated the maximal permissible exposure times of 13 dental curing lamps using data on spectral output and irradiance. In their calculations, performed according to the exposure limit guidelines set by the American Conference of Governmental and Industrial Hygienists and the International Commission on Non-Ionising Radiation Protection , they assumed a 30% reflection of the curing light radiation and at a distance of 30 cm (the distance between the dental operation site and the operator’s eye). They estimated the blue light maximum for the eye to be approximately 1 min/day for reflected light, whereas a direct (accidental) blue-light exposure with zero distance from the eye should not exceed 1 s. This maximum was also true for the UV component of halogen lamps. It should be remembered however that these studies were carried out in the mid 1980s when many of the more modern higher irradiance curing lamps were not available.
The aims of the present study were therefore to calculate the maximum permissible daily exposure times for a number of currently available light curing units and to evaluate the effect of reflected light using different orthodontic brackets.
2
Materials and methods
The maximum permissible daily exposure times and blue light hazards of 11 curing lights (1 plasma arc, 2 halogen and 8 LED lights) ( Table 1 ) were determined by measuring their spectral outputs using an integrated spectroradiometer (DMc150-MDE, Bentham Instruments Ltd, UK) ( Fig. 1 ). The lights under investigation, along with their wavelengths (nm) and irradiances (mW/cm 2 or power per unit area) derived from the manufacturer’s data, are illustrated in Table 1 . The spectroradiometer was configured to measure source irradiance over the spectral range of 400 nm and 550 nm and at 7 predetermined distances from the light curing tip. These distances were 2 cm, 10 cm, 20 cm, 30 cm, 40 cm, 50 cm and 60 cm and were chosen in order to simulate direct exposure at 2 cm, through to a distance of 30 cm representing the light tip to eye distance of the orthodontist and finally up to 60 cm, which represents the light tip to eye distance of the assistant during normal bonding. The 2 cm distance was chosen as it was the minimum distance at which the spectroradiometer could operate, without saturation due to the very high irradiances from certain light curing unit models. A specially constructed jig was used to ensure the predetermined distances were the same for each light curing unit relative to the fixed detector head of the spectroradiometer.
Light unit | Type | Wavelength (nm) | Irradiance mW/cm 2 |
---|---|---|---|
Apollo 95 E | Plasma arc | 460–490 | 1600 |
Cromalux | Halogen | 400–500 | 650–800 |
CU80 | Halogen | 380–510 | 600 |
DEMIOrtho | LED | 420–465 | 1100–1330 |
Elipar Freelight 2 | LED | 430–480 | 1000 |
Fusion | LED | 385–430 | 1500 |
Mini LED | LED | 420–480 | 1250 |
Mini LED2 | LED | 420–480 | 2000 |
Ortholux | LED | 430–480 | 1600 |
Smartlite | LED | 450–490 | 950 |
Starlight | LED | 440–480 | 100 |
The spectroradiometer itself consists of a double monochromator based on two 150 mm focal length single monochromators in the Czerny Turner configuration, and integrated DC detection electronics. Light is coupled from the detector, which includes a cosine diffuser (D7H-WT), to the monochromator via a 1 m randomized quartz optical fiber bundle. The cosine response of this diffuser was calibrated with a less than 3% f2 error. The system was controlled using Bentham proprietary software (Benwin + version 3.0.10.0) and the spectroradiometer produced plots of irradiance (w cm −2 nm −1 ) against wavelength (nm), as well as calculating the weighted irradiance using the blue light hazard function.
The spectroradiometer was calibrated by a dedicated CL6 light source with calibration traceable to the National Physics Laboratory (NPL) standard. The CL6 light source is powered by a constant current source at 8.500 A with a tungsten halogen lamp, providing a very stable output irradiance. The spectroradiometer was calibrated before each measurement session to eliminate any inaccuracy within the system. The uncertainty within the system after calibration is less than ±1.5%.
Irradiance measurement for each type of curing light unit began with a scan covering the wavelengths between 250 nm and 800 nm in order to identify the output spectrum. Subsequence measurements were made only at the relevant wavelengths. The combined tolerance of the measurement was typically less than 5%, including temporal variation due to continuous battery discharge condition. Care was taken to ensure each of the lights was tested with a fully charged battery and a new bulb was placed in each of the quartz tungsten halogen lights prior to testing. The ambient light was minimized during testing by closing the blinds and turning off indoor fluorescent lighting. In the second part of this study, determining the effect of different brackets and bracket materials (metal and esthetic – see Table 2 ) on reflected light and therefore the maximum daily exposure limits, a phantom head unit with acrylic teeth was used, as it was felt these were less variable than using extracted human incisor teeth. All the measurements for reflected light were performed with the light curing unit that had been found to produce the greatest spectral output in the first part of the experiment, namely the Fusion LED curing light. The spectroradiometer tip was placed at a distance of 10 cm from the brackets and was held in place using the custom made jig ( Fig. 1 ). The brackets were placed on upper canine to canine with Transbond XT (3 M) adhesive and the light tip held at a distance of 0.5 cm from the central incisor teeth.
Bracket type | Trade name | Manufacturer |
---|---|---|
Ceramic | Clarity | 3M Unitek |
Ceramic | Clarity SL | 3M Unitek |
Stainless steel | Victory | 3M Unitek |
Stainless steel | Microarch | GAC International |
Ceramic | Encore | Ortho Technology |
Composite | Tiger | TOC |
Stainless steel | Mini Ovation | GAC International |
Stainless steel | TOC bracket | TOC |
2
Materials and methods
The maximum permissible daily exposure times and blue light hazards of 11 curing lights (1 plasma arc, 2 halogen and 8 LED lights) ( Table 1 ) were determined by measuring their spectral outputs using an integrated spectroradiometer (DMc150-MDE, Bentham Instruments Ltd, UK) ( Fig. 1 ). The lights under investigation, along with their wavelengths (nm) and irradiances (mW/cm 2 or power per unit area) derived from the manufacturer’s data, are illustrated in Table 1 . The spectroradiometer was configured to measure source irradiance over the spectral range of 400 nm and 550 nm and at 7 predetermined distances from the light curing tip. These distances were 2 cm, 10 cm, 20 cm, 30 cm, 40 cm, 50 cm and 60 cm and were chosen in order to simulate direct exposure at 2 cm, through to a distance of 30 cm representing the light tip to eye distance of the orthodontist and finally up to 60 cm, which represents the light tip to eye distance of the assistant during normal bonding. The 2 cm distance was chosen as it was the minimum distance at which the spectroradiometer could operate, without saturation due to the very high irradiances from certain light curing unit models. A specially constructed jig was used to ensure the predetermined distances were the same for each light curing unit relative to the fixed detector head of the spectroradiometer.
Light unit | Type | Wavelength (nm) | Irradiance mW/cm 2 |
---|---|---|---|
Apollo 95 E | Plasma arc | 460–490 | 1600 |
Cromalux | Halogen | 400–500 | 650–800 |
CU80 | Halogen | 380–510 | 600 |
DEMIOrtho | LED | 420–465 | 1100–1330 |
Elipar Freelight 2 | LED | 430–480 | 1000 |
Fusion | LED | 385–430 | 1500 |
Mini LED | LED | 420–480 | 1250 |
Mini LED2 | LED | 420–480 | 2000 |
Ortholux | LED | 430–480 | 1600 |
Smartlite | LED | 450–490 | 950 |
Starlight | LED | 440–480 | 100 |
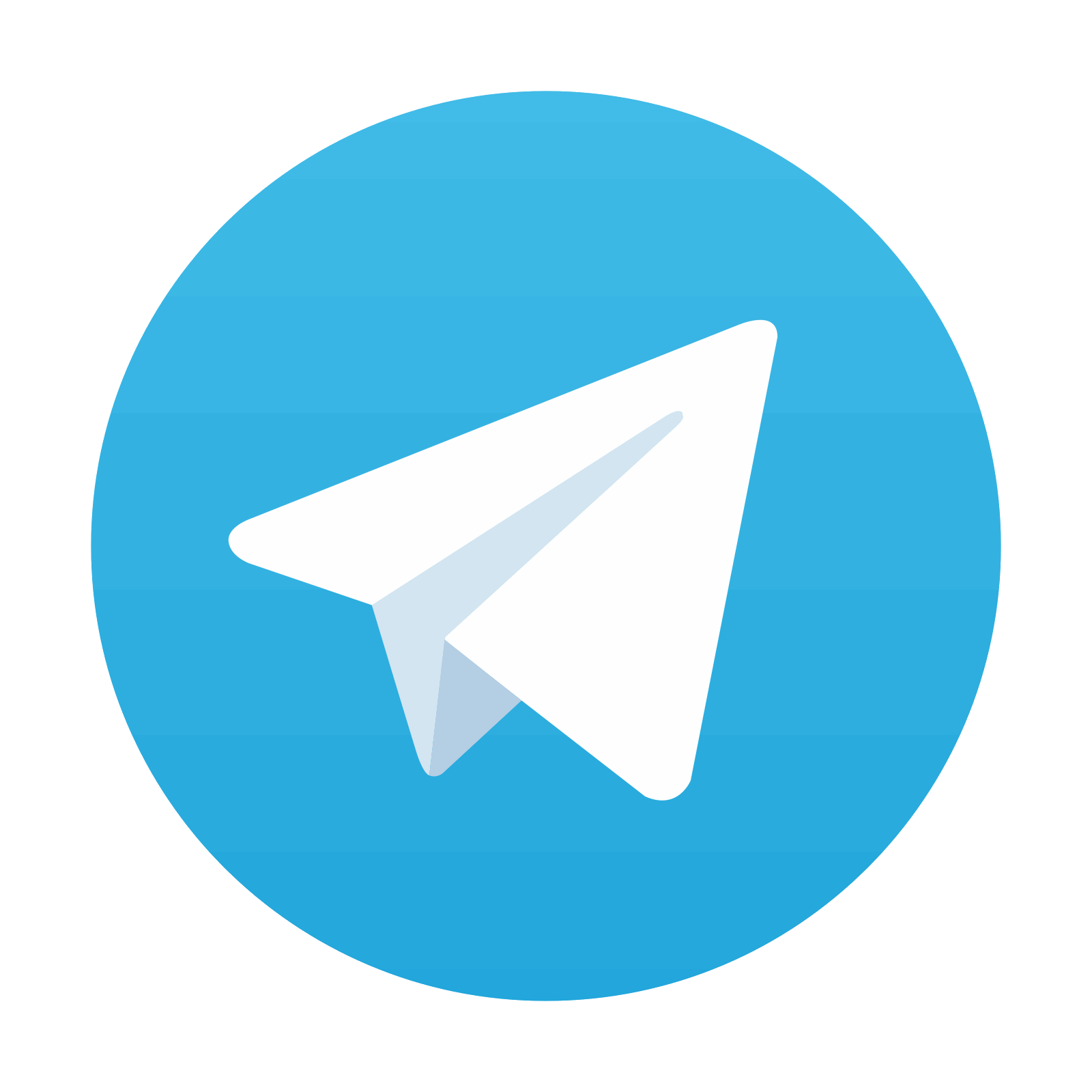
Stay updated, free dental videos. Join our Telegram channel

VIDEdental - Online dental courses
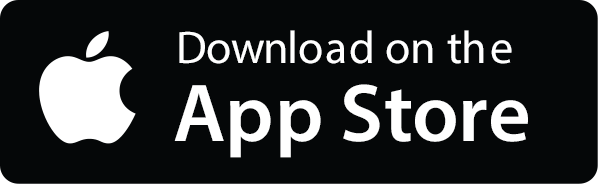
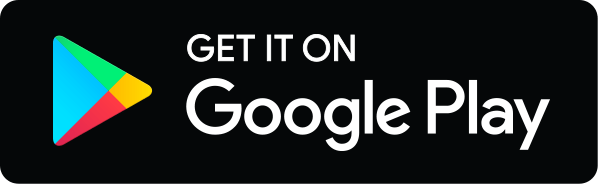
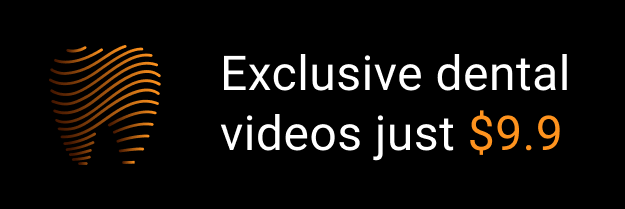