13
Lasers in Surgical Therapy of Peri‐implantitis
Nathan E. Estrin1, Akira Aoki2, Anton Sculean3, Richard J. Miron3, and Georgios E. Romanos4
1 Lake Erie College of Osteopathic Medicine School of Dental Medicine, Bradenton, FL, USA
2 Department of Periodontology, Tokyo Medical and Dental University, Tokyo, Japan
3 Department of Periodontology, University of Bern, Bern, Switzerland
4 Department of Periodontics and Endodontics, School of Dental Medicine, Stony Brook University, Stony Brook, NY, USA
Introduction
Similar to periodontitis, surgical therapy of peri‐implantitis includes both regenerative and resective approaches [1]. During resective surgery of peri‐implantitis, the goal is to remove granulation tissue, eliminate osseous cratering, and apically position the tissues. This is often done in combination with implantoplasty in which the goal is to smooth the implant threads and rough surface of the implant to provide a smoother surface unfavorable to biofilm formation [1]. However, implantoplasty is controversial in the amount of titanium particles expelled into the surrounding tissue during the process [2, 3]. Surgical therapy also involves decontaminating the implant surface and applying biological materials, such as bone grafts, membranes, and growth factors in an attempt to regenerate the lost implant–supporting structures [4].
Whether the treatment is non‐surgical therapy, or surgical therapy, the goal in treating peri‐implantitis is to disinfect the implant surface [5]. Even in resection of the peri‐implant tissues, implantoplasty is often done in order to remove the contaminated titanium oxide layer and threads of the implant and provide a smoother surface less favorable for biofilm formation [4], while managing periodontitis on the other hand, teeth are debrided with curettes removing a superficial layer of cementum and bacterial load in the periodontal pocket prior to periodontal repair. However, curettes have shown to be ineffective against implant surfaces [5]. Additionally, utilizing curettes against implant surfaces has been shown to create titanium particles in the surrounding tissues, potentially contributing to the inflammatory response [3]. Therefore, alternative methods are pursued in decontaminating the implant surfaces including chemotherapeutics, such as antiseptics and antibiotics, as well as laser irradiation and photodynamic therapy [6]. Laser irradiation allows for the decontamination of the implant providing a useful adjunct in the management of peri‐implantitis [7].
The term “laser” is an acronym for “Light Amplification for Stimulated Emission of Radiation” [8, 9]. Laser light differs from standard light in that it is monochromatic, coherent, and collimated [8, 10]. Each laser system has an active medium that directly contributes to the monochromatic wavelength produced. Knowledge of the various wavelengths is critical as this directly relates to the type of laser‐tissue interaction utilized [8, 10]). Specifically in medicine, there are four different types of interactions with tissues [8, 9]. These include absorption, reflection, transmission, and scattering of the laser light [8, 9]. While absorption is the most desired outcome, the type of laser‐tissue interactions depends on both the laser wavelength and the characteristics of the tissue [8, 9]. Depending on the laser wavelength, there are different effects in both hard and soft tissues as well as titanium surfaces [7, 8, 9]. Given that different wavelengths exhibit different laser‐tissue interactions depending on the targeted chromophore, each wavelength will exhibit different interactions with the titanium surface. Lasers in the treatment of peri‐implantitis in general lacks significant evidence due to the difficulties of conducting randomized controlled clinical trials in a split‐mouth fashion. Therefore, more publications are needed from basic in‐vitro studies, animal studies, and various clinical case series and randomized clinical trials. The purpose of this chapter was to review the characteristics of various laser wavelengths available in dentistry and discuss their effects on current evidence on interactions with titanium implant surfaces and treatment in peri‐implantitis.
Diode Lasers for Peri‐implantitis Therapy
Diode lasers are classified by having a semi‐conductor as their active medium with resulting wavelengths in the infrared and near‐infrared spectrum. While the most commonly utilized laser wavelengths in dentistry range from 810 to 980 nm, a new 445 nm blue light has shown promising results in wound healing and warrants further investigation [11, 12] (Figure 13.1).
Given that diode lasers have a relatively high absorption coefficient for melanin and hemoglobin, near‐infrared diode lasers are advantageous when utilized for coagulation and soft tissue surgery [10]. Diode lasers are commonly used in clinical settings due to the relatively low cost and smaller size when compared to other wavelengths such as the Nd:YAG and Er:YAG [13, 14]. The delivery method for diode lasers is fiberoptic tips which provide versatility to its use [15, 16] (Figure 13.2). Initiators such as articulating paper or a cork provide a dark pigmented mass at the end of the fiberoptic tip which focuses the laser beam. This provides concentrated maximum energy on the targeted tissue, creating a “hot tip” for precise cutting of the soft tissues [15, 16]. Diode lasers are very effective in cutting soft tissue precisely and are known for stalling and dragging during the cutting process if not reinitiated [14]. However, this is an advantage of diode lasers as the tissue can be “felt” while cutting unlike the non‐contact devices, such as CO2 and erbium lasers [14]. In contrast, non‐initiated tips can be used in a non‐contact modality to provide a defocused beam aiding in coagulation or for photobiomodulation [10]. To further increase its versatility, diode lasers are commonly utilized during photodynamic therapy where a photosensitizer is activated by the laser light to carry out a desired photochemical effect. When evaluating the potential and use of diode lasers in peri‐implantitis, it is important to consider the method utilized and the combination of initiated, versus non‐initiated tip in addition to the wavelength. Additionally laser safety protocols to control overheating and risks from laser irradiation.

Figure 13.1 New developed diode laser with visible blue light (445 nm) and near‐infrared 970 nm laser wavelength (Sirona, Bensheim, Germany).
Effects of Diode Lasers on Titanium Surfaces
Previous studies on laser‐irradiated titanium discs with either Nd:YAG and 980 nm diode lasers showed that the 980 nm diode laser displayed no surface damage even at 15 W compared to the use of pulsed Nd:YAG laser which melted the titanium surface and created cracks and other surface damages [17]. This is consistent with the study by Kreisler et al. in which the Nd:YAG, Ho:YAG, Er:YAG, CO2, and GaAlAs (diode) lasers were compared on various implant surfaces. Sandblasted, acid‐etched, plasma‐sprayed, hydroxyapatite‐coated, and smooth titanium disks were irradiated with different laser groups and analyzed via SEM and energy dispersive spectroscopy [18]. Consistent with the previous studies mentioned, the Nd:YAG laser‐induced melting, cracking, and crater formation on all implant surfaces. The Ho:YAG laser also produced these surface alterations on all tested surfaces. The Er:YAG and the CO2 laser caused surface alterations only when utilized at higher power output, specifically with superpulsed mode for the CO2 laser. The GaAlAs (809 nm) appeared to be the safest laser and did not induce any damage on any of the titanium disks [18]. Another in‐vitro study analyzed the thermal changes on titanium surfaces after irradiation with diode, Er:YAG, and CO2 lasers [19]. The diode laser demonstrated clinically unacceptable temperature changes for both the 810 and 980 nm when utilized in continuous mode [19]. Therefore, carefully selected parameters are required when utilizing diode lasers on implant surfaces. Similarly, in‐vitro studies assessed the thermal effect of the 445 nm diode laser on five dental implant systems. The studies showed that laser irradiation systems can prevent harmful rises in temperature and surface alteration when used at moderate laser parameters [20]. It was shown that the 445 nm wavelength caused temperature increases of more than 10 °C at or above the 0.8 W power level working in continuous wave mode for 5 seconds and in pulsed mode at 3 W for 20 seconds with 10% duty cycle. The highest rises in temperature were seen in the Straumann Pure ceramic implant, lowest temperature observed in the Ankylos system.

Figure 13.2 Irradiation of an implant for decontamination of the implant surface (810 nm diode, Ivoclar, Amherst, NY).
Clinical Studies
In a clinical, split‐mouth trial involving 10 patients with 48 implants, a diode laser (810 nm) as an adjunct to traditional scaling was evaluated. However, the trial concluded that the diode lasers did not yield any positive influence on peri‐implantitis when used in conjunction with mechanical therapy [21]. In another randomized clinical trial, 27 patients requiring treatment for peri‐implantitis were treated with either conventional non‐surgical therapy alone or in combination with the 810 nm diode laser [22]. With a total of 125 implants (750 sites) included, the authors found a statistically significant reduction in PPD and BOP in the treatment group compared to the control group. However, the study only had a follow‐up of six months. Both of these studies were included in a systematic review evaluating the management of peri‐implantitis with the diode laser in non‐surgical therapy [23]. All three studies included utilized the 810 nm wavelength. While the two studies discussed above showed statistical significance with the diode as an adjunctive therapy, the randomized clinical trial by Bach et al. [24] failed to report a statistical significance between the two groups after a five‐year follow‐up [24]. Due to the high heterogeneity of the studies, no meta‐analysis was completed. Therefore, the results of the systematic review were that additional studies are needed, and the evidence thus far does not support the adjunctive use of diode lasers in non‐surgical peri‐implantitis therapy.
In regard to surgical therapy, a randomized clinical trial evaluated the efficacy of utilizing a diode laser as an adjunct in conventional open‐flap debridement of failing implants. With 19 patients evaluated, both groups showed significant improvement in periodontal parameters after six months of treatment. However, the group irradiated with the diode laser only provided marginal improvement in CAL, while no difference between test and control groups was noted for PD and BOP [25]. However, more studies are necessary with the 810 nm and other diode wavelengths in the surgical management of peri‐implantitis.
Summary of Diode Lasers in Peri‐implant Therapy
While there is a lack of clinical trials on the use of diode lasers in peri‐implantitis therapy, in‐vitro results are promising. Studies by Kreisler et al. and Romanos et al. displayed no surface alterations to the titanium disks with an 810 and 980 nm diode laser when compared to the Nd:YAG laser which produced irreversible changes [17, 18]. While the adjunctive use of surgical therapy with the 810 nm diode laser showed promising results by Papadopoulos et al. [25], additional studies are necessary. Additionally, a newer diode wavelength of a visible blue light with a 445 nm wavelength has been suggested to have improved hemostatic effects and wound healing potential [12, 26]. However, this wavelength has not yet been investigated clinically in peri‐implantitis treatment. Given that the goal of laser irradiation in peri‐implant and periodontal protocols is to produce a blood coagulum in the base of the pocket to promote regeneration, the blue light might be a useful tool in peri‐implantitis. However, more research with this wavelength is necessary, especially in in‐vivo applications. While there is a general lack of study on diode lasers in peri‐implantitis therapy, it is important for future studies to evaluate both initiated and non‐initiated tips as well as the variable wavelengths available in the diode family.
Nd:YAG Lasers in Peri‐implantitis Therapy
Nd:YAG lasers have a solid‐state active medium in which about 1% of the yttrium is replaced with neodymium. The resulting wavelength is 1064 nm therefore having a deep penetration depth in soft tissues [7, 9] a strong coagulation affect [5, 10] and has also been successful in decontaminating the periodontal pockets [27]. While its first use in dentistry was for the removal of caries [28] the Nd:YAG laser is primarily utilized for periodontal therapy and soft tissue excision [7]. The Nd:YAG laser is most commonly known today by its trademarked technique: Laser‐Assisted New Attachment Procedure (LANAP®), which includes a specific protocol for periodontal regenerative therapy that has been verified with histological evidence [29, 30]. Although it is one of the most commonly utilized lasers in periodontal therapy, it has the deepest penetration depth in soft tissue [7] and due to a high risk of overheating (Figure 13.3), this laser is only utilized in dentistry in a pulsed setting.
While it’s success in periodontal therapy is well documented, the Nd:YAG has actually shown to have detrimental effects when utilized on implant surfaces. Block et al. [31] reported irreversible changes including melting, loss of porosity, cracking, and ruptures on the surfaces of the HA‐coated implant in an in‐vitro setting [31]. Alarmingly, this occurred at all power settings tested. In a different study by Romanos et al. [17], three different titanium discs which were either hydroxyapatite (HA)‐coated, titanium plasma sprayed (TPS), or sandblasted were irradiated with an Nd:YAG or 980 nm diode laser [17]. Scanning electron microscopy displayed extensive melting in all Nd:YAG laser irradiated areas. All disks that were TPS and HA‐coated displayed damage from the dental pulsed Nd:YAG laser, even at the lowest power setting of 2.0 W [17].
Summary of Nd:YAG Lasers in Peri‐implant Therapy
In summary, the undesirable outcomes with the Nd:YAG laser on titanium implants are well documented [17, 18, 32, 33. Only when utilized in a soft setting the Nd:YAG achieved desirable results [34]. Therefore, it is becoming increasingly understood by clinicians that utilizing the Nd:YAG laser on implant surfaces should be avoided.
Erbium Lasers in Peri‐implantitis Therapy
The pulsed Er:YAG laser (2940 nm) has the dual ability to effectively ablate both soft and hard biological tissues with minimal thermal side‐effects, due to its high absorption by water (Figure 13.4) [35, 36]. The Er,Cr:YSGG laser (2780 nm), another laser type from the erbium laser family, also shows performance similar to the Er:YAG, with a difference being that the Er,Cr:YSGG laser’s energy is absorbed more by hydroxyl (OH−) ions than by water molecules [37]. Thus, erbium lasers can be applied not only for oral soft tissue surgery but also for hard tissue treatments such as caries removal, root surface debridement, bone ablation, as well as implant surface debridement [38]. Currently, erbium laser systems have the broadest range of periodontal and peri‐implant applications (Figure 13.5) [7, 38, 39].

Figure 13.3 Titanium surface showing melting after Nd:YAG laser irradiation.
Tissue Ablation and Wound Healing Following Erbium Laser Irradiation
Erbium lasers evaporate soft tissues via photo‐thermal effects, delivering precise ablation with the advantage (among available dental lasers) of minimal thermal effects [40–42], particularly when used in combination with a water spray [38, 40]. Between the Er:YAG and Er,Cr:YSGG lasers, the effect of soft tissue ablation is reportedly greater for Er:YAG, with less thermal side‐effects, compared to Er,Cr:YSGG [40, 43]. The width of the coagulation layer on porcine gingival tissue following erbium laser ablation is minimal: approximately 18 μm with water spray for Er:YAG and 33 μm for Er,Cr:YSGG with water spray [40]. Compared to electrosurgery, Er:YAG laser surgery shows much less thermal change in gingiva as well as faster and more favorable gingival wound healing [44]. These characteristics make the erbium laser a suitable choice for peri‐implant soft tissue management [7]. Erbium lasers can also ablate hard tissues such as calculus, root cementum and dentin, and bone without major thermal damage [38, 39, 45]. This is accomplished through “thermo‐mechanical” effects based on the production of “micro‐explosions” of water molecules within the hard tissues [38, 39, 46, 47].

Figure 13.4 Laser absorption spectrum for water. Data were calculated from Hale and Querry [35]. Ar (488 nm), Diode (810 nm), Nd:YAG (1064 nm), Er,Cr:YSGG (2780 nm), Er:YAG (2940 nm), and CO2 (10,600 nm) are indicated in the figure.

Figure 13.5 Erbium Laser apparatuses and associated handpieces. (a) Er:YAG, Erwin AdvErLTM Evo, J. Morita Mfg. Corp., Kyoto, Japan (b) Er:YAG, LightTouch, Light Instruments Ltd., Yoknea, Israel (c) Er:YAG, LightWalker AT S NE, Fotona, d.o.o., Ljubljana, Slovenia (d) Er,Cr:YSGG, Waterlase iPlus, BIOLASE, Inc., California, USA.
In addition, lasers possess the interesting and characteristic property of bio‐stimulation effects (photobiomodulation: PBM) induced by low power laser energy [48, 49]. Briefly, during soft tissue surgery with high power laser irradiation, a certain amount of low energy simultaneously scatters or penetrates into the surrounding or underlying tissues, stimulating biological response and promoting wound healing/regeneration [50]. The Er:YAG laser, also capable of PBM effects, induces promotion of cell proliferation [51–54] and enhances calcification on osteogenic cells [55–57].
Effects of Erbium Lasers on Titanium Surfaces
Erbium lasers cause no visible changes to the titanium surface under appropriate irradiation conditions, although irradiation at high energy levels produces distinct surface changes on titanium [58–64] (Figure 13.6). The use of water spray minimizes temperature elevations of titanium during Er:YAG laser irradiation [58, 65]. The Er,Cr:YSGG laser can also be applied safely and effectively on the titanium surface in a manner similar to that with the Er:YAG laser [62, 66, 67].
The erbium lasers are capable of effectively removing calcified deposits and plaque on micro structured surfaces [64,67–70], when compared to conventional mechanical methods such as ultrasonics, plastic or titanium curettes, or cotton pellets [71–73] (Figure 13.7). The Er:YAG laser possesses a high bactericidal potential on implants with different surface characteristics, even at low energy densities [74]. Erbium laser decontaminated surfaces recover their biocompatibility, showing the attachment of osteoblasts or fibroblasts [61, 67, 75, 76]. Thus, both Er:YAG laser and Er,Cr:YSGG laser irradiation are effective methods for decontamination without surface alterations under suitable irradiation conditions [7, 77].
The erbium lasers can preserve most of the microstructured surfaces under suitable irradiation conditions, but the anodic oxidized surface cannot be preserved by the Er:YAG laser [59, 64, 78]. With the anodized microstructure, instead of being preserved, complete peeling off the oxidized layer followed by exposure of a fresh rough titanium surface has been proposed [79, 80]. Thus, erbium lasers generally show effective and safe application for the debridement of microstructured titanium surfaces except for anodized surfaces. Nonetheless, when erbium lasers are used on titanium surfaces, the operator always needs to be mindful of the irradiation parameters and conditions so as not to induce the concentration or accumulation of heat during irradiation [7, 62, 64].

Figure 13.6 Stereomicroscopy and scanning electron microscopy (SEM) images of commercially available micro‐structured titanium implants after Er:YAG laser irradiation. Er:YAG laser spot irradiation was performed at 30, 40, and 50 mJ/pulse (energy density: 10.6, 14.2, and 17.7 J/cm2/pulse) and 30 Hz in near contact mode for five seconds. For the SLA® surface, 30 and 40 mJ/pulse with water spray showed no changes in stereomicroscopy observations, but 40 mJ/pulse produced a slight change. In SEM images, 40 and 50 mJ/pulse with water spray showed slight melting. Without water, 50 mJ/pulse produced severe thermal changes. For the TiUnite® surface, the anodized microstructure was always destructed, resulting in exposure of a fresh rough titanium surface (arrowheads) below the original microstructure. Arrows indicate color change, melting, cracking, or resolidification of the micro structured surface. Control: non‐irradiated control; Water: water spray.
(Source: Lasers in Medical Science © 2013 / with permission from Springer Nature).

Figure 13.7 Stereomicroscopy images of contaminated microstructured surface of an explanted implant after Er:YAG laser irradiation and ultrasonic scaling (US) with a plastic tip. Before treatment, calcified deposits (arrowheads) were evident on the microstructured surface (a). Er:YAG laser irradiation was performed at 20 and 30 mJ/pulse (energy density: 7.1 and 10.6 J/cm2/pulse) and 30 Hz under water spray in near contact mode. Calcified deposits were effectively removed without visible damage to the microstructured surface in all treated areas (b). Thin white‐gray fragments (possibly plastic debris) were observed throughout the US‐treated area (b).
(Source: Lasers in Medical Science © 2013 / with permission from Springer Nature).
In‐vivo Studies of Erbium Lasers in Peri‐implantitis Therapy
Erbium lasers, different from other high‐power lasers, can be advantageously applied not only for fixture debridement but also for degranulation in the peri‐implant bone defect [60,81–83].
In animal studies on surgical therapy of peri‐implantitis, Schwarz et al. [84] reported that the application of Er:YAG laser irradiation during flap surgery seemed to promote re‐osseointegration on contaminated implant surfaces more than plastic curettes plus metronidazole gel and ultrasonic devices in a circumferential crater‐like bone defect. Takasaki et al. [85] also demonstrated that the Er:YAG laser debridement of bone defects as well as implant surfaces tended to produce greater bone‐implant contact than curette treatment in flap surgery for experimentally‐induced peri‐implant infection in dehiscence‐type defects in dogs (Figure 13.8). Nevins et al. [80] observed in dog experiments that Er:YAG laser decontamination of implant surfaces allowed for regeneration and improved bone‐to‐implant contact.
Clinical Studies
Recently, erbium lasers have been increasingly applied non‐surgically and surgically in peri‐implantitis treatment [7, 86, 87].
Non‐surgical Therapy
In non‐surgical applications, Schwarz et al. [72, 88] reported that Er:YAG laser treatment led to significant clinical improvements six months after therapy with a significant higher reduction of BOP compared to mechanical debridement using plastic curettes and antiseptic therapy, but its effectiveness seemed to be limited to a period of six months and may not be sufficient for the maintenance of failing implants in the long term due to a remaining mixed chronic inflammatory cell infiltrate in the connective tissue stroma [89]. Also, Renvert et al. [1] and Persson et al. [90] reported that clinical improvements following the non‐surgical treatment of peri‐implantitis lesions using an Er:YAG laser was limited at six months.
From systematic reviews, although Muthukuru et al. in 2012 [91] and Yan et al. in 2015 [92] reported that Er:YAG laser non‐surgical treatment could potentially provide short‐time additional benefits in comparison to conventional treatment, Kotsakis et al. in 2014 [93] and Mailoa et al. [94] could not identify any superiority of laser treatment.
Thus, based on the current evidence of short‐term benefits of laser use in peri‐implant disease, non‐surgical laser therapy might be useful as initial treatment for controlling acute inflammation before surgical therapy [86]. Non‐surgical therapy seems to not always be sufficient for complete healing of peri‐implantitis sites [88], which indicates that depending on the case, a surgical approach may be necessary for the treatment of peri‐implant lesions in order to achieve favorable clinical healing.

Figure 13.8 Surgical treatment of experimentally induced dehiscence‐type peri‐implantitis lesions in dogs. At the time of access for therapeutic surgery, all defects were filled with granulation tissue (a and b). Degranulation and implant surface decontamination were performed by either an Er:YAG laser device (c), or plastic curets (d). At four months following therapy, favorable formation of new bone was observed in the laser group (e) when compared to the control group (f). Villanueva golder stain, bar = 500 μm, BBD, bottom of the bone defect; CBD, coronal level of bone defect.
(Source:
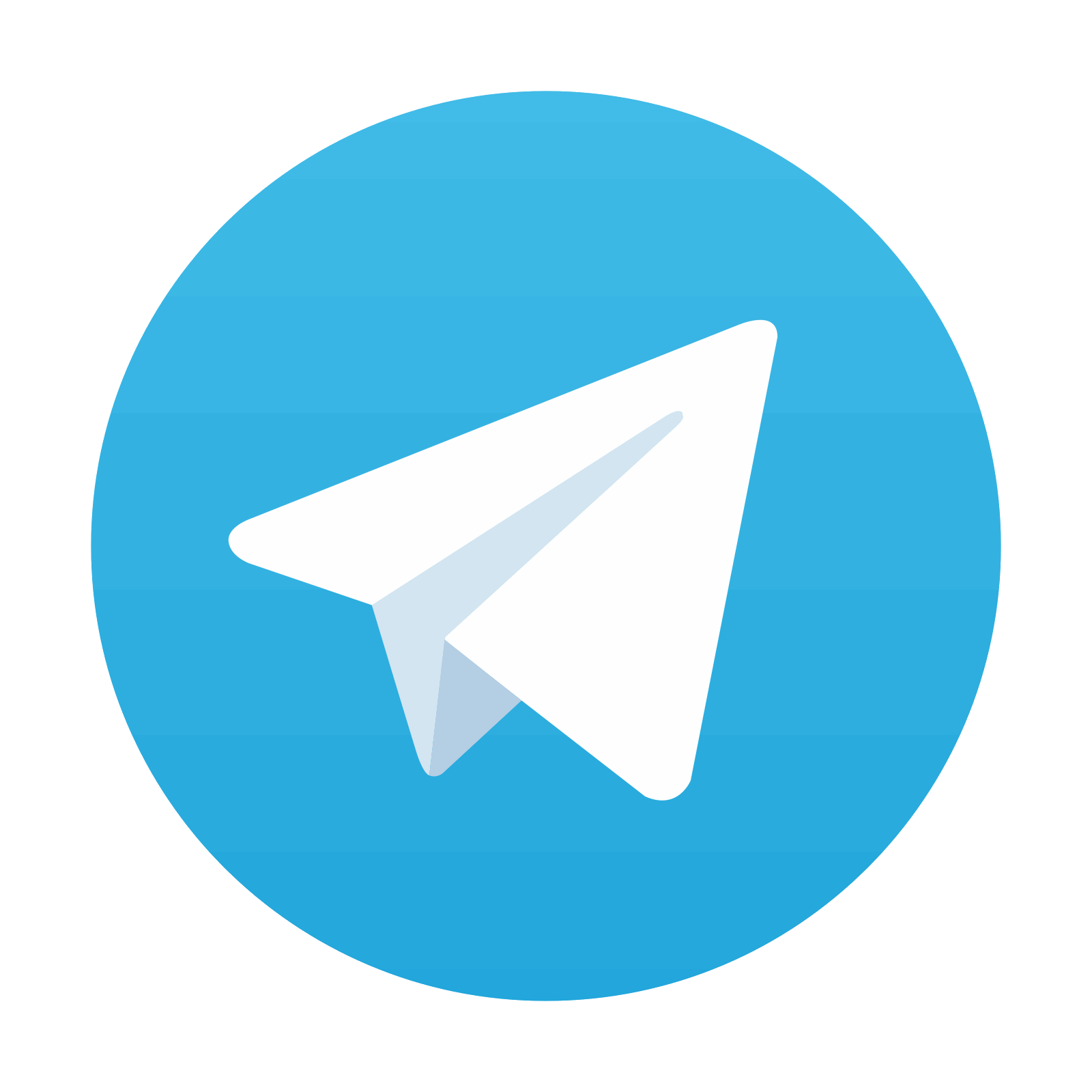
Stay updated, free dental videos. Join our Telegram channel

VIDEdental - Online dental courses
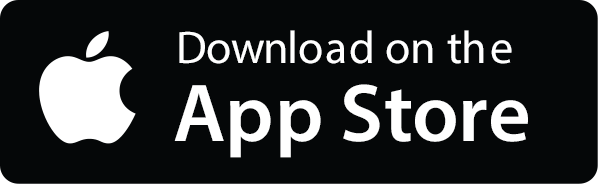
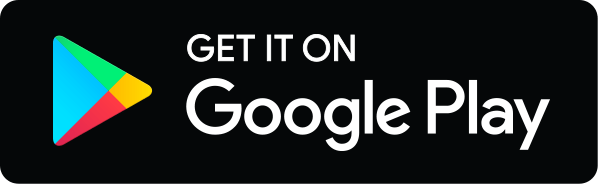