Head and neck vascular pathology is routinely encountered by the maxillofacial surgeon. Although these anomalies have been traditionally managed by surgical means, adjunctive therapies have been popularized in recent years. The use of laser therapy has gained attention for its ability to better access and to provide more predictable outcomes in the highly intricate and vascular areas of the head and neck. Laser therapy allows for the selective targeting of diseased tissue while maintaining the integrity of surrounding healthy tissue.
Key points
- •
The maxillofacial surgeon commonly encounters vascular pathology in the head and neck region such as hemangiomas, telangiectasia, and venous malformations.
- •
Surgical management of vascular anomalies in the head and neck region poses unique challenges in terms of accessibility and selectivity of the diseased tissue.
- •
Laser therapy has gained attention as an adjunct to surgical measures in the management of vascular pathology in this region for its ability to access and target selective tissue while maintaining the surrounding healthy tissue.
- •
Laser therapy may allow for a superior patient perioperative experience and minimal postoperative pain and recovery time when compared with the surgical management of such lesions.
Introduction
The maxillofacial surgeon will routinely encounter and manage pathology of the vasculature found in the head and neck region and will by default treat such pathology by surgical means. Nonsurgical treatment modalities, however, have emerged as both promising alternatives and adjunctive measures in the management of head and neck vasculature anomalies ( Fig. 1 ). Some of the most notable and emerging nonsurgical methods in practice today include drug therapies, medical lasers, image-guided embolization, and sclerotherapy. A combination of the aforementioned techniques, with or without a surgical component, is beginning to gain popularity in achieving an optimized clinical response when compared with surgery alone.

Given that the head and neck region is home to some of the most complex and delicate vasculature confined in such a small space when compared with any other region of the human body, the use of laser therapy in this region may be one of the most exciting emerging nonsurgical tools. Laser therapy allows the skillful surgeon to selectively target the diseased tissue while leaving the healthy tissue untouched, allowing for a much more conservative approach than standard surgical measures. Of course, every emerging medical tool comes with its laybacks; medical lasers as we know of today do not adequately penetrate the deeper tissues, making them a less useful tool when targeting the deep vessels of the head and neck. However, when used for appropriately indicated cases, laser therapy can provide superior cosmetic results without foregoing treatment outcomes. In addition, this treatment modality has proven to be a generally safe and comfortable procedure for not only the patient but the surgeon as well.
This article focuses on laser therapy, not as an all-in-one replacement for surgical procedures, but instead as an emerging tool that is quickly being adapted in the management of vascular lesions, particularly in maxillofacial surgery. The authors aim to describe the current state of medical lasers in this field, paying particular attention to their biophysics, clinical uses, and shortcomings. Nonsurgical adjuncts to laser therapy will also be discussed to comprehensively describe its realistic clinical application.
History of laser and current modalities
Although the fundamental concept of laser generation was popularized by Albert Einstein in 1916 with his “The Quantum Theory of Radiation,” the practical application of this theory was not introduced until 1960. Theodore Maiman, an American physicist, discovered the groundbreaking ruby crystal laser, which incorporated a high-intensity lamp to flash-excite native chromium electrons. On return of these chromium electrons to their original energy states, strong red light pulses would be generated resulting in what is now described as the “laser light.” With the advent of this laser beam system, laser application in medicine subsequently began to develop over the following decade. Advanced laser systems such as CO 2 , argon, and Nd:YAG grew tremendously at first in dermatology but rapidly escalated to other specialties.
Laser surgery was first set forth as a superior method to conventional surgical modalities for vascular lesions due to its thermal conduction. The continuous energy beam emitted by laser diodes would allow for thermal destruction of target tissues while maintaining hemostasis. Despite its advantages in highly vascularized sites, laser beam surgery was not free of limitations. The continuous high-energy beam was found to cause nonspecific tissue destruction, resulting in damage to healthy tissue and hypertrophic scar formation. It was not until 1981 that this problem was addressed through the discovery of “selective thermolysis” by Anderson and Parrish. This targeted tissue excision revolved around the concept of chromophores: specific wavelengths of light along the absorption spectrum would be managed preoperatively to spare healthy, nonvascular tissue.
In hopes of optimizing the selectivity of thermolysis, the laser was adjusted to be pulsatile in nature with adjustable wavelengths to maximize the targeted tissue’s absorption and minimize the competing components’ absorption. The time required for the tissue to lose 50% of its heat through diffusion, termed thermal relaxation, was another important component considered in the optimization of early lasers. It was also put forward that the pulse duration (duration of the beam) should be shorter than the thermal relaxation of the tissue in context. This rule ensures the confinement of the energy released by the laser within the targeted tissue and avoids its emission to the surroundings. In addition, the pulse duration must be of adequate duration for the needed thermal energy inside the vessel to obtain effects of clinical value. Although these principles align well with superficial lesions and the smaller vessel types of the head and neck region, they do not exactly apply to larger diameter vessels or those found at greater depths. In such cases, the appropriate depth of penetration may be achieved by adjusting the wavelength and pulse duration for sufficient thermal energy to build up within the vessel lumen.
The first-generation pulsed dye laser (PDL) was developed in the 1980s and produced light at 577 nm. It was introduced as a replacement for argon lasers, both for its increased safety and efficacy in the treatment of superficial vessel anomalies. The modern day PDLs produce light at 585- or 595-nm wavelengths and usually have built-in cooling systems, improving their efficiency and safety in penetrating greater depths while leaving adjacent tissue untouched. The flash lamp portion of the PDL produces yellow light at a particular wavelength by pumping energy through a rhodamine dye dissolved in a solvent. The energy generated is emitted by fiber-optic technology and shows preferential absorption by hemoglobin and oxyhemoglobin, resulting in thermal-induced damage and coagulative activity within the targeted vessel. The approximated thermal relaxation time of such small cutaneous vessels with diameters of less than 100μm is 1 to 5 ms. Depth continues to be a major limitation of modern day PDLs. Melanin in particular is believed to play a role in the laser’s inability to penetrate deeper depths because melanin itself absorbs light produced at the same wavelength as the PDL. This should especially be taken into account when using PDLs on patients with darker skin tones (Fitzpatrick skin types IV/V) because these individuals have a greater concentration of melanin. Given such limitations, PDL use is mainly reserved for vascular lesions of no more than 1.2 mm in depth.
In the head and neck region, the PDL is most commonly used in the setting of superficial vascular abnormalities such as capillary malformations (CMs), superficial infantile hemangiomas, and telangiectasias. Although considered a generally low-risk procedure, some common adverse effects of the use of PDLs for small cutaneous anomalies include erythema, edema, and purpura for up to 2 weeks post-operation. The purpura occurs due to red blood cell leakage out of the vessel walls due to the rapid thermal buildup induced by the laser and associated vessel damage. This adverse effect may be minimized or avoided altogether by using a lower peak energy, which is achieved by increasing the PDL. These adjustments result in a slower and less harmful thermal effect that does not precipitate absolute red blood cell leakage; documented events of purpura after the use of long-pulsed lasers are not known in the current literature. Nevertheless, it is imperative that the surgeon reviews these specific adverse effects before moving forward with PDL therapy, given that they are a common reason for patient refusal of treatment.
Frequency-Doubled Q-Switched Nd:YAG/Potassium Titanyl Phosphate Laser (532 nm)
As its name describes, the frequency-doubled, Q-switched Nd:YAG laser or KTP laser produces green light at 532 nm by using frequency-doubling techniques (second harmonic generation). The 532-nm wavelength is easily absorbed by oxyhemoglobin, making it advantageous in its use for laser thermolysis. Laser energy with a 1064-nm wavelength is produced by pumping the Nd:YAG crystal with a diode laser before focusing it onto a potassium titanyl phosphate (KTP) crystal. This technique results in a second light energy whose frequency is doubled and wavelength is halved to 532 nm (λ = v/f; λ = wavelength, f = frequency, v = velocity of light).
The 532-nm laser functions at a standard pulse duration of 1- to 50-ms fluence, generating energy as great as 40 J/cm 2 . Its utility over other lasers in targeting larger vessels is in part due to its longer pulse duration necessitated by the estimated thermal relaxation of larger vessels (1–10 ms). This phenomenon allows the laser to focus a substantial and effective amount of thermal energy within these larger vessels, specifically those with a diameter greater than 200 nm. The 532-nm laser was also quickly accepted for its malleability regarding pulse duration and fluence, allowing the clinician to carefully adjust such settings to serve the particular clinical case in question.
One of the major drawbacks of the frequency-doubled Nd:YAG is in regard to its activity with competing melanin. The melanin found in skin has better absorption of light at 532 nm compared with 585 nm, resulting in less penetration of the frequency-doubled Nd:YAG laser in skin types with highly concentrated melanin (ie, Fitzpatrick skin types IV and V). When used in such skin types, the laser may result in discoloration and blistering. This laser’s competing activity with melanin, along with the need for a lengthy pulse duration, seem to be the basis of such adverse clinical effects. The implementation of a cooling device perioperatively, however, adds a barrier of protection against unexpected skin damage. It should be noted that these cooling devices do not completely remove the risk of such adverse effects, and these lasers persistently carry a higher risk (18%) of scarring than the standard PDLs (3%). Overall, the frequency-doubled Nd:YAG serves as a reliable alternative in patients who fail to appropriately respond to PDL attempts or those hoping to achieve laser therapy with a lessened risk of developing purpura but should be used with caution in those with darker skin tones.
Long-Pulsed Nd:YAG Laser (1064 nm)
The year of 1964 introduced the 1064-nm Nd:YAG laser, which initially its use was reserved for procedures more cosmetic in nature such as hair removal and scar ablation. It was not until 30 years later in which its application for the management of certain vascular abnormalities was set forth. As the name implies, the 1064-nm Nd:YAG laser uses a wavelength of 1064 nm, functioning through a medium of impure yttrium-aluminum-garnet crystal doped by 1% neodymium ions. The 1064-nm Nd:YAG emerged as a drastically advanced laser; its lower level of absorption effectively reduced the laser’s activity with competing melanin, allowing it to reach vessels at depths (5–6 mm) that would otherwise be unreachable by previous lasers. Its lessened competition with melanin compared with previous lasers gained its popularity in the management of vessel anomalies in patients with Fitzpatrick skin types IV and V.
The better suited wavelength of this laser in the context of competing melanin, however, comes with unavoidable interactions with hemoglobin and oxyhemoglobin. These molecules absorb light at 1064 nm, adding a level of distraction between the laser and targeted vessels. The clinician may bypass these interactions by increasing the laser fluence as high as 300 J/cm 2 to ensure adequate generation of thermal energy to promote the coagulation within the targeted vessel. The pulse duration can also be appropriately adjusted between 0.1 and 300 ms to minimize damage imposed by the increased thermal energy on the surrounding tissue, doing so also minimizes the risk of purpura development postoperatively.
During routine practice, the laser is brought into direct contact with the cutaneous tissue. The clinician will make either a single or double pass over the target lesion until blanching or shrinkage of the lesion is observed clinically. Additional passes or persistent contact with the cutaneous tissue exposes the skin to probable postoperative damage and should be avoided. The number of passes over the targeted tissue should be kept at a minimum, and the laser itself should be in continuous movement. In practice, the type of lesion should also be considered; a nodular lesion may warrant the use of a glass slide to flatten the lesion and improve the penetrating depth. The location of the lesion is another characteristic to consider; for lesions with difficult accessibility (ie, palate, floor of the mouth), a test tube with a side window, termed the interstitial technique, may ameliorate the clinician’s ability to reach the lesion. A 14-gauge angio-catheter is used to inset a fiber-optic cable into the targeted lesion, allowing for more selective delivery of the laser energy. , This technique in particular helps protect the overlying tissue when ablating thicker lesions or accessing greater depths. It also may reduce the patient’s experienced duration of postoperative pain.
The longer pulsed laser provides reliable outcomes in terms of its use in the treatment of hemangiomas and vascular anomalies; it has been shown to result in a 50% improvement in 71.5% to 80% of all treated vascular lesions. , An additional study reported an absolute clearance rate of 77% in intraoral lesions after one session; persistent lesions were cleared with one or two additional sessions. Although such numbers offer promising results, the safety window of these longer pulsed lasers is rather narrow and must be approached with caution. As with previous lasers, a cooling device can help avoid the laser’s possibility of collateral damage such as blistering and scarring. The clinician should conservatively approach its use; short, multiple sessions offer a reduced risk of damaging effects compared with a single aggressive session.
CO 2 Laser (10,600 nm)
Although the human body is composed of 85% H 2 O, mucosal tissue consists of an even higher proportion of H 2 O, amounting to roughly 95%. This is largely attributed to the tissue’s increased vascularity and secretory contents. The CO 2 laser has therefore been considered a reliable method for ablative procedures because of its ability to penetrate water-rich structures. The thermal energy conducted by this type of laser is effective in both excision and hemostasis. Smaller blood vessels with diameters extending to 0.5 mm can be predictably cauterized by this method. , The CO 2 laser works under two operative modes: focused and defocused. The focused mode is effective in targeted excision, whereas the defocused setting allows for vaporization of tissue in a layer-by-layer fashion. The defocused mode is preferred in the setting of biopsy-proven dysplasia due to its usability and predictable postoperative healing. Despite its practicality, the clinician must take care in accounting for important anatomic structures due to this method’s risk of irregular depth penetration, a complication of tissue water content variability. Furthermore, this approach tends to obliterate tissue samples, thereby disabling concurrent biopsy procedures. The focused method in particular may allow for the gathering of intraoperative biopsy specimens; however, adjacent margins may still be altered, although to a lesser degree as compared with the defocused setting. In the use of focused CO 2 laser biopsy, larger margins may be necessary to include healthy tissue margins within the sample submitted for pathology. Nevertheless, one study focusing on the morphologic, histochemical, and immunocytochemical effects showed blisters, erosions, clefts of epithelium, and nuclei changes in samples retrieved via CO 2 laser.
CO 2 laser ablation has advantages in hemostasis, postoperative healing period, and usability, which directly correlates to reduced intraoperative time. This surgical approach creates an immediate hemostatic site that facilitates fibrin clot deposition and maturation. Furthermore, the unique tissue site created by CO 2 laser ablation delays and reduces inflammatory cell infiltrate, thereby resulting in diminished postoperative swelling. With this reduction in inflammation, postoperative pain has also been reported to be reduced in patients undergoing CO 2 laser surgery. This approach also decreases the amount of residual viable myofibroblasts, a cell-type integral in scar contracture. Owing to this unique feature, CO 2 laser has become a mainstay in surgical resection of pathology in the floor of the mouth as well as buccal mucosa. Wounds are left to heal by secondary intention and do not require primary closure or additional dressing application. For these reasons, CO 2 laser ablation has been adopted as a powerful tool in surgical approaches to maxillofacial pathology.
Diode Laser (532, 800, 810, 940, and 980 nm)
One of the most commonly used lasers by maxillofacial surgeons today is the diode laser, not only for its portability and economic unit system but more importantly for its adjustability and practicality. Engineered through a solid-state semiconductor, the light transmitted by this laser can be programmed to have variable intensity and wavelengths. In line with the principle of selective thermolysis, the 532-nm pulsed mode functions in alignment with the PDL and is effective in the management of superficial vascular defects while preserving surrounding tissues. Its differences lie in the diode laser’s capability of emitting longer pulsed energy (1–100 ms) in the absence of causing purpura because of its slower rate of vaporization, preventing vessel rupture. The diode laser can also reach deeper vessels when functioning at 800 to 900 nm and 3 to 5 W, all while sustaining a high rate of selectivity for hemoglobin chromophores. These particular parameters are selected for when aiming to penetrate deeper lesions that are resistant to standard PDL or KTP treatment.
The diode laser’s adjustable wavelength, power, and pulse duration are the foundation of its practicality in the clinical setting. The laser bypasses the somewhat narrow use of preceding laser therapies and into the realm of more complex and previously inaccessible anomalies such as superficial CMs, telangiectasias, and deep venous malformations (VMs). When applied continuously, the diode laser effectively acts as a scalpel in ablating highly vascularized defects while keeping the procedure hemostatic; the need for sutures is rarely indicated. Much of the diode laser’s popularity among maxillofacial surgeons can be attributed to its modifiable settings, which effectively allow it to carry the function of multiple previously mentioned lasers within a single unit ( Table 1 ).
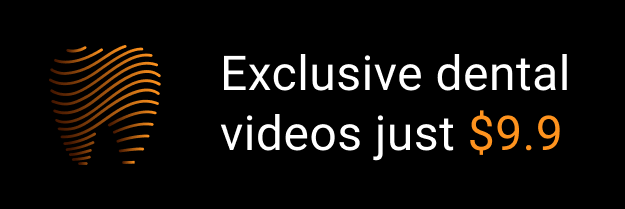