Abstract
Objective
To evaluate a range of mechanical parameters of composite resins and compare the data to the frequency of fractures and wear in clinical studies.
Methods
Based on a search of PubMed and SCOPUS, clinical studies on posterior composite restorations were investigated with regard to bias by two independent reviewers using Cochrane Collaboration’s tool for assessing risk of bias in randomized trials. The target variables were chipping and/or fracture, loss of anatomical form (wear) and a combination of both (summary clinical index). These outcomes were modelled by time and material in a linear mixed effect model including random study and experiment effects. The laboratory data from one test institute were used: flexural strength, flexural modulus, compressive strength, and fracture toughness (all after 24-h storage in distilled water). For some materials flexural strength data after aging in water/saliva/ethanol were available. Besides calculating correlations between clinical and laboratory outcomes, we explored whether a model including a laboratory predictor dichotomized at a cut-off value better predicted a clinical outcome than a linear model.
Results
A total of 74 clinical experiments from 45 studies were included involving 31 materials for which laboratory data were also available. A weak positive correlation between fracture toughness and clinical fractures was found (Spearman rho = 0.34, p = 0.11) in addition to a moderate and statistically significant correlation between flexural strength and clinical wear (Spearman rho = 0.46, p = 0.01). When excluding those studies with “high” risk of bias (n = 18), the correlations were generally weaker with no statistically significant correlation. For aging in ethanol, a very strong correlation was found between flexural strength decrease and clinical index, but this finding was based on only 7 materials (Spearman rho = 0.96, p = 0.0001). Prediction was not consistently improved with cutoff values.
Significance
Correlations between clinical and laboratory outcomes were moderately positive with few significant results, fracture toughness being correlated with clinical fractures and flexural strength with clinical wear. Whether artificial aging enhances the prognostic value needs further investigations.
1
Introduction
The mechanical stability of direct dental materials is one of the prerequisites for the long-term clinical success of restorations made of these materials . Other important parameters are e.g., biocompatibility and colour stability . Due to mastication during food consumption, unconscious bruxism at night or during the day or gnashing, restored and unrestored teeth are submitted to a multitude of mechanical and chemical interaction in the oral cavity . If the mechanical loading surpasses the material-inherent ability to withstand occlusal forces during mastication and bruxism, cracks and fractures of the material may ensue. Especially multi-surface restorations in both the posterior and anterior region are at risk of material fractures, particularly Class II restorations which restore the proximal–part of the tooth and/or even cusps and Class IV restorations which include the restoration of the incisal edge . Recent published systematic reviews showed that fracture is one of the most frequent reasons of failure of composite resin in posterior teeth .
More fractures occur in patients with high chewing forces; especially bruxist patients are at risk of fractures . Another consequence of the mechanical loading of the material is the two- and three body wear of especially the occlusal surface, which is clinically expressed as loss of anatomical shape . If we take into consideration the quantity of resin material sold , around 800 million composite resin restorations were placed worldwide in 2015 alone; about 80% were placed in the posterior region and 20% in the anterior region. A meta-analysis on posterior resin restorations has shown that at least 5% of them will fail due to fracture of the material and about 12% will show noticeable wear within an observation period of 10 years . In other words, at least about 32 million of posterior resin restorations placed in 2015 will be replaced or will need repair work due to fracturing by 2025.
One way to diminished the economical impactis to better evaluate resin composites in vitro. Ferracane evaluated different tests that may be appropriate for the prediction of the clinical performance . The author notes that there is low evidence that clinical wear is related to flexural strength, fracture toughness and degree of conversion of the polymer matrix. He concluded though that the overall clinical success of dental composites is multi-factorial and therefore it is unlikely that even a battery of in vitro test methods accurately predicts the clinical performance.
The laboratory tests used to characterize the mechanical stability of artificial materials are numerous and include flexural strength tests of different kinds (e.g., three-point/four-point bending test, biaxial flexural strength), tensile strength test, compressive strength test, fracture toughness, various surface hardness tests and tests to determine the modulus of elasticity. Furthermore, specimens can be tested after 24 h of immersion in water or after artificial aging. Other concepts to determine the mechanical behavior of composite resin propose dynamic loading tests to determine the fatigue resistance of specific materials .
The ISO standard 4049 on polymer based restorative materials describes only the three-point flexural strength test after 24 h storage in water . This test is also the most commonly used test . For load-bearing restorations in the posterior region (Class I/Class II) the test demands a mean minimal flexural strength value of 80 MPa. No other mechanical test is included in any ISO standard related to dental polymer materials.
To assess the wear behavior, different laboratory wear tests have been developed. In 2001, the International Organization for Standardization ISO published a Technical Specification on “Guidance on testing of wear”, describing 8 different test methods of two- and/or three-body contact . These test methods vary with regard to load, number of cycles and their frequency, abrasive medium, type of force actuator, sliding movement, etc. In addition to these tests, numerous other tests have been developed. Many of these tests fail to define a qualification protocol for the test equipment or a validation procedure for the test method to run in conjunction with the equipment . Both qualification and validation, however, are indispensable prerequisites for a test to become a standard laboratory test .
The mechanical stability of composite resin materials decreases with increased time in a wet environment and interaction with the bacterial biofilm and saliva, resulting in the absorption of water, leaching of monomers and loss of fillers and this will eventually lead to a degradation of the filled resin . Therefore, some research workers claim that mechanical testing of resin composite should only be carried out after artificial aging. There are different methods for artificial aging such as thermocycling with hot and cold water, typically at 5 °C and 55 °C, prolonged storage in water or artificial saliva or storage in ethanol. A laboratory study showed that flexural strength significantly decreased in 3 out of 12 analyzed resin composites after one month of water storage and 5000 cycles of thermocycling . Compomer materials are especially affected by the loss of mechanical stability after artificial aging and show also more fractures in clinical trials .
The question is: what are the reasons for the material fractures? Possible influencing factors are: (1) the quality of the restoration, which is influenced by the quality and skill of the operator; (2) patient-specific factors like chewing force, habits, etc. or (3) material-dependent factors like the composition of the material which influences the long-term mechanical properties. Another explanation could also be that the material class of composite resin as such does have an inherent risk of possible material fractures, especially in regions where it is mainly stressed due to shear movements like the anterior region. The above-mentioned meta-analysis of posterior restorations did not show any statistically significant difference with regard to the type of resin material for the posterior region (microfill, macrofill, hybrid) . In Class IV restorations, however, hybrid composite showed significantly less fracture than micro- and macrofilled composites . In addition, the meta-analysis on Class II restorations also reveals a similar frequency of fractures in amalgam and resin composite fillings . However, restorations with compomer materials showed significantly more chippings than restorations made of the other types of material (9% after 10 years on average, compared to 5%). In other meta-analyses, however, amalgam restorations showed significantly more fractures than composite restorations . The reason for the latter finding could be that more extensive restorations were placed in these studies.
The above-mentioned results from clinical trials may indicate that the mechanical properties as measured in the laboratory play a crucial role as compomer and microfilled materials showed lower flexural strength and lower fracture toughness than composite resin materials . The first indication in the literature about the correlation of a material property like fracture toughness and the frequency of fractures of Class IV restorations was published as early as 1990 . To our knowledge there is, however, no systematic review on the correlation between mechanical parameters of resin composite materials and the clinical fracture/wear rate of the same materials.
For the present study, an existing data-base on clinical trials on posterior composite resin restorations has been up-dated using the same inclusion criteria. However, in this study an additional criterion has been included: the bias that was assessed by established criteria. Therefore, an additional research question was formulated to examine whether bias or no bias of a clinical trial alters the correlation with the laboratory data. The reasons for bias include non-randomization of subjects, lack of blinding/masking of operators and evaluators, sponsorship by third parties, especially industry, insufficient reporting of drop-outs and the reason for the drop-outs, etc. . A systematic review on meta-analyses and systematic reviews in dentistry revealed that studies indexed in MEDLINE present greater effects than non-indexed ones and small randomized trials present greater effects than large ones .
The following null hypotheses were formulated:
- 1.
There is no correlation between any of the laboratory tests (flexural strength [after 24 h and after aging in water/saliva/ethanol] and flexural modulus, compressive strength, and fracture toughness after 24-h storage in distilled water) and the frequency of material fractures/chipping in prospective clinical studies evaluated according to their bias.
- 2.
There is no correlation between any of the laboratory tests (flexural strength [after 24 h and after aging in water/saliva/ethanol] and flexural modulus, compressive strength, and fracture toughness after 24-h storage in distilled water) and the frequency of loss of anatomical contour in prospective clinical studies.
2
Material and methods
2.1
Selection of in vivo studies
For the clinical performance of posterior composite resin restorations, results from prospective studies on Class I/II were retrieved from the literature. The database used for the meta-analysis on Class I/II composite resin restorations and published in the Journal of Adhesive Dentistry in 2010 was up-dated with studies that were published between May 2011 and April 2015, using the same criteria as described in the publication, the same databases (PubMed and SCOPUS) and the same search words. Only those studies on composite materials were selected for whom also laboratory data had been published.
The inclusion criteria were as follows:
- 1
Prospective clinical trial in Class II cavities or in Class I and Class II cavities.
- 2
Minimal duration of 2 years.
- 3
Minimal sample size at last recall: 20 restorations per material group.
- 4
The study had to report on the following outcome variables: material fractures and anatomical shape.
For both variables, the data were graded into three categories:
1 = good or very good, corresponds to Ryge criterion “Alpha”.
2 = acceptable or repairable, corresponds to Ryge criteria “Beta” or “Charlie”.
3 = inacceptable needing replacement, corresponds to Ryge criterion “Delta”.
- 5
These categories are based on the FDI criteria on the evaluation of posterior restoration . These criteria comprise 5 grades. In the present study grade “1” and “2” were summed up to “1” and “3” and “4” to “2”.
- 6
The study had to report on the applied materials, conditioning technique of teeth as well as the operative technique, e.g., bevelling of enamel, preparation, isolation technique, type of curing, and layering technique.
Studies that evaluated flowable bulk fill materials (up to 4 mm layer thickness) that needed a capping layer with a high viscous composite resin materials were excluded. However, clinical studies with a normal flowable resin as first layer with a layer thickness of 1–1.5 mm were taken into consideration and so were studies with a first layer of a glass ionomer cement.
Quality assessments of the selected clinical studies were carried out by two independent reviewers, using the Cochrane Collaboration’s tool for assessing risk of bias in randomized trials . The assessment criteria contain six items: sequence generation, allocation concealment, blinding of the outcome assessors, incomplete outcome data, selective outcome reporting, and other possible sources of bias. However, only adequate sequence generation, allocation concealment and examiner blinding were considered as key domains.
During data selection and quality assessment, any disagreements between the reviewers were solved through discussion, and if needed, by consulting a third reviewer. The risk of bias was scored following recommendations as described in the Cochrane Handbook for Systematic Reviews of Interventions 5.1.0 ( ). At domain level, the judgment for each entry involved recording “yes”, indicating low risk of bias, “no” indicating high risk of bias, and “unclear” indicating either lack of information or uncertainty regarding the potential for bias. At the study level, the study was considered to be at “low” risk of bias if two or three key domains for each outcome were at “low” risk of bias. If two or more key domains were judged as “unclear”, the study as a whole was considered as “unclear”. If two or more key domains were judged as “high” risk of bias, the study as a whole was considered at “high” risk of bias.
2.2
Selection of in vitro studies
For laboratory studies on dental resin composites, only data from one study center were included to reduce the variability due to different operators, modification of tests, different test equipment, etc. The test center was the Department of Operative Dentistry and Periodontology at the Ludwig-Maximilian-University in Munich (Germany). All the data that were to be used for the in vitro/in vivo correlation had already been published in dental journals. In one publication, data on the flexural strength, flexural modulus, diametric tensile strength and compressive strength of 72 hybrid, nano-hybrid, micro-filled, packable, ormocer-based composite, flowable composites, compomers and flowable compomers were published . Another study that was published 4 years later investigated the flexural strength, flexural modulus, indentation modulus, Vickers hardness and creep of 34 nano-hybrid, micro-hybrid and flowable composite resin materials . However, data of several identical composite materials were published in both investigations. If data were present for the same material in both publications and clinical data were also present, the data of the second, more recent publication was used. In two other publications from the same research institute, composite resin materials were artificially aged with 5000 cycles thermocycling and subsequent storage in either ethanol, water, or artificial saliva . The same research center assessed the fracture toughness of 69 micro-hybrid, nano-filled, micro-filled, packable, ormocer-based, and flowable resin-based composites, compomers and flowable compomers, as well as conventional glass ionomer cements and resin-modified GIC .
For the details on the laboratory test methods, the authors refer the reader to the original publications. In what follows is a brief description of the test methods.
The flexural strength and flexural modulus were determined in a three-point bending test according to ISO/DIN 4049 and for fracture toughness (KI C ) was determined according to ASTM test E 399-83 , using single-edge notched-beam specimens of each material. Eight specimens were fabricated for each material and for each test. The specimens were made by compressing the composite material between two glass plates with intermediate polyacetate sheets, separated by a steel mold, which had an internal dimension of 16 mm length × 2 mm height × 2 mm width. The material was cured for 40 s from both sides in a light-curing device (Dentacolor XS, Kulzer, Wehrheim). The specimens were ground with silicon carbide sand paper [grit size P 1200/4000 (Leco)] – after removal from the mold. All specimens were then stored in distilled water at 37 °C prior to testing after 24 h. For the fracture toughness test a notch (0.3 mm wide, 1 mm deep) was machined for each specimen prior to testing with a diamond saw (Proxxon, Föhren, Germany) using water coolant. The depth of the notch was measured with a light microscope; it varied between 0.90 mm and 1.10 mm. For the flexural strength and the fracture toughness test specimens were loaded until failure in a universal testing machine (MCE 2000ST, quick test Prüfpartner GmbH, Langenfeld, Germany) using a three-point bending test device. During testing, the specimens were immersed in distilled water at room temperature. The crosshead speed was 0.5 mm/min. The bending modulus was calculated from the slope of the linear part of the force-deflection diagram .
The compressive strength was determined on cylindrical specimens (8 mm in height and 4 mm in diameter) made in a Teflon mold by curing and storing the specimens similar as described previously (n = 8 per material). The specimens were placed with the flat end on the supporting plate of the universal testing machine and a compressive load was applied axially at a crosshead speed of 0.5 mm/min.
For artificial aging, the specimens were first thermocycled for 5000 cycles at 5 °C and 55 °C. Afterwards, the specimens were randomly divided into groups of 20 (specimens), stored in either distilled water, commercial artificial saliva or a 50:50 mixture of 96% ethanol and distilled water for four weeks at 37 °C. The storage solutions were exchanged daily, keeping the volume of liquid constant. The composition of the artificial saliva was as follows (ref 1 L; pH 6.9): Potassium chloride 1.2 g, sodium chloride 0.84 g, potassium phosphate 0.26 g, calcium-chloride-dihydrate 0.14 g.
2.3
Statistical analysis
2.3.1
Modeling and summarizing in vivo studies
In vivo outcomes include the probability to be free of clinical fracture and the probability not to undergo a loss of anatomical form (wear), where high probabilities represent good outcomes. We have applied a monotonic log–log transformation to convert these probabilities (which are bounded quantities between 0% and 100%) into unbounded quantities which can be modeled via a linear model. As applied in a previous study on the efficacy of Class II restorations , the dependence of an in vivo outcome on time t and material j was modeled as:
with a different slope (fixed effect) beta_j for each available material, to constrain these probabilities to be 100% at time t = 0 while decreasing monotonously with increasing values of t. Note that a linear decrease on the transformed scale implies a non-linear decrease on the original scale, where our model ensured that these probabilities do not fall below 0%. A random study effect and a random experiment effect were included in this model to take into account the fact that we had repeated measurements along time implying partly the same subjects, yielding a linear mixed model. Each measurement was weighted by the number of subjects available at a given time point, to give more weight to those measurements based on more subjects when estimating the coefficients in this model. The nlme package from the R statistical software (version 3.1.0) was used to fit these models. Each in vivo outcome parameter was considered twice, using either all clinical studies available or excluding those studies with “high” risk of bias.
In addition we included three potential confounding variables (treated as categorical factors) in the model:
- –
tooth type ratio (percentage of the number of restored molars in relation to premolars): ≤25%, 25–50%, 50–75%, >75%.
- –
Class I:Class II ratio (percentage of the number of Class I restorations of molars in relation to Class II restorations): 0%, 0–50%, >50%.
- –
rubber dam (yes/no/missing information).
A slope beta_j in our model characterized thus the in vivo performance of a specific material j (the higher, the better). Since these slopes are not necessarily straightforward to interpret, they were standardized, i.e., they were divided by a robust estimate of their standard deviation after subtraction of their median, obtaining a standard deviation score (SDS). A SDS value of 0 indicates average performance while a SDS value of e.g., +1 indicates one standard deviation above average (which is good), and a SDS value of e.g., −2 indicates two standard deviations below average (which is bad).
Using the SDS allows a meaningful combination of several indicators to obtain a summary index. A clinical index to summarize the in vivo performance of a given material was thus obtained by averaging its available SDS (as standardized slopes) with respect to clinical fracture and wear.
2.3.2
Summarizing in vitro studies
Similarly, a laboratory index to summarize the in vitro performance of a given material was obtained by averaging its available SDS with respect to e.g., flexural strength [after 24 h and after aging in water/saliva/ethanol] and flexural modulus, compressive strength, and fracture toughness, where SDS was calculated by dividing the raw values of the corresponding in vitro outcome by a robust estimate of their standard deviation after subtraction of their median. Here also, a positive SDS for a given material represents a performance above average and a negative SDS a performance below average.
2.3.3
Correlation in vivo versus in vitro performance
In vivo outcomes (i.e., the slopes expressed as SDS for the different in vivo outcomes and the clinical index) were correlated with the in vitro outcomes (including the laboratory index) over all available materials via Spearman correlation. The goal was to see whether and to which extent the materials with a good in vitro performance were identical with the materials with a good in vivo performance.
Correlations for which the p-value was smaller than 0.05 were considered as statistically significant. For the main in vivo outcomes (clinical fracture, wear and the clinical index) and the main in vitro outcomes (fracture toughness, flexural strength and the laboratory index), we explored whether a linear model to predict the in vivo performance in function of the in vitro performance explained more or less accurately the variance of the in vivo response than a model with a jump at some optimal (cut-off) value of the in vitro predictor. For each combination of in vitro predictor and in vivo response, we thus selected the optimal position for a jump (i.e., maximizing R-squared) on some pre-specified grid of values for the in vitro predictor and compared the R-squared obtained in the process with the R-squared obtained from a linear model.
2
Material and methods
2.1
Selection of in vivo studies
For the clinical performance of posterior composite resin restorations, results from prospective studies on Class I/II were retrieved from the literature. The database used for the meta-analysis on Class I/II composite resin restorations and published in the Journal of Adhesive Dentistry in 2010 was up-dated with studies that were published between May 2011 and April 2015, using the same criteria as described in the publication, the same databases (PubMed and SCOPUS) and the same search words. Only those studies on composite materials were selected for whom also laboratory data had been published.
The inclusion criteria were as follows:
- 1
Prospective clinical trial in Class II cavities or in Class I and Class II cavities.
- 2
Minimal duration of 2 years.
- 3
Minimal sample size at last recall: 20 restorations per material group.
- 4
The study had to report on the following outcome variables: material fractures and anatomical shape.
For both variables, the data were graded into three categories:
1 = good or very good, corresponds to Ryge criterion “Alpha”.
2 = acceptable or repairable, corresponds to Ryge criteria “Beta” or “Charlie”.
3 = inacceptable needing replacement, corresponds to Ryge criterion “Delta”.
- 5
These categories are based on the FDI criteria on the evaluation of posterior restoration . These criteria comprise 5 grades. In the present study grade “1” and “2” were summed up to “1” and “3” and “4” to “2”.
- 6
The study had to report on the applied materials, conditioning technique of teeth as well as the operative technique, e.g., bevelling of enamel, preparation, isolation technique, type of curing, and layering technique.
Studies that evaluated flowable bulk fill materials (up to 4 mm layer thickness) that needed a capping layer with a high viscous composite resin materials were excluded. However, clinical studies with a normal flowable resin as first layer with a layer thickness of 1–1.5 mm were taken into consideration and so were studies with a first layer of a glass ionomer cement.
Quality assessments of the selected clinical studies were carried out by two independent reviewers, using the Cochrane Collaboration’s tool for assessing risk of bias in randomized trials . The assessment criteria contain six items: sequence generation, allocation concealment, blinding of the outcome assessors, incomplete outcome data, selective outcome reporting, and other possible sources of bias. However, only adequate sequence generation, allocation concealment and examiner blinding were considered as key domains.
During data selection and quality assessment, any disagreements between the reviewers were solved through discussion, and if needed, by consulting a third reviewer. The risk of bias was scored following recommendations as described in the Cochrane Handbook for Systematic Reviews of Interventions 5.1.0 ( ). At domain level, the judgment for each entry involved recording “yes”, indicating low risk of bias, “no” indicating high risk of bias, and “unclear” indicating either lack of information or uncertainty regarding the potential for bias. At the study level, the study was considered to be at “low” risk of bias if two or three key domains for each outcome were at “low” risk of bias. If two or more key domains were judged as “unclear”, the study as a whole was considered as “unclear”. If two or more key domains were judged as “high” risk of bias, the study as a whole was considered at “high” risk of bias.
2.2
Selection of in vitro studies
For laboratory studies on dental resin composites, only data from one study center were included to reduce the variability due to different operators, modification of tests, different test equipment, etc. The test center was the Department of Operative Dentistry and Periodontology at the Ludwig-Maximilian-University in Munich (Germany). All the data that were to be used for the in vitro/in vivo correlation had already been published in dental journals. In one publication, data on the flexural strength, flexural modulus, diametric tensile strength and compressive strength of 72 hybrid, nano-hybrid, micro-filled, packable, ormocer-based composite, flowable composites, compomers and flowable compomers were published . Another study that was published 4 years later investigated the flexural strength, flexural modulus, indentation modulus, Vickers hardness and creep of 34 nano-hybrid, micro-hybrid and flowable composite resin materials . However, data of several identical composite materials were published in both investigations. If data were present for the same material in both publications and clinical data were also present, the data of the second, more recent publication was used. In two other publications from the same research institute, composite resin materials were artificially aged with 5000 cycles thermocycling and subsequent storage in either ethanol, water, or artificial saliva . The same research center assessed the fracture toughness of 69 micro-hybrid, nano-filled, micro-filled, packable, ormocer-based, and flowable resin-based composites, compomers and flowable compomers, as well as conventional glass ionomer cements and resin-modified GIC .
For the details on the laboratory test methods, the authors refer the reader to the original publications. In what follows is a brief description of the test methods.
The flexural strength and flexural modulus were determined in a three-point bending test according to ISO/DIN 4049 and for fracture toughness (KI C ) was determined according to ASTM test E 399-83 , using single-edge notched-beam specimens of each material. Eight specimens were fabricated for each material and for each test. The specimens were made by compressing the composite material between two glass plates with intermediate polyacetate sheets, separated by a steel mold, which had an internal dimension of 16 mm length × 2 mm height × 2 mm width. The material was cured for 40 s from both sides in a light-curing device (Dentacolor XS, Kulzer, Wehrheim). The specimens were ground with silicon carbide sand paper [grit size P 1200/4000 (Leco)] – after removal from the mold. All specimens were then stored in distilled water at 37 °C prior to testing after 24 h. For the fracture toughness test a notch (0.3 mm wide, 1 mm deep) was machined for each specimen prior to testing with a diamond saw (Proxxon, Föhren, Germany) using water coolant. The depth of the notch was measured with a light microscope; it varied between 0.90 mm and 1.10 mm. For the flexural strength and the fracture toughness test specimens were loaded until failure in a universal testing machine (MCE 2000ST, quick test Prüfpartner GmbH, Langenfeld, Germany) using a three-point bending test device. During testing, the specimens were immersed in distilled water at room temperature. The crosshead speed was 0.5 mm/min. The bending modulus was calculated from the slope of the linear part of the force-deflection diagram .
The compressive strength was determined on cylindrical specimens (8 mm in height and 4 mm in diameter) made in a Teflon mold by curing and storing the specimens similar as described previously (n = 8 per material). The specimens were placed with the flat end on the supporting plate of the universal testing machine and a compressive load was applied axially at a crosshead speed of 0.5 mm/min.
For artificial aging, the specimens were first thermocycled for 5000 cycles at 5 °C and 55 °C. Afterwards, the specimens were randomly divided into groups of 20 (specimens), stored in either distilled water, commercial artificial saliva or a 50:50 mixture of 96% ethanol and distilled water for four weeks at 37 °C. The storage solutions were exchanged daily, keeping the volume of liquid constant. The composition of the artificial saliva was as follows (ref 1 L; pH 6.9): Potassium chloride 1.2 g, sodium chloride 0.84 g, potassium phosphate 0.26 g, calcium-chloride-dihydrate 0.14 g.
2.3
Statistical analysis
2.3.1
Modeling and summarizing in vivo studies
In vivo outcomes include the probability to be free of clinical fracture and the probability not to undergo a loss of anatomical form (wear), where high probabilities represent good outcomes. We have applied a monotonic log–log transformation to convert these probabilities (which are bounded quantities between 0% and 100%) into unbounded quantities which can be modeled via a linear model. As applied in a previous study on the efficacy of Class II restorations , the dependence of an in vivo outcome on time t and material j was modeled as:
with a different slope (fixed effect) beta_j for each available material, to constrain these probabilities to be 100% at time t = 0 while decreasing monotonously with increasing values of t. Note that a linear decrease on the transformed scale implies a non-linear decrease on the original scale, where our model ensured that these probabilities do not fall below 0%. A random study effect and a random experiment effect were included in this model to take into account the fact that we had repeated measurements along time implying partly the same subjects, yielding a linear mixed model. Each measurement was weighted by the number of subjects available at a given time point, to give more weight to those measurements based on more subjects when estimating the coefficients in this model. The nlme package from the R statistical software (version 3.1.0) was used to fit these models. Each in vivo outcome parameter was considered twice, using either all clinical studies available or excluding those studies with “high” risk of bias.
In addition we included three potential confounding variables (treated as categorical factors) in the model:
- –
tooth type ratio (percentage of the number of restored molars in relation to premolars): ≤25%, 25–50%, 50–75%, >75%.
- –
Class I:Class II ratio (percentage of the number of Class I restorations of molars in relation to Class II restorations): 0%, 0–50%, >50%.
- –
rubber dam (yes/no/missing information).
A slope beta_j in our model characterized thus the in vivo performance of a specific material j (the higher, the better). Since these slopes are not necessarily straightforward to interpret, they were standardized, i.e., they were divided by a robust estimate of their standard deviation after subtraction of their median, obtaining a standard deviation score (SDS). A SDS value of 0 indicates average performance while a SDS value of e.g., +1 indicates one standard deviation above average (which is good), and a SDS value of e.g., −2 indicates two standard deviations below average (which is bad).
Using the SDS allows a meaningful combination of several indicators to obtain a summary index. A clinical index to summarize the in vivo performance of a given material was thus obtained by averaging its available SDS (as standardized slopes) with respect to clinical fracture and wear.
2.3.2
Summarizing in vitro studies
Similarly, a laboratory index to summarize the in vitro performance of a given material was obtained by averaging its available SDS with respect to e.g., flexural strength [after 24 h and after aging in water/saliva/ethanol] and flexural modulus, compressive strength, and fracture toughness, where SDS was calculated by dividing the raw values of the corresponding in vitro outcome by a robust estimate of their standard deviation after subtraction of their median. Here also, a positive SDS for a given material represents a performance above average and a negative SDS a performance below average.
2.3.3
Correlation in vivo versus in vitro performance
In vivo outcomes (i.e., the slopes expressed as SDS for the different in vivo outcomes and the clinical index) were correlated with the in vitro outcomes (including the laboratory index) over all available materials via Spearman correlation. The goal was to see whether and to which extent the materials with a good in vitro performance were identical with the materials with a good in vivo performance.
Correlations for which the p-value was smaller than 0.05 were considered as statistically significant. For the main in vivo outcomes (clinical fracture, wear and the clinical index) and the main in vitro outcomes (fracture toughness, flexural strength and the laboratory index), we explored whether a linear model to predict the in vivo performance in function of the in vitro performance explained more or less accurately the variance of the in vivo response than a model with a jump at some optimal (cut-off) value of the in vitro predictor. For each combination of in vitro predictor and in vivo response, we thus selected the optimal position for a jump (i.e., maximizing R-squared) on some pre-specified grid of values for the in vitro predictor and compared the R-squared obtained in the process with the R-squared obtained from a linear model.
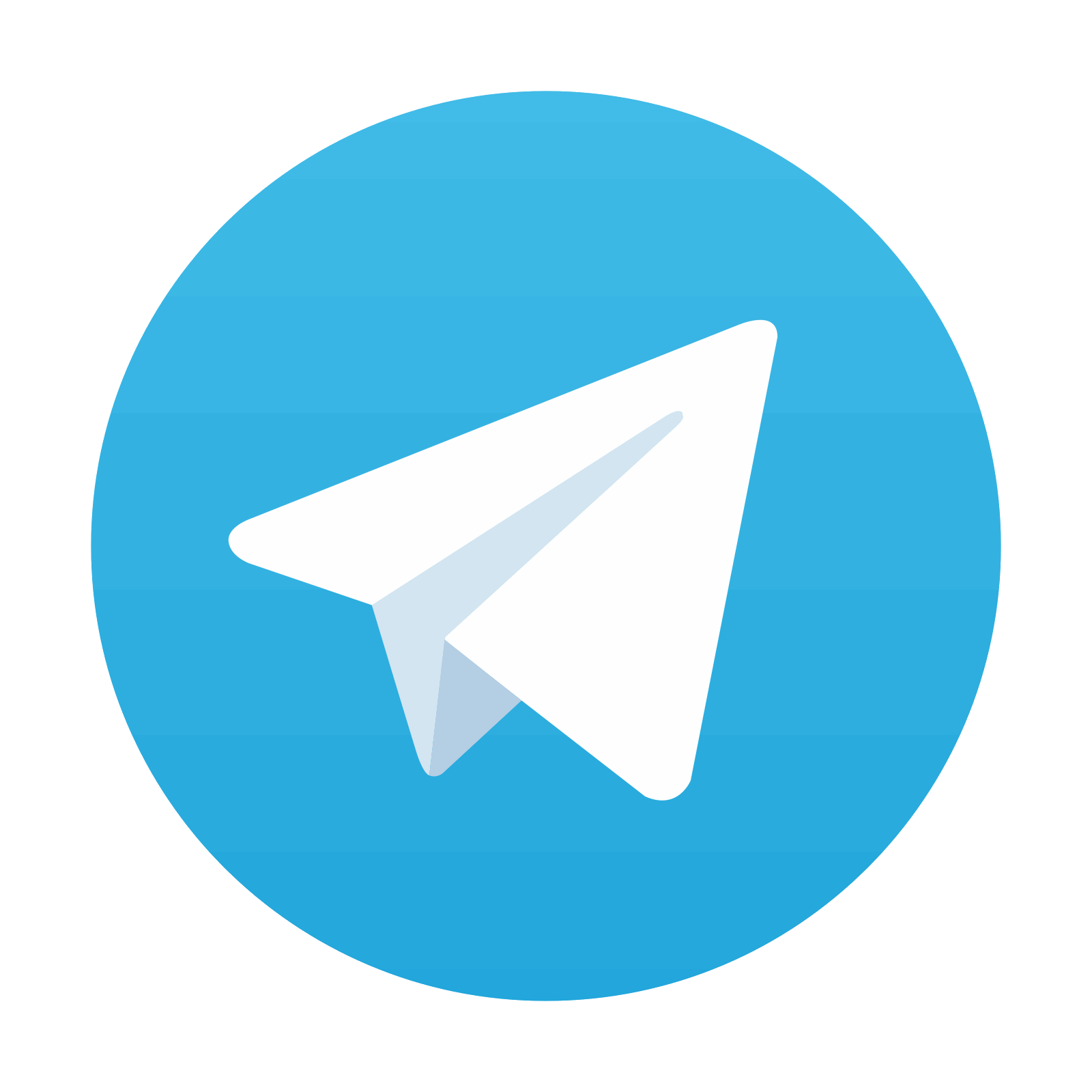
Stay updated, free dental videos. Join our Telegram channel

VIDEdental - Online dental courses
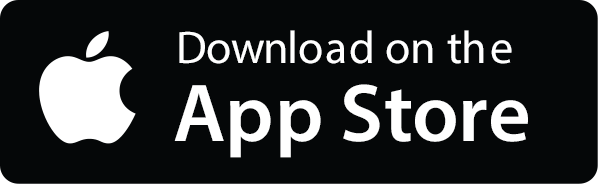
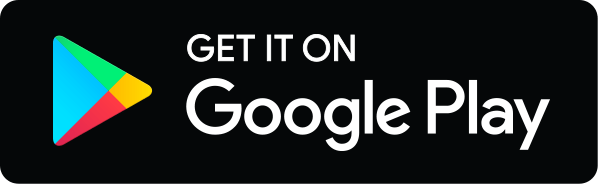