Abstract
Leucocyte- and platelet-rich plasma (L-PRP) is an autogenous platelet concentrate enriched with leukocytes that releases various growth factors responsible for the proliferation, regulation, and differentiation of mesenchymal cells during wound healing. Since the bone and medullary tissue are contiguous and share the same origin, this study evaluated the effect of L-PRP on the repair of calvaria bone using histomorphometric analysis of the newly formed bone, and compared the results in the presence of osteocalcin (OC) and peroxisome proliferator-activated receptor gamma (PPAR-γ) detected by immunohistochemistry. Artificial circular bone defects (5 mm diameter) were produced in the calvaria of 42 rats. The defects were treated with autograft, autograft combined with L-PRP, or without grafting material (sham). The animals were euthanized at 15 or 40 days postsurgery ( n = 7 in each group). Data obtained were analyzed by Student–Newman–Keuls test for histomorphometric and immunohistochemical interpretation. The development of bone matrix was significantly less in the defects treated with L-PRP, while the medullary area composed of fatty cells was larger. This coincided with the minor expression of OC and expressive presence of PPAR-γ. These results suggest that L-PRP may impair osteoneogenesis and alter the ratio of differentiation between bone matrix and fatty cells, increasing the medullary tissue.
Introduction
Platelet-rich plasma (PRP) is a non-toxic and non-antigenic autologous blood product enriched with platelets that has been considered an essential source of growth factors, which are thought to improve wound healing in both soft and hard tissue. Since Marx et al. reported that the use of PRP promotes faster maturation of autologous bone grafts and a consequent increase in new bone formation in mandibular defects, the use of PRP combined with autografts has been used frequently in the reconstruction of craniofacial deformities.
However, the positive effects of PRP in osteoneogenesis are the subject of debate and several studies have revealed a failure of bone maturation when PRP is used. A relevant speculation in the divergent results produced by PRP combined with autograft has been assigned in particular to the fabrication of PRP. While the classical definition of PRP, as proposed by Marx et al., would suggest a pure mixture of plasma and platelets, the usual definition of PRP has expanded to include a variety of final products; thus the PRP may vary markedly not only in the final concentration of platelets, but also in the amounts of leukocytes that are commonly included in the final preparation.
Leukocytes are considered to play a key role in the preliminary phases of inflammation and they may contribute to the variation in results found in the literature regarding the efficiency of bone wound healing. This hypothesis seems likely given that these inflammatory cells commonly increase the levels of some platelet growth factors, especially transforming growth factor beta 1 (TGF-β1), which is also present in bone loss.
The presence of platelet growth factors is considered important to bone turnover and repair, since they contribute to the chemotaxis of osteoprogenitor cells at the site of injury. In contrast, some studies have revealed that TGF-β1 in particular may also induce negative effects on osteoblastogenesis, since this cytokine antagonizes bone morphogenetic protein (BMP) expression and impairs osteoblast maturation; this may contribute to changes in the ratios of cellular differentiation during bone repair.
Osteoblasts and adipocytes are the principal cells that constitute the microarchitecture of bone tissue. Commonly the adipocytes of the bone marrow and the osteoblasts are present in anatomically contiguous tissues and also derive from the same mesenchymal cell lineage. Whether adipogenesis or osteoneogenesis occurs is commonly associated with the activation of certain proteins and/or transcription factors, with one process suppressing the other. This constitutes the essential pathway by which cells commit to a specific differentiation lineage. The presence of these proteins detected by immunohistochemistry may identify a particular type of cell in bone development while the adipose differentiation pathways are inhibited, and vice versa.
Osteocalcin (OC) is a vitamin K-dependent osteoblast-specific protein and is commonly produced and expressed by mature osteoblasts. OC may also accumulate in the extracellular matrix produced by osteoblasts and has a high affinity for hydroxyapatite and mineral ions, properties that are considered determinants for the development and deposition of bone matrix.
Peroxisome proliferator-activated receptor gamma (PPAR-γ) is expressed in adipocytes; these receptors are responsible for the regulation of the expression of genes that control lipid metabolism, and at the same time inhibit the actions of proinflammatory genes. In addition, PPAR-γ inhibits osteoblastic differentiation and the expression of several anabolic mediators involved in bone formation, such as BMPs, type I collagen, and OC.
It was hypothesized that leucocyte- and platelet-rich plasma (L-PRP) could alter the histophenotype of bone repair, changing the proportions of osteoblasts and adipocytes. Thus the aim of this study was to evaluate the ratio of OC to PPAR-γ detected by immunohistochemistry, and to compare these results with a histomorphometric analysis of the bone matrix deposited in the presence and absence of L-PRP.
Materials and methods
Forty-two male rats ( Rattus norvegicus albinus, Wistar), 5–6 months old, weighing 450–500 g and with no previous disease were used in this study; the study protocol was approved by the institutional animal care and use committee. The rats were kept in a room with a controlled temperature (approximately 22 °C) and maintained under a 12-h light–dark cycle.
PRP fabrication and quantification
A 3.2-ml sample of autologous blood was collected from each animal through cardiac puncture, into a syringe containing 0.35 ml of 10% sodium citrate. The blood samples were centrifuged at 200 × g for 20 min at room temperature to separate the plasma containing the platelets from the red cells (Beckman J-6 M Induction Drive Centrifuge; Beckman Instruments Inc., Palo Alto, CA, USA). The plasma fraction was collected from the top of the supernatant. The remaining fractions were centrifuged once more at room temperature for 10 min at 400 × g to separate the platelets. The platelet-poor plasma was removed from the upper level of the supernatant, leaving the PRP and buffy coat. Both the buffy coat and PRP (0.35 ml) were resuspended and activated with a mixture of 10% calcium chloride (0.05 ml/ml of PRP). They were then added to the previously prepared L-PRP and mixed for approximately 1 min until they formed a gel.
The platelets and leukocytes in the L-PRP were measured after centrifugation using a Coulter STKS haematology counting machine (Beckman-Coulter, Chicago, IL, USA). The average whole blood platelet count was 617.66 ± 69.81 × 10 3 platelets/μl, while the average L-PRP platelet count was 2826.44 ± 309.12 × 10 3 platelets/μl ( P ≤ 0.001). The average concentration of leukocytes in the L-PRP was 8.14 ± 0.23 × 10 3 leukocytes/μl, while the average initial blood value was 3.18 ± 0.91 × 10 3 leukocytes/μl ( P ≤ 0.001).
Surgical procedure
The rats were anesthetized by intramuscular injection of xylazine (5 mg/kg) and ketamine (70 mg/kg). The surgical region was shaved and aseptically prepared, and sterile barriers were created to limit the surgical area. A 5-cm midline dermo-periosteal incision was made to expose the calvarial surface with complete removal of the periosteum. Circular artificial defects of 5 mm in diameter were created with a trephine (Biomedical Research Instruments Inc., Silver Spring, MD, USA) under abundant saline solution irrigation in each rat.
Bone fragments removed from the calvarial defects were particulated and used as autograft. In a randomized manner, one group received treatment with 0.01 ml of autograft and another group had the defect filled with 0.01 ml of autograft associated with 150 μl of L-PRP. The control animals (sham) underwent the same surgical procedures without the use of any grafting material. Soft tissues were repositioned and sutured to achieve primary closure (4-0 silk, Ethicon, Sao Paulo, SP, Brazil). Each animal received a prophylactic intramuscular injection of 24,000 IU of penicillin G benzathine and a daily dose of 200 mg/kg/day of liquid acetaminophen administrated orally.
Euthanasia procedure and tissue processing
At 15 or 40 days postsurgery, animals were euthanized by brief exposure in a CO 2 chamber until they ceased to move. The skull caps of each animal were removed in blocks using an inverted cone bur. Calvaria specimens obtained were fixed in 10% buffered formalin for 48 h and decalcified in 20% formic acid and sodium citrate for 7 days. The specimens were washed with tap water, dehydrated, cleared with xylene, and embedded in paraffin. Serial sections of 3 μm made parallel to the mid-sagittal suture were cut from the centre of each defect using a microtome (RM2155, Leica Microsystems GmbH, Nussloch, Germany) and stained with haematoxylin and eosin to permit histomorphological and histomorphometric evaluation.
Immunohistochemistry processing
Three-micrometre thicknesses of each specimen were deparaffinized and subjected to antigen retrieval in 1% trypsin solution (pH 1.8) for 60 min at 37 °C for the anti-OC and anti-PPAR-γ antibodies. Slides containing the histological fragments were immersed in 3% hydrogen peroxide for 30 min to eliminate endogenous peroxidase activity, followed by incubation with 1% phosphate-buffered saline (PBS; pH 7.4). The sections were incubated with the primary antibody anti-OC (200 μg/ml; Santa Cruz Biotechnology Inc., CA, USA) with a dilution factor of 1:200, and anti-PPAR-γ (200 μg/ml; Santa Cruz Biotechnology Inc., CA, USA) with a dilution factor of 1:150. A labelled streptavidin biotin antibody-binding detection system (Universal HRP Immunostaining Kit; Diagnostic BioSystems, Pleasanton, CA, USA) was employed to detect the primary antibodies. The immune reaction was observed with diaminobenzidine tetrachloride chromogen solution (Universal HRP Immunostaining Kit), which produces a brown precipitate at the antigen site. The specimens were counterstained with Harris haematoxylin. A negative control was included for all samples, using rabbit polyclonal isotype IgG (2 μg/ml, Abcam, Cambridge, UK) for 20 min at room temperature as a primary antibody. For each specimen, three slides were utilized for incubation with each antibody.
Image analysis
The images of both the histological and immunohistochemistry sections were captured by digital camera (SDC-310; Samsung, Gyeonggi-do, South Korea) coupled to a light microscope (Zeiss, Oberkochen, Germany) with an original magnification of 200×. Each digital image was gathered and preserved at 300 dpi resolution, producing a virtual frame of 113 cm × 80 cm. As it was not possible to capture the entire defect in one image at the magnification level used, a composite digital image of the whole defect was then constructed by combining five smaller images based on histomorphological reference structures, in particular the deposited bone trabeculae and blood vessels. All histomorphometric and immunohistochemical measurements were completed using the software Image J (National Institute of Health, Bethesda, MD, USA). A 1-mm calibration slide was used to calibrate all measurements. The histomorphometric data were compiled manually and expressed as areas (mm 2 ). The perimeters of the deposited histological bone matrix were carefully traced and the areas computed. At the same time, positive OC and PPAR-γ cells/mm 2 were manually counted and tagged.
Statistical analysis
Each parameter was evaluated within the monitoring period. Analysis of variance (ANOVA) was used to determine if there were significant differences among groups, followed by the Student–Newman–Keuls non-parametric test. A P -value of less than 0.05 was considered to be statistically significant.
Materials and methods
Forty-two male rats ( Rattus norvegicus albinus, Wistar), 5–6 months old, weighing 450–500 g and with no previous disease were used in this study; the study protocol was approved by the institutional animal care and use committee. The rats were kept in a room with a controlled temperature (approximately 22 °C) and maintained under a 12-h light–dark cycle.
PRP fabrication and quantification
A 3.2-ml sample of autologous blood was collected from each animal through cardiac puncture, into a syringe containing 0.35 ml of 10% sodium citrate. The blood samples were centrifuged at 200 × g for 20 min at room temperature to separate the plasma containing the platelets from the red cells (Beckman J-6 M Induction Drive Centrifuge; Beckman Instruments Inc., Palo Alto, CA, USA). The plasma fraction was collected from the top of the supernatant. The remaining fractions were centrifuged once more at room temperature for 10 min at 400 × g to separate the platelets. The platelet-poor plasma was removed from the upper level of the supernatant, leaving the PRP and buffy coat. Both the buffy coat and PRP (0.35 ml) were resuspended and activated with a mixture of 10% calcium chloride (0.05 ml/ml of PRP). They were then added to the previously prepared L-PRP and mixed for approximately 1 min until they formed a gel.
The platelets and leukocytes in the L-PRP were measured after centrifugation using a Coulter STKS haematology counting machine (Beckman-Coulter, Chicago, IL, USA). The average whole blood platelet count was 617.66 ± 69.81 × 10 3 platelets/μl, while the average L-PRP platelet count was 2826.44 ± 309.12 × 10 3 platelets/μl ( P ≤ 0.001). The average concentration of leukocytes in the L-PRP was 8.14 ± 0.23 × 10 3 leukocytes/μl, while the average initial blood value was 3.18 ± 0.91 × 10 3 leukocytes/μl ( P ≤ 0.001).
Surgical procedure
The rats were anesthetized by intramuscular injection of xylazine (5 mg/kg) and ketamine (70 mg/kg). The surgical region was shaved and aseptically prepared, and sterile barriers were created to limit the surgical area. A 5-cm midline dermo-periosteal incision was made to expose the calvarial surface with complete removal of the periosteum. Circular artificial defects of 5 mm in diameter were created with a trephine (Biomedical Research Instruments Inc., Silver Spring, MD, USA) under abundant saline solution irrigation in each rat.
Bone fragments removed from the calvarial defects were particulated and used as autograft. In a randomized manner, one group received treatment with 0.01 ml of autograft and another group had the defect filled with 0.01 ml of autograft associated with 150 μl of L-PRP. The control animals (sham) underwent the same surgical procedures without the use of any grafting material. Soft tissues were repositioned and sutured to achieve primary closure (4-0 silk, Ethicon, Sao Paulo, SP, Brazil). Each animal received a prophylactic intramuscular injection of 24,000 IU of penicillin G benzathine and a daily dose of 200 mg/kg/day of liquid acetaminophen administrated orally.
Euthanasia procedure and tissue processing
At 15 or 40 days postsurgery, animals were euthanized by brief exposure in a CO 2 chamber until they ceased to move. The skull caps of each animal were removed in blocks using an inverted cone bur. Calvaria specimens obtained were fixed in 10% buffered formalin for 48 h and decalcified in 20% formic acid and sodium citrate for 7 days. The specimens were washed with tap water, dehydrated, cleared with xylene, and embedded in paraffin. Serial sections of 3 μm made parallel to the mid-sagittal suture were cut from the centre of each defect using a microtome (RM2155, Leica Microsystems GmbH, Nussloch, Germany) and stained with haematoxylin and eosin to permit histomorphological and histomorphometric evaluation.
Immunohistochemistry processing
Three-micrometre thicknesses of each specimen were deparaffinized and subjected to antigen retrieval in 1% trypsin solution (pH 1.8) for 60 min at 37 °C for the anti-OC and anti-PPAR-γ antibodies. Slides containing the histological fragments were immersed in 3% hydrogen peroxide for 30 min to eliminate endogenous peroxidase activity, followed by incubation with 1% phosphate-buffered saline (PBS; pH 7.4). The sections were incubated with the primary antibody anti-OC (200 μg/ml; Santa Cruz Biotechnology Inc., CA, USA) with a dilution factor of 1:200, and anti-PPAR-γ (200 μg/ml; Santa Cruz Biotechnology Inc., CA, USA) with a dilution factor of 1:150. A labelled streptavidin biotin antibody-binding detection system (Universal HRP Immunostaining Kit; Diagnostic BioSystems, Pleasanton, CA, USA) was employed to detect the primary antibodies. The immune reaction was observed with diaminobenzidine tetrachloride chromogen solution (Universal HRP Immunostaining Kit), which produces a brown precipitate at the antigen site. The specimens were counterstained with Harris haematoxylin. A negative control was included for all samples, using rabbit polyclonal isotype IgG (2 μg/ml, Abcam, Cambridge, UK) for 20 min at room temperature as a primary antibody. For each specimen, three slides were utilized for incubation with each antibody.
Image analysis
The images of both the histological and immunohistochemistry sections were captured by digital camera (SDC-310; Samsung, Gyeonggi-do, South Korea) coupled to a light microscope (Zeiss, Oberkochen, Germany) with an original magnification of 200×. Each digital image was gathered and preserved at 300 dpi resolution, producing a virtual frame of 113 cm × 80 cm. As it was not possible to capture the entire defect in one image at the magnification level used, a composite digital image of the whole defect was then constructed by combining five smaller images based on histomorphological reference structures, in particular the deposited bone trabeculae and blood vessels. All histomorphometric and immunohistochemical measurements were completed using the software Image J (National Institute of Health, Bethesda, MD, USA). A 1-mm calibration slide was used to calibrate all measurements. The histomorphometric data were compiled manually and expressed as areas (mm 2 ). The perimeters of the deposited histological bone matrix were carefully traced and the areas computed. At the same time, positive OC and PPAR-γ cells/mm 2 were manually counted and tagged.
Statistical analysis
Each parameter was evaluated within the monitoring period. Analysis of variance (ANOVA) was used to determine if there were significant differences among groups, followed by the Student–Newman–Keuls non-parametric test. A P -value of less than 0.05 was considered to be statistically significant.
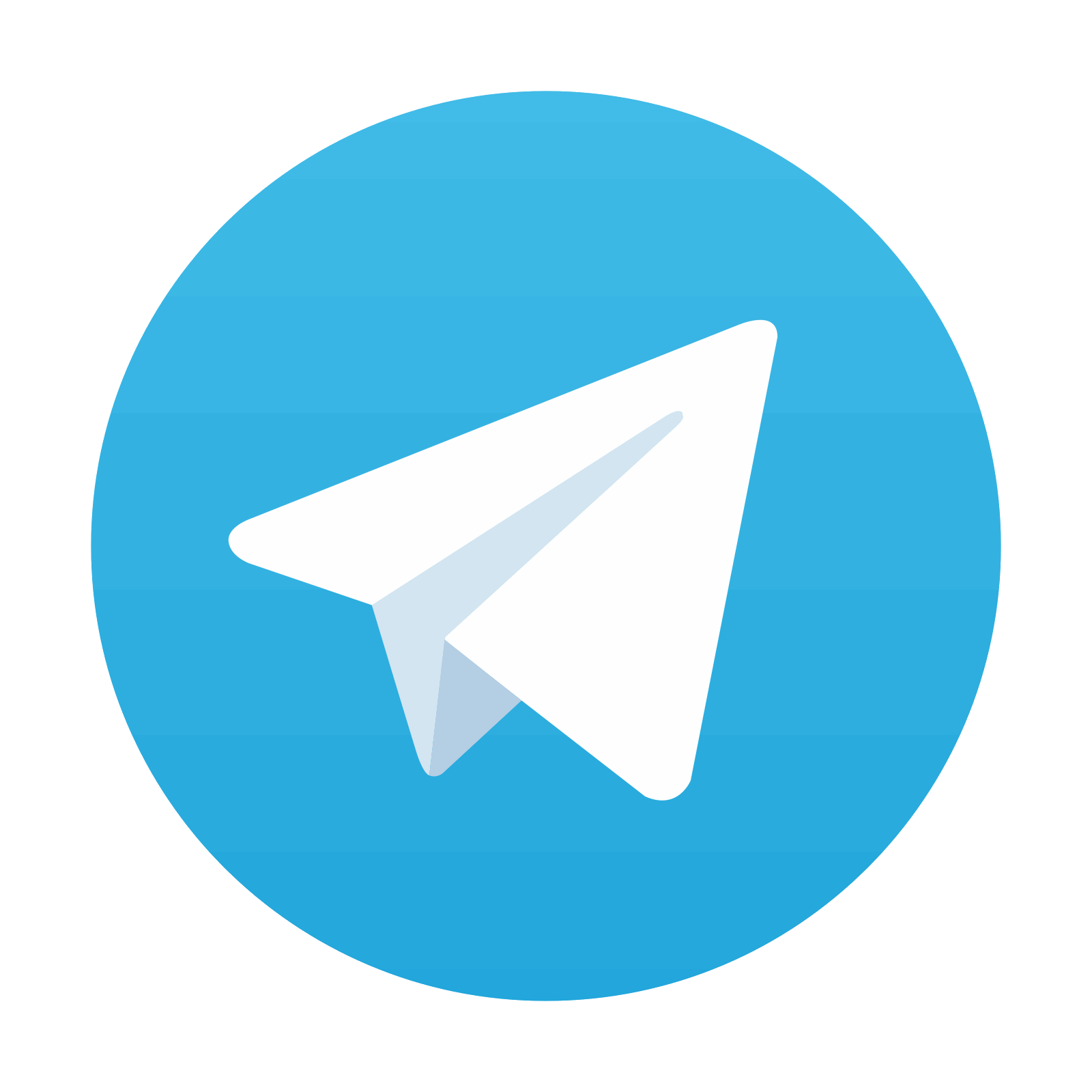
Stay updated, free dental videos. Join our Telegram channel

VIDEdental - Online dental courses
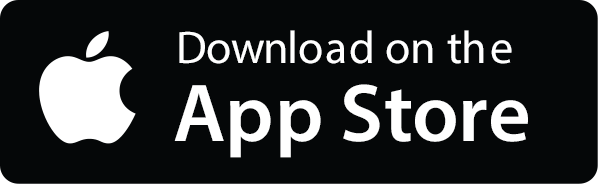
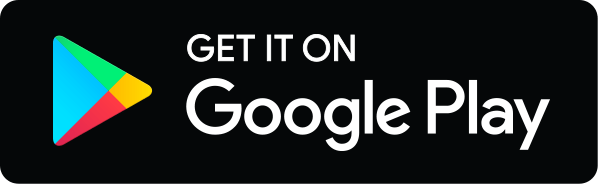