Abstract
Objective
Test the hypothesis that glass-bonding of free-form veneer and core ceramic layers can produce robust interfaces, chemically durable and esthetic in appearance and, above all, resistant to delamination.
Methods
Layers of independently produced porcelains (NobelRondo™ Press porcelain, Nobel BioCare AB and Sagkura Interaction porcelain, Elephant Dental) and matching alumina or zirconia core ceramics (Procera alumina, Nobel BioCare AB, BioZyram yttria stabilized tetragonal zirconia polycrystal, Cyrtina Dental) were joined with designed glasses, tailored to match thermal expansion coefficients of the components and free of toxic elements. Scanning electron microprobe analysis was used to characterize the chemistry of the joined interfaces, specifically to confirm interdiffusion of ions. Vickers indentations were used to drive controlled corner cracks into the glass interlayers to evaluate the toughness of the interfaces.
Results
The glass-bonded interfaces were found to have robust integrity relative to interfaces fused without glass, or those fused with a resin-based adhesive.
Significance
The structural integrity of the interfaces between porcelain veneers and alumina or zirconia cores is a critical factor in the longevity of all-ceramic dental crowns and fixed dental prostheses.
1
Introduction
Dental crowns and bridges are generally laminar structures, with complex geometries . Traditionally, an esthetic porcelain is veneered onto a strong core material layer by layer. In the case of all-ceramic restorations, the core material is usually alumina or (more recently) zirconia. Alumina crowns tend to fail by bulk fracture initiating at the cementation surface . Zirconia is considerably stronger so that failure is more likely to occur in the porcelain overlayer, by chipping or by spallation from the core – the integrity of the structure then rests with the relative toughness of the porcelain overlayer and the veneer/core interface. While successful, current veneering techniques are painstakingly time consuming and expensive, and the interfacial bonding is often less than perfect. With the move toward increased automation in the dental industry, there is room for the introduction of novel and economical procedures.
A potential alternative fabrication route is free-form processing, in which each layer is shaped individually, e.g. by robocasting or computer aided manufacturing , and then fused together. It is possible to join two components by fusion at elevated temperature, but for all-ceramic components the required temperatures are very high . Also, the interfaces do not always wet or fuse perfectly, leaving weaknesses that can act as sources of failures. Conversely, one might use a polymeric resin to bond the components at room temperature, but the resultant interface is then relatively weak and subject to chemical degradation . A reasonable compromise is a glass bond. Glass joining is a long established technology , widely used in vacuum seals for metal components, electronic packaging, fuel cells and coating applications . Principal requirements for a successful join are: matching coefficients of thermal expansion (CTE, typically within 1 × 10 −6 /°C) to minimize residual stresses; good wetting and interlayer diffusion, to effect a strong bond; low firing temperature, to minimize any degradation of the veneer layer during processing; and bioinertness, to avoid adverse chemical degradation in a reactive environment. In the case of dental joins, the joining glass should be lead- and bismuth-free, to avoid toxicity concerns.
In this study we describe a procedure for using lead-free glasses to fuse free-standing porcelain layers to alumina and zirconia core layers. As indicated, in all-ceramic systems both veneer and core layers are vulnerable to fracture, most generally to cracks that traverse the layer thickness – so-called ‘transverse’ cracks. More importantly, the interlayer between the two layers is itself vulnerable. Can the glass bond prevent cracks in the veneer layer from penetrating into the core, or vice versa? Above all, can the glass interlayer prevent veneer cracks from delaminating the interface? Our procedure was to select commercial porcelain and core alumina and zirconia plates, and to design and custom-fabricate matching glasses for bonding the layers with good wetting, low porosity and minimum CTE mismatch stress. Electron microprobe analysis was then used to characterize interdiffusion chemistry at the interfaces and simple mechanical testing with a Vickers indenter applied to characterize the bond integrity.
2
Materials and methods
Since we were concerned here only with the joining process itself, experiments were confined to flat layers, mindful that the techniques will ultimately need to be applicable to more complex geometrical shapes. The procedure was to choose commercially matched porcelain and core material plates and to design and custom-fabricate glasses for bonding the layers with minimum CTE mismatch. Two dental material systems were selected for study. The first was Procera alumina (Nobel BioCare AB, Stockholm, Sweden) with CTE-matching NobelRondo™ Press porcelain (Nobel BioCare AB, Stockholm, Sweden). The second was Cyrtina Dental yttria stabilized tetragonal zirconia polycrystal (Biozyram, Cyrtina Dental, Netherlands) with CTE-matching Sagkura Interaction porcelain (Elephant Dental, The Netherlands). The alumina and zirconia core materials were obtained as blocks and cut and ground into plates with sides 10 mm and thickness 0.7 mm. The porcelains were received as powders, which were mixed into water-based slurries and poured into refractory molds with 7 mm sides. After drying, the molds were vacuum-fired to a peak temperature of 850 °C over a 20 min heating–cooling cycle, according to manufacturer specifications. The as-fired porcelain specimens were then ground to 1 mm thickness. The surfaces of both core and veneer plates were polished flat and parallel to 1 μm finish using diamond paste, followed in the case of zirconia with colloidal silica suspension to remove any strain-induced transformations. Essential properties of the alumina, zirconia and porcelain components are shown in Table 1 , using data obtained from manufacturer’s specifications and routine in-house materials characterization (see below).
Alumina system | Function | Supplier | Modulus, E (GPa) | Hardness, H (GPa) | Toughness, T (MPa m 1/2 ) | CTE (10 −6 /°C) |
---|---|---|---|---|---|---|
Procera | Core | Nobel Biocare | 370 | 13.5 | 3.1 | 7.15 |
Rondo | Veneer | Nobel Biocare | 65 | 6.4 | 0.8 | 7.20 |
Zirconia system | Function | Supplier | Modulus, E (GPa) | Hardness, H (GPa) | Toughness, T (MPa m 1/2 ) | CTE (10 −6 /°C) |
---|---|---|---|---|---|---|
BioZyram | Core | Cytina Dental | 205 | 12.0 | 5.3 | 10.5 |
Sakura Interaction | Veneer | Elephant Dental | 65 | 6.2 | 0.8 | 9.9 |
The choice of glass compositions for bonding core and veneer layers was less straightforward. Several commercially available lead-free glasses were investigated, but none matched our selected dental ceramics sufficiently to produce suitable quality joins. Accordingly, we used an in-house sol–gel wet chemistry fabrication route to produce custom non-toxic glass compositions with CTE values matching those listed in Table 1 for each veneer/core bilayer combination. The methodology used glass components with documented properties and rule-of-mixtures formulae to obtain compositions with requisite CTE, elastic modulus and softening temperatures. Details of this selection process are described elsewhere . The tailored glass compositions and ensuing physical properties used here are shown in Table 2 for each veneer–core system. Dilatometery (Orton DIL 2016 STD, Westerville, OH) was used to determine CTE values for each glass.
System | SiO 2 (mol%) | Al 2 O 3 (mol%) | CaO (mol%) | Na 2 O (mol%) | BaO (mol%) | B 2 O 3 (mol%) | CTE (10 −6 /°C) |
---|---|---|---|---|---|---|---|
Alumina system | 60 | 3.03 | 8.37 | 6.41 | 6.05 | 16.14 | 7.0 |
Zirconia system | 60 | 3.13 | 9.4 | 14.64 | 6.56 | 6.27 | 10.4 |
The glasses were fined at 1200 °C then quenched into shards and ground into frit with maximum particle size of 38 μm, a upper limit for ensuing firing without inducing residual porosity in the glass. The frits were then mixed into 50/50 vol.% water/ethanol slurries. The slurries were applied evenly to the top surfaces of each of the alumina and zirconia core and matching porcelain veneer plates. In this way coating layers 50–100 μm thick were built up. To wet the glasses onto the surfaces, a prefiring cycle was conducted for each specimen layer at a rate of 30 °C/min, with a 5 min hold at 750 °C for the alumina core and veneer layers and 700 °C for the zirconia layers. The specimens were then slowly cooled within the furnace to avoid crazing or porosity in the coating.
The coated surfaces of each core and veneer combination were then brought into mutual contact and vacuum heated at 40 °C/min under light pressure to 830 °C for the alumina system and 800 °C for the zirconia system, at a common hold time 30 min. These temperatures were sufficient to fuse the veneers to the cores without compromising the shape of the composite structures. Prescribed hold times of 30 min at peak temperature were examined. Comparative tests at 15 min and 45 min hold times showed little difference in the final results.
The fired specimens were sectioned with a diamond saw, followed by grinding and polishing to 1 μm finish, in order to view the bonded interface by optical microscopy. All the specimens survived the cutting and finishing processes, providing a useful initial screening test for bond integrity. Uniformity of thickness and lack of porosity were taken as further indicators of a ‘good’ interface.
Wavelength dispersive spectroscopy (JEOL JXA-8900 SuperProbe, Japan Electron Optics, Tokyo, Japan) with a 1 μm-wide electron beam was used to examine ion diffusion between the bonding glass and the ceramic sandwich layers. Intensity readings were taken every 1.5–2.8 μm and recorded as profiles across the interface. Special attention was given to mobile K + ions, since these ions were originally present in the porcelains but absent in the glasses, thus providing a definitive marker for interdiffusion.
To explore bond toughness, Vickers indentations were placed across the sections at various prescribed distances from the bonding interfaces in each of the porcelain/alumina and porcelain/zirconia layer systems using a microindentation tester (Zwick, Riverview, MI, USA). Some indents were made in separate alumina, zirconia and porcelain plates as comparative controls. Different loads were used in the various layer materials, to maintain indentations with well-defined cracks without chipping: in the porcelains, P = 10 N; in alumina, P = 10 N; in zirconia, P = 40 N. In the layer structures, corner cracks were aligned parallel and perpendicular to the glass interface, so that the lead transverse crack (at the corner closest to the interface) may intersect the interface itself. For each indent, a high power microscope was used to measure the lead arm crack size c measured from the indent center and tip location x measured relative to the glass/core interface.
2
Materials and methods
Since we were concerned here only with the joining process itself, experiments were confined to flat layers, mindful that the techniques will ultimately need to be applicable to more complex geometrical shapes. The procedure was to choose commercially matched porcelain and core material plates and to design and custom-fabricate glasses for bonding the layers with minimum CTE mismatch. Two dental material systems were selected for study. The first was Procera alumina (Nobel BioCare AB, Stockholm, Sweden) with CTE-matching NobelRondo™ Press porcelain (Nobel BioCare AB, Stockholm, Sweden). The second was Cyrtina Dental yttria stabilized tetragonal zirconia polycrystal (Biozyram, Cyrtina Dental, Netherlands) with CTE-matching Sagkura Interaction porcelain (Elephant Dental, The Netherlands). The alumina and zirconia core materials were obtained as blocks and cut and ground into plates with sides 10 mm and thickness 0.7 mm. The porcelains were received as powders, which were mixed into water-based slurries and poured into refractory molds with 7 mm sides. After drying, the molds were vacuum-fired to a peak temperature of 850 °C over a 20 min heating–cooling cycle, according to manufacturer specifications. The as-fired porcelain specimens were then ground to 1 mm thickness. The surfaces of both core and veneer plates were polished flat and parallel to 1 μm finish using diamond paste, followed in the case of zirconia with colloidal silica suspension to remove any strain-induced transformations. Essential properties of the alumina, zirconia and porcelain components are shown in Table 1 , using data obtained from manufacturer’s specifications and routine in-house materials characterization (see below).
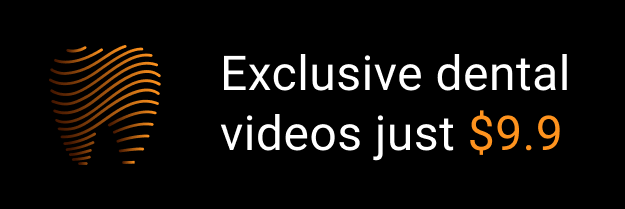