Abstract
Objectives
The aim of this study was to investigate the effectiveness of a variety of techniques to bond new composite to artificially aged composite of different compositions.
Methods
Composite resin blocks were made of five different commercially available composites ( n = 30) (Clearfil AP-X, Clearfil PhotoPosterior, Photo Clearfil Bright, Filtek Supreme XT and HelioMolar). After aging the composite blocks (thermo-cycling 5000×), blocks were subjected to one of 9 repair procedures: no treatment (control), diamond bur, sandblasting alumina particles, CoJet™, phosphoric acid, 3% hydrofluoric acid 20 s or 120 s, 9.6% hydrofluoric acid 20 s or 120 s. In addition, the cohesive strength of the tested composites was measured. Two-phase sandwiches (‘repaired composite’) were prepared using each of the 9 repair protocols, successively followed by silane and adhesive (OptiBond FL) treatment, prior to the application of the same composite. Specimens were subjected to micro-tensile bond strength testing. Data were analyzed using ANOVA and Tukey’s HSD ( p < 0.05).
Results
For all composites the lowest bond strength was obtained when no specific repair protocol (control) was applied; the highest for the cohesive strength. Compared to the control for the microhybrid composite (Clearfil AP-X) five repair techniques resulted in a significantly higher repair strength ( p < 0.05), whereas for the nano-hybrid composite (Filtek Supreme XT) and hybrid composite containing quartz (Clearfil PhotoPosterior) only one repair technique significantly increased the bond strength ( p < 0.01).
Significance
None of the surface treatments can be recommended as a universally applicable repair technique for the different sorts of composites. To optimally repair composites, knowledge of the composition is helpful.
1
Introduction
With the introduction of dental adhesive technology, minimally invasive tooth-restoration techniques became popular . Complete replacement of a deficient dental restoration results in further extension of the preparation , and therefore repair by partial replacement of the restoration or local extension adjacent to the existing restoration is recommended . In such cases, providing sufficient attachment to the old restoration is important, which can be achieved macro-mechanically, micro-mechanically or chemically. Macro-mechanical retention can be obtained by retention holes, undercuts or by simply roughening the surface with a coarse diamond bur . Micro-mechanical retention can be created by partially dissolving glass by breaking down the Si–O bond in SiO 2 , the major component of most glass-filler particles in dental composites, by etching with hydrofluoric acid . As an alternative method, sandblasting or air-abrasion can be performed with aluminum oxide powder . Finally, a chemical bond may be established between resin and silica glass filler particles by application of a silane coupling agent .
As the composition of composites may differ among brands of materials, it is likely that these different materials may react differently to various repair techniques. Until now, an optimal universally applicable technique to repair various types of composite restorations has not been described. Therefore, the aim of this study was to investigate the effectiveness of a variety of repair techniques to bond composite to artificially aged composite, of different compositions. The first hypothesis tested was that there was no difference in bond strength for the different repair treatments. The second hypothesis was that all types of used composites would react in the same way to various repair techniques.
2
Materials and methods
2.1
Specimen preparation
Standardized composite blocks were made using a silicon mold with a dimension of 7 mm × 7 mm × 5 mm ( Fig. 1 a ). Composite was injected into the mold in two horizontal layers of 2.5 mm each. The first layer was applied and polymerized for 20 s with a LED polymerization unit (LEDemetron I, Kerr, Orange, CA, USA; light intensity 600 mW/cm 2 ). Subsequently, the second layer was applied and a glass plate was pressed on top of the mold to create a flat superficial layer. With the glass plate in situ the composite was polymerized for 20 s. After polymerization, the composite block was removed from the mold, and post-cured from different directions for 120 s in a halogen-light curing unit (Unilux, Hereaus Kulzer, Hanau, Germany). The top surface was then polished for 15 s with a 600-grit wet silicon carbide abrasive paper, using running water as lubricant. All blocks were subjected to an aging procedure by thermo-cycling (5000 cycles, between 5 and 55 °C, dwell time: 30 s) . Subsequently, the blocks were ultrasonically cleaned for 15 min in distilled water and finally stored in distilled water at room temperature for two weeks.
In addition, separate solid composite blocks ( n = 3/composite material) were made to determine the cohesive strength (=positive control) of each composite. These blocks were not aged and no surface treatment procedure was applied.
Five composites were selected for this study, varying in composition and content of the filler ( n = 27/group):
- (a)
Micro-hybrid composite heavily filled with fine barium silica glass particles (Clearfil AP-X, Kuraray Medical, Osaka, Japan).
- (b)
Hybrid composite heavily filled with quartz particles (Clearfil PhotoPosterior, Kuraray Medical, Osaka, Japan).
- (c)
Hybrid composite containing quartz, silicium oxide and pre-polymerized particles on basis of silicium oxide (Photo Clearfil Bright, Kuraray Medical, Osaka, Japan).
- (d)
Nano-hybrid composite containing zirconium oxide and silicium oxide (Filtek Supreme XT, 3M ESPE Dental Products, Seefeld, Germany)
- (e)
Micro-filled composite containing barium and silica (HelioMolar, Ivoclar Vivadent, Schaan, Liechtenstein).
2.2
Repair technique
Three blocks of each group were subjected to one of 9 different surface treatments:
- 1.
No surface treatment (=negative control).
- 2.
Roughening the surface with a long course diamond bur (grit: 100 μm, Komet, Lemgo, Germany) with 200,000 rpm, perpendicular to the surface of the surface.
- 3.
Sandblasting with 50 μm Al 2 O 3 powder for 20 s at a distance of 15 mm (MicroEtcher, Danville Engineering, San Ramon, USA) with a pressure of 2.5 bar.
- 4.
Sandblasting with 30 μm SiO 2 powder for 20 s at a distance of 15 mm (MicroEtcher and CoJet™ powder, 3 M ESPE, Seefeld, Germany) with a pressure of 2.5 bar.
- 5.
Etching with 37% phosphoric acid for 20 s (DMG, Hamburg, Germany).
- 6.
Etching with 3% hydrofluoric acid for 20 s (Porcelock Porcelain etching, DenMat, Santa Maria, CA, USA).
- 7.
Etching with 3% hydrofluoric acid for 120 s (Porcelock, DenMat).
- 8.
Etching with 9.6% hydrofluoric acid for 20 s (Porcelain etch gel, Pulpdent Co., Watertown, USA).
- 9.
Etching with 9.6% hydrofluoric acid for 120 s (Porcelain etch gel, Pulpdent).
Table 1 summarizes the product profiles, material properties and LOT-numbers of the composites selected.
Materials | Manufacturers | Composition | LOT |
---|---|---|---|
Clearfil AP-X (shade A2 + A4) | Kuraray Medical, Osaka, Japan | 70 vol% barium glass filler | Shade A2: 0257A Shade A4: 449AA |
Clearfil PhotoPosterior (shade US + B) | Kuraray Medical, Osaka, Japan | 71 vol% quartz filler | Shade US: 0021GB Shade B: 113IA |
Photo Clearfil Bright (shade US + B) | Kuraray Medical, Osaka, Japan | 72 vol% quartz and silicon dioxide and pre-polymerized particles containing silicon dioxide | Shade US: 051BA Shade B: 025IA |
HelioMolar (shade A2 + A4) | Ivoclar Vivadent, Schaan, Liechtenstein | 46 vol% silicon dioxide, ytterbium trifluoride and copolymer | Shade A2: J25067 Shade A4: H07720 |
Filtek Supreme XT (shade A2B + A4B) | 3M ESPE Dental products, Seefeld, Germany | 58 vol% aggregated zirconia/silica cluster filler and nonagglomerated/nonaggregated silica filler | Shade A2B: 20070926 Shade A4B: 20071129 |
Silane Primer | Kerr, Orange, CA, USA | 3 wt% Silane; 15 wt% Resin monomers (BisGMA, TEGDM, EBPADMA); 82 wt% Ethanol; <1% additives and iniators | 2748078 |
OptiBond FL | Kerr, Orange, CA, USA | Adhesive: Bis-GMA, HEMA, GDMA, CQ, ODMAB, Filler (fumed SiO 2 , barium aluminoborosilicate, Na 2 SiF 6 ), coupling factor A174 (approximately 48 wt% filled) | Adhesive: 2880482 |
Diamond bur | Komet, Lemgo, Germany | Grit: 100 μm | 83,73,14,014 |
Aluminum Oxide powder | Rønvig, Dental mfg. A/S. Daugaard, Denmark | 50 μm Aluminum Oxide | – |
CoJet powder | 3M ESPE Dental products, Seefeld, Germany | 30 μm Silicated SiO 2 | 152745 |
Etching gel | DMG, Hamburg, Germany | 37% Phosphoric acid | 630485 |
Porcelock Porcelain Etching Solution | DenMat, Santa Maria, CA, USA | 3% Hydrofluoric acid | HO95010055 |
Porcelain Etch Gel | Pulpdent Co., Watertown, USA | 9.6% Hydrofluoric acid | IA061130 |
After surface treatment in all groups, a silane solution (Kerr, Orange, CA, USA) was applied on top of the composite block for 15 s and gently air-dried. Then, the adhesive resin (OptiBond FL, Kerr), without dentin primer, was applied, gently air-thinned and cured for 20 s. Subsequently, a silicon mold of 7 mm × 7 mm × 9 mm was used to standardize the insertion of 4 mm of fresh composite resin material to the aged and pre-treated composite block ( Fig. 1 c). Each specimen was repaired with the same brand of composite of a clearly distinguished shade. The composite was added in two horizontal layers and light-cured for 20 s per layer. Then, the mold was removed and samples were post-cured for 20 s from all four lateral sides.
2.3
μTBS measurement
After one week water-storage, out of each block four specimens of 1.8 mm × 1.8 mm × 8 mm were prepared for micro-tensile bond strength (μTBS) testing. Specimens were sectioned perpendicular to the bonding surface using a slow-speed diamond saw (Isomet 1000, Buehler Ltd, Lake Bluff, IL, USA) under continuous water cooling ( Fig. 1 d). The four central specimens were mounted in the pin-chuck of the MicroSpecimen Former and trimmed at the composite–composite interface into an hour-glass shape with a bonding surface of approximately 1 mm 2 using a cylindrical fine-grit diamond bur (5835KREF, Komet, Lemgo, Germany) in a high-speed turbine under air/water coolant ( Fig. 1 e). Specimens were then fixed to a Ciucchi’s jig with a cyanoacrylate glue (Model Repair II Blue, Dentsply-Sankin, Ohtawara, Japan) and stressed at a crosshead speed of 1 mm/min until failure using a universal testing device (LRX; Lloyd, Hampshire, UK) with a load cell of 100 N ( Fig. 1 f). μTBS is expressed in MPa as derived from dividing the applied force (N) at the time of fracture by the bond area (mm 2 ). The occurrence of failure prior to the actual testing was included in the calculation of the mean μTBS as 0 MPa with an explicit note of the number of pre-testing failures (ptf). Moreover, the highest and the lowest value in each group were excluded.
2.4
Failure mode
The mode of failure was determined at a magnification of 50× using the stereomicroscope (Wild M5A) and recorded as ‘cohesive in aged composite’, ‘adhesive at interface’ (interfacial), ‘cohesive in new composite’ (including failures within the adhesive layer and/or composite) or ‘mixed adhesive-cohesive’. The presence of porosities or voids are present in the interface, this will be scored separately.
2.5
Statistical analysis
One-way analysis of variance (ANOVA) and post hoc Tukey’s HSD multiple comparisons were used to determine statistical differences in μTBS within groups at a significance level of 0.05.
2
Materials and methods
2.1
Specimen preparation
Standardized composite blocks were made using a silicon mold with a dimension of 7 mm × 7 mm × 5 mm ( Fig. 1 a ). Composite was injected into the mold in two horizontal layers of 2.5 mm each. The first layer was applied and polymerized for 20 s with a LED polymerization unit (LEDemetron I, Kerr, Orange, CA, USA; light intensity 600 mW/cm 2 ). Subsequently, the second layer was applied and a glass plate was pressed on top of the mold to create a flat superficial layer. With the glass plate in situ the composite was polymerized for 20 s. After polymerization, the composite block was removed from the mold, and post-cured from different directions for 120 s in a halogen-light curing unit (Unilux, Hereaus Kulzer, Hanau, Germany). The top surface was then polished for 15 s with a 600-grit wet silicon carbide abrasive paper, using running water as lubricant. All blocks were subjected to an aging procedure by thermo-cycling (5000 cycles, between 5 and 55 °C, dwell time: 30 s) . Subsequently, the blocks were ultrasonically cleaned for 15 min in distilled water and finally stored in distilled water at room temperature for two weeks.
In addition, separate solid composite blocks ( n = 3/composite material) were made to determine the cohesive strength (=positive control) of each composite. These blocks were not aged and no surface treatment procedure was applied.
Five composites were selected for this study, varying in composition and content of the filler ( n = 27/group):
- (a)
Micro-hybrid composite heavily filled with fine barium silica glass particles (Clearfil AP-X, Kuraray Medical, Osaka, Japan).
- (b)
Hybrid composite heavily filled with quartz particles (Clearfil PhotoPosterior, Kuraray Medical, Osaka, Japan).
- (c)
Hybrid composite containing quartz, silicium oxide and pre-polymerized particles on basis of silicium oxide (Photo Clearfil Bright, Kuraray Medical, Osaka, Japan).
- (d)
Nano-hybrid composite containing zirconium oxide and silicium oxide (Filtek Supreme XT, 3M ESPE Dental Products, Seefeld, Germany)
- (e)
Micro-filled composite containing barium and silica (HelioMolar, Ivoclar Vivadent, Schaan, Liechtenstein).
2.2
Repair technique
Three blocks of each group were subjected to one of 9 different surface treatments:
- 1.
No surface treatment (=negative control).
- 2.
Roughening the surface with a long course diamond bur (grit: 100 μm, Komet, Lemgo, Germany) with 200,000 rpm, perpendicular to the surface of the surface.
- 3.
Sandblasting with 50 μm Al 2 O 3 powder for 20 s at a distance of 15 mm (MicroEtcher, Danville Engineering, San Ramon, USA) with a pressure of 2.5 bar.
- 4.
Sandblasting with 30 μm SiO 2 powder for 20 s at a distance of 15 mm (MicroEtcher and CoJet™ powder, 3 M ESPE, Seefeld, Germany) with a pressure of 2.5 bar.
- 5.
Etching with 37% phosphoric acid for 20 s (DMG, Hamburg, Germany).
- 6.
Etching with 3% hydrofluoric acid for 20 s (Porcelock Porcelain etching, DenMat, Santa Maria, CA, USA).
- 7.
Etching with 3% hydrofluoric acid for 120 s (Porcelock, DenMat).
- 8.
Etching with 9.6% hydrofluoric acid for 20 s (Porcelain etch gel, Pulpdent Co., Watertown, USA).
- 9.
Etching with 9.6% hydrofluoric acid for 120 s (Porcelain etch gel, Pulpdent).
Table 1 summarizes the product profiles, material properties and LOT-numbers of the composites selected.
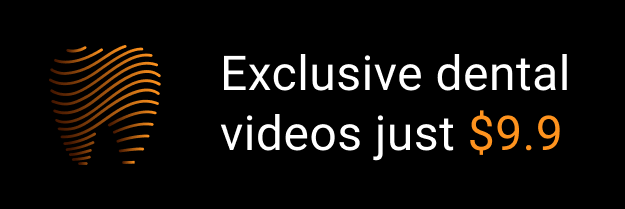