Abstract
Objective
The objective of this study was to test the null hypothesis that the interfacial toughness of each of two types of bonded core-veneer bilayer ceramics is not significantly different from the apparent fracture toughness of the control monolithic glass veneer.
Methods
T-shaped short-bars of a lithia-disilicate glass–ceramic core (LC) and yttria-stabilized polycrystalline zirconia core ceramic (ZC) were prepared according to the manufacturer’s recommendations. V-shaped notches were prepared by using 25-μm-thick palladium foil, leaving the chevron-notch area exposed, and the bars were veneered with a thermally compatible glass veneer (LC/GV and ZC/GV). Additionally, we also bonded the glass veneer to itself as a control group (GV/GV). Specimens were kept in distilled water for 30 days before testing in tension. Eight glass veneer bars were prepared for the analysis of fracture toughness test using the indentation-strength technique.
Results
The mean interfacial toughness of the LC/GV group was 0.69 MPa m 1/2 (0.11), and did not significantly differ from that of the GV/GV control group, 0.74 MPa m 1/2 (0.17) ( p > 0.05). However, the difference between the mean interfacial toughness of the ZC/GV group, 0.13 MPa m 1/2 (0.07), and the LC/GV and the GV/GV groups was statistically significant ( p < 0.05).
Significance
For bilayer all-ceramic restorations with high-strength core materials, the veneering ceramics are the weakest link in the design of the structure. Since all-ceramic restorations often fail from chipping of veneer layers or crack initiation at the interface, the protective effects of thermal mismatch stresses oral prosthesis design should be investigated.
1
Introduction
All-ceramic restorations are desired by patients and dentists because of their esthetics and biocompatibility. Recently, new dental ceramic core systems with high strength and high toughness were introduced as alternatives to metals. However, chipping and fracture of veneer layers have been reported as the failure of some all-ceramic systems , which raises uncertainty on the benefits of using these all-ceramic prostheses.
Glass–ceramics are polycrystalline materials, whose crystallization by nucleation and crystal growth are controlled by special heat treatment . Because of the small amount of glass phase, these materials tend to be relatively pore-free. Recently, two glass–ceramics containing 70 vol% of elongated lithia-disilicate crystals have been introduced as core ceramics (Empress ® 2 and e.max ® Press, Ivoclar-Vivadent, Schaan, Liechtenstein). Ceramic restorations that are made from glass–ceramics are more translucent than purely crystalline ceramics because of their glassy phases. However, because of their low fracture toughness, these systems are limited to three-unit fixed partial dentures that replace a missing tooth anterior to the second premolar .
Polycrystalline dental ceramics such as alumina and zirconia have no glassy phase, and they are relatively opaque compared with ceramics that contain a glass phase. Therefore, these stronger and tougher materials are used as substructures (cores) to support weaker but more translucent glass veneers. The ceramics in these systems include a densely sintered high-purity alumina and partially stabilized zirconia that can be milled to dense anatomic shapes by a CAD-CAM device in either partially sintered or fully dense form .
A shear test is frequently used to measure the bond strength between two bonded substrates, from which the stress at failure is determined. Shear bond test analyses ignore the stress distribution generated within the adherence zone, which can have a significant effect on the mode of failure. Moreover, the loading device for the shear test typically makes contact at a slight distance from the interface because of a small radius of curvature at the edge of the loading blade or wire . This causes flexural failure rather than the desired shear failure. Flexural failure can lead to the false interpretation of the resultant bond strength data.
Chevron-notch specimens have been developed for testing the fracture toughness of ceramics, high-strength metals, and other brittle materials . Barker proposed short-rod and short-bar designs for fracture toughness tests . These specimens have advantages over traditional fracture toughness specimens. They are relatively small, simple, and inexpensive. Precracks are not required because there are extremely high stress concentrations at the tip of the chevron-notch. Thus, cracks can be initiated at a low applied load in a stable manner. Fracture toughness can be evaluated from the maximum load. Consequently, a load–displacement record is not required and measurement of crack length is not necessary .
The primary advantage of the short-bar fracture toughness test is that a precrack is not needed. Other advantages include the small sample dimension, the dependence of fracture toughness on the peak load alone, freedom from measuring the crack length, and the applicability to both ductile and brittle materials. Short-rod and short-bar designs have been used to test the toughness of bonded interfaces between luting cements and dentin and between resin composite and dentin . However, there are no published studies that have been based on the use of the chevron-notch design for testing of bilayer, dental ceramics.
Mecholsky and Barker developed a method of using the chevron-notch specimens to measure the interfacial toughness of ceramic–metal interfaces. To ensure equal compliances for the two halves of the specimens with different elastic moduli, they assumed that, E 1 I 1 = E 2 I 2 where E 1 I 1 and E 2 I 2 are the product of the elastic modulus and moment of inertia of materials 1 and 2, respectively. Values of d 1 and d 2 are chosen such that:
E 1 d 1 3 = E 2 d 2 3
where d is the thickness of the short-bar. The compliances of both halves will be approximately the same ( Fig. 1 a) .

The interfacial toughness can be derived from the equation:
K c = Y m P max B W 1 / 2
where K c is the fracture toughness; Y m is the minimum stress intensity coefficient; P max is the maximum load to failure; B is the width of the specimen; W is the length of the specimen.
The objective of the present study was to determine the interfacial fracture toughness of two types of bilayer dental ceramics using the short-bar chevron-notch test and to test the null hypothesis that the interfacial toughness of core-veneer bonded bilayers do not differ significantly from the fracture toughness of the monolithic veneer ceramic.
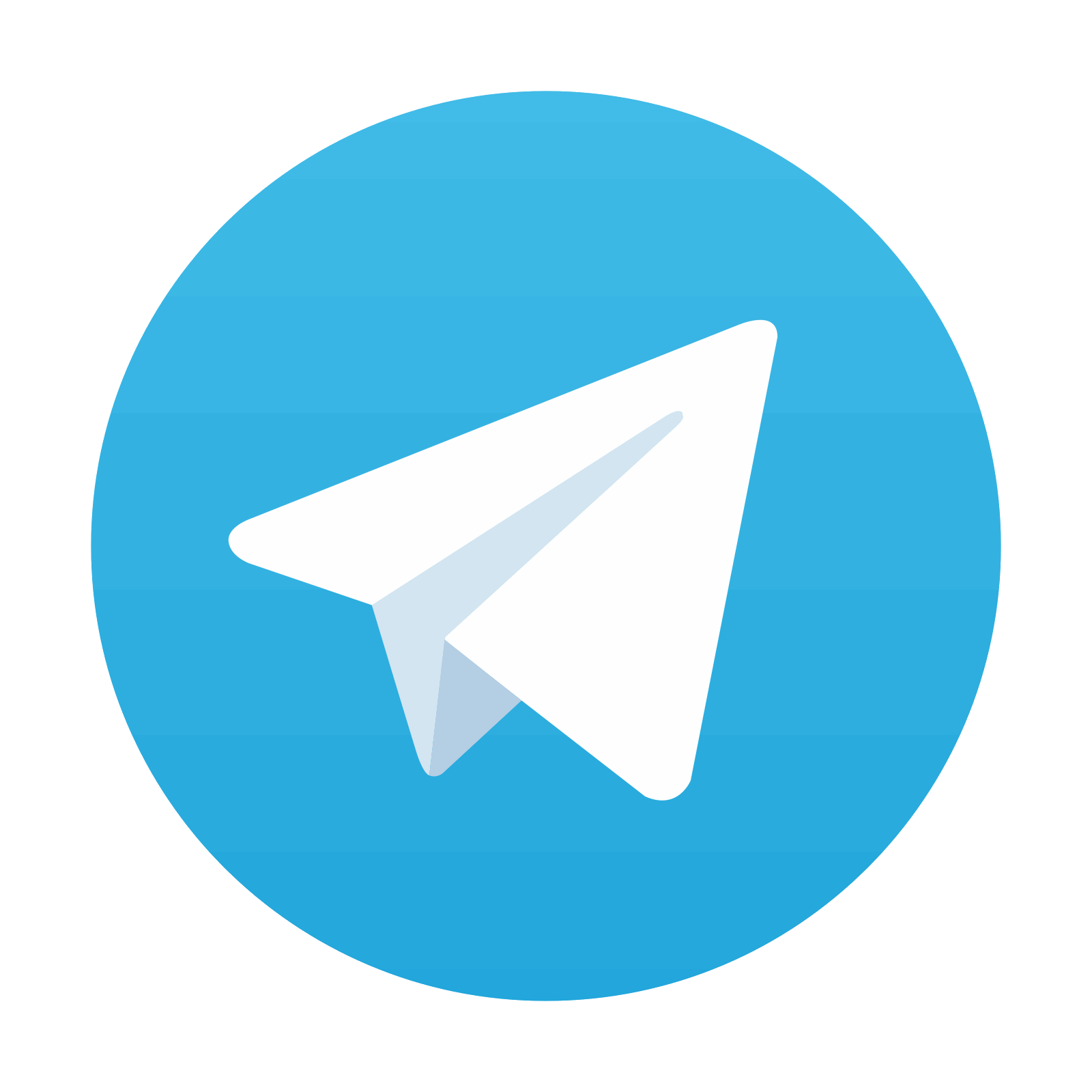
Stay updated, free dental videos. Join our Telegram channel

VIDEdental - Online dental courses
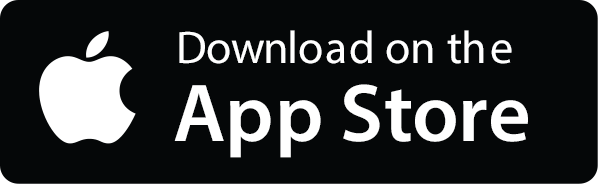
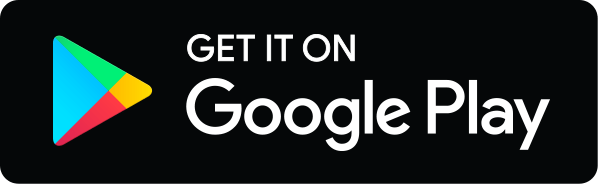