Abstract
Objectives
To examine the effect of ultraviolet light (UV) treatment on the surface characteristics of two acid-etched zirconia-based dental implant materials.
Methods
Discs of two zirconia-based materials (Zr1 and Zr2) with smooth (m) and roughened (r) surfaces were treated by UV light for 15 min. The surface topography was investigated by scanning electron microscopy (SEM) and atomic force microscopy (AFM). The surface elemental composition of all samples was determined by X-ray photoelectron spectroscopy (XPS), the crystalline property by X-ray diffraction (XRD) and the hydrophilic status by contact angle (CA) measurements of a water droplet.
Results
SEM and AFM revealed quantitative and qualitative differences between the roughened and smooth surfaces. UV treatment did not induce any topographic changes of the tested surfaces ( p > 0.05). All UV-treated samples showed a significant surface elemental content change with a decrease of carbon by 43–81%, an increase of oxygen by 19–45%, and an increase of zirconia by 9–41%. Upon UV treatment, a 19–25% increase of the crystalline monoclinic phase was observed on surfaces of material Zr1, whereas a slight increase on the smooth Zr2 surface (+3%) and a decrease on the roughened Zr2 surface by 20% was observed. For all samples, the hydrophilic status changed significantly from hydrophobic to hydrophilic by UV treatment ( p < 0.0001). The average contact angles were between 56.4° and 69° before and 2.5° and 14.1° after UV-light treatment.
Significance
UV treatment altered the physicochemical properties of the two zirconia implant surfaces investigated. The mechanism by which such changes are induced requires further investigation.
1
Introduction
The generation and maintenance of bone tissue around implants, referred to as osseointegration, distinguish this phenomenon from other biological responses of bone, such as wound healing and remodeling . It is well known today that the surface characteristics of implants and their alterations play an important role in the establishment of osseointegration . For example, investigations have shown that changes of surface roughness, i.e. R a values from 0.5 to 1.5 μm, results in more active bone tissue response, increases the percentage of bone-to-implant contact and yields higher forces to break implant-bone anchorage compared to implants with machine-turned or plasma-sprayed titanium surfaces . Consequently, most commercially available titanium implant surfaces are currently fabricated by 1- or a 2-processing steps such as oxidizing, sandblasting, acid-etching or combination of these techniques after machining . The resultant surfaces depict distinct topographic features that are visible at the micron and/or the supra-micron levels of resolution .
In addition to titanium, biomedical grade zirconia (ZrO 2 ) is one of the most frequently used implant materials . As a crystalline dioxide of the chemical element zirconium (Zr), it can be formed in three different structures, namely monoclinic, tetragonal and cubic . To improve its mechanical and crystalline stability, zirconia is generally mixed with other metallic oxides, such as MgO, CaO, CeO 2 or Y 2 O 3 . For instance, zirconia alloyed with yttria, which is presently the most studied material combination and also known as tetragonal zirconia polycrystal (Y-TZP), is obtained by adding 2–3 mol% of Y 2 O 3 .
Due to its favorable characteristics, specifically biomechanical stability and biocompatibility , osteoconductivity , soft tissue stability , low plaque accumulation and bone like color , zirconia has become popular as a material for the fabrication of implants targeted for the oral esthetic zone as well as for patients requesting a metal-free treatment . Despite different perceived and reported advantages, however, the long-term clinical evidence regarding zirconia implants is still lacking . Moreover, low temperature degradation (LTD), also termed “aging”, of the meta-stable tetragonal phase to monoclinic zirconia with time is considered a serious limitation of this material . Also surface-roughening procedures that are used for titanium may influence zirconia surface properties negatively . Thus, there is a need for specific surface modification techniques to enhance the clinical outcomes compared with current problems associated with “conventional” zirconia surface roughening procedures . As a consequence, zirconia implants are still not recommended for daily practice .
Recently, the effect of ultraviolet (UV) light on titanium surfaces has been shown to improve their bioactivity and osteoconductive capacity by increased protein adsorption, as well as osteogenic cell attachment and proliferation . This phenomenon, termed ‘UV light-mediated photofunctionalization’, has been found to be associated with the photochemical and photocatalytic removal of hydrocarbons from the titanium surfaces, the generation of hydrophilicity and a modification of the surface electrostatic properties .
For zirconia-based materials, a limited number of studies have investigated the effect of UV light treatment . However, the results of these studies are contradictory. Thus it was considered that an examination of the influence of UV treatment on well characterized zirconia surfaces would provide valuable information about the applicability of this technology. Therefore, the aim of this study is to evaluate the effect of UV treatment on the surface characteristics of two zirconia-based implant materials with different surface features.
2
Materials and methods
Altogether 34 discs of two zirconia-based materials (Zr1 and Zr2) with either smooth (m) or roughened (r) surfaces (20 mm in diameter and 1.5 mm in thickness) were used. Material Zr1 consisted of metal oxides, namely oxides of aluminum, zirconium, yttrium, cerium, hafnium, magnesium and also in low proportions, iron, lanthanum, chromium, strontium, silicon and calcium. In contrast, material Zr2 was a more conventional yttrium tetragonally stabilized zirconium oxide containing zirconium, hafnium and yttrium. While the smooth surface of material Zr1 was just the as sintered material, the smooth surface of Zr2 was additionally polished. The roughened discs of both materials were prepared by sandblasting with Al 2 O 3 , followed by acid-etching using 38–40% hydrofluoric acid (HF) and a final thermal treatment step at >1100 °C . All specimens were cleaned with 70% ethanol and bidistilled water, followed by ultrasonic cleaning in bidistilled water for 5 min and air-drying. Subsequently, the specimens were sterilized in a low-temperature hydrogen peroxide gas plasma sterilizer at 55 °C (STERRAD ® , 100NX™ System, Johnson & Johnson Medical, Norderstedt, Germany), sealed and stored for one month in the dark . Then, half of the discs were treated with UV light for 15 min in a UV-activation device (TheraBeam, Affiny, Ushio Inc, Otemachi, Japan). The UV light was delivered as a mixture of spectra via single source of UV lamp at λ = 360 nm and λ = 250 nm.
2.1
Scanning electron microscopy (SEM)
For each surface type, one untreated and one UV-treated disc ( n = 1) was examined using scanning electron microscopy (LEO 1525 Field Emission Gun, FEG SEM, Zeiss, Jena, Germany) at 20 kV and 225 mA current at low (5000×) and high magnifications (30,000×).
2.2
Atomic force microscopy (AFM)
The topographical features of the zirconia-based samples were studied via multimode atomic force microcopy (AFM) (Nanoscope IIIa, Veeco-Digital Instruments, Santa Barbara, USA). Due to the limited size of the AFM device, the discs were sectioned before evaluation. One section was used for each surface treatment group ( n = 1) resulting in altogether two discs for the whole AFM analysis. On each section, nine separate surfaces with 10 μm × 10 μm surface area at a 2-μm z -range were examined and the standard descriptors of roughness such as the arithmetic roughness ( R a ), the root mean square roughness (RMS) and the mean peak to valley height ( R z ) were calculated.
The cantilever tip (SSS-NCLR, Nanosensors, Neuchatel, Switzerland) was used in a tapping mode and had a tip radius of approximately 2 nm. The scan rate was set at 0.3 Hz and the resonance frequency was in the range of 146–236 kHz. An image correction “planefit order 1” was conducted for every single record. The generated images had a resolution of 512 × 512 pixels/data points. All roughness parameters and surface data were collected and averaged according to their groups.
2.3
X-ray photoelectron spectroscopy (XPS)
To examine the chemical composition of untreated and UV-treated surfaces, Electron Spectroscopy for Chemical Analysis (ESCA) was conducted by X-ray photoelectron spectroscopy (XPS) (Perkin Elmer PHI 5600 ESCA System, Physical Electronics, Inc., Chanhassen, Minnesota, USA) under ultra-high vacuum (UHV) conditions (5 × 10 −8 mbar). This system uses Mg Kα X-ray source (X-ray voltage: 13 KV, Anode Power 300 W) at a take off angle of 45°. The XPS spectra were obtained by irradiating a circular area of 800-μm diameter per sample surface with mono-energetic x-rays achieving a depth of penetration of 3–10 nm. One disc was used for each surface treatment group ( n = 1). In addition to the survey spectra, further detailed spectra for O1s, C1s and Zr3d electron energy levels were performed on the same samples. All spectra were aligned based on the binding energy of the O1s peak (O1s, 531 eV BE). The identification and correction of peaks was based on the NIST Database of principal lines in XPS . Obtained data and changes in elemental composition were quantified by data interpretation software for XPS (MultiPak Version 9, Physical Electronics, Inc., Chanhassen, MN, USA).
2.4
X-ray diffraction (XRD)
An X-ray diffractometer (XRD) with a Cu-Kα source (D 8 Advance X-ray diffractometer, Bruker AXS, Karlsruhe, Germany) was used to determine the crystalline phases of the untreated and UV-treated surfaces. XRD spectra were collected on the different samples over a 2 θ range between 15° and 70° at a step size of 0.01° and 4 s per step. An accelerating voltage of 40 kV and a beam current of 40 mA were used. The monoclinic phase fraction was calculated by the Garvie-Nicholson method . The identification and correction of peaks (monoclinic/tetragonal/cubic) was based on the ICSD database . One disc was used for each surface treatment group ( n = 1).
2.5
Contact angle measurements
The surface wettability of the zirconia discs was examined by contact angle measurements of 1-μl H 2 O droplets using a contact angle meter (Dataphysics, Model OCA 10, Data-Physics Instruments, Filderstadt, Germany). The H 2 O contact angles on both sides of a drop and at four different areas ( n = 4) of one zirconia surface (1 disc) were measured and averaged.
The number of used discs and their distribution according to the different tests is presented in Table 1 .
SEM | AFM | XPS | XRD | CA | Total | |
---|---|---|---|---|---|---|
Zr1-m | 1 | ¼ | 1 | 1 | 1 | 4 ¼ |
Zr1-m-UV | 1 | ¼ | 1 | 1 | 1 | 4 ¼ |
Zr1-r | 1 | ¼ | 1 | 1 | 1 | 4 ¼ |
Zr1-r-UV | 1 | ¼ | 1 | 1 | 1 | 4 ¼ |
Zr2-m | 1 | ¼ | 1 | 1 | 1 | 4 ¼ |
Zr2-m-UV | 1 | ¼ | 1 | 1 | 1 | 4 ¼ |
Zr2-r | 1 | ¼ | 1 | 1 | 1 | 4 ¼ |
Zr2-r-UV | 1 | ¼ | 1 | 1 | 1 | 4 ¼ |
Total | 8 | 2 | 8 | 8 | 8 | 34 |
2.6
Statistical analysis
A t -test was performed to analyze the differences in the roughness parameters between different surfaces. A significance level of α = 0.05 was set.
To evaluate the effect of material, surface and treatment on the contact angles, a linear mixed model with random intercept was performed, as repeated measurements per drop were undertaken. Least squares means (ls) was used for identifying the main effects, all interaction terms and differences of ls means between interaction terms of interest were calculated with their 95% confidence intervals. The calculations were performed with the statistical software SAS 9.2 using PROC MIXED and R version 2.13.1.
2
Materials and methods
Altogether 34 discs of two zirconia-based materials (Zr1 and Zr2) with either smooth (m) or roughened (r) surfaces (20 mm in diameter and 1.5 mm in thickness) were used. Material Zr1 consisted of metal oxides, namely oxides of aluminum, zirconium, yttrium, cerium, hafnium, magnesium and also in low proportions, iron, lanthanum, chromium, strontium, silicon and calcium. In contrast, material Zr2 was a more conventional yttrium tetragonally stabilized zirconium oxide containing zirconium, hafnium and yttrium. While the smooth surface of material Zr1 was just the as sintered material, the smooth surface of Zr2 was additionally polished. The roughened discs of both materials were prepared by sandblasting with Al 2 O 3 , followed by acid-etching using 38–40% hydrofluoric acid (HF) and a final thermal treatment step at >1100 °C . All specimens were cleaned with 70% ethanol and bidistilled water, followed by ultrasonic cleaning in bidistilled water for 5 min and air-drying. Subsequently, the specimens were sterilized in a low-temperature hydrogen peroxide gas plasma sterilizer at 55 °C (STERRAD ® , 100NX™ System, Johnson & Johnson Medical, Norderstedt, Germany), sealed and stored for one month in the dark . Then, half of the discs were treated with UV light for 15 min in a UV-activation device (TheraBeam, Affiny, Ushio Inc, Otemachi, Japan). The UV light was delivered as a mixture of spectra via single source of UV lamp at λ = 360 nm and λ = 250 nm.
2.1
Scanning electron microscopy (SEM)
For each surface type, one untreated and one UV-treated disc ( n = 1) was examined using scanning electron microscopy (LEO 1525 Field Emission Gun, FEG SEM, Zeiss, Jena, Germany) at 20 kV and 225 mA current at low (5000×) and high magnifications (30,000×).
2.2
Atomic force microscopy (AFM)
The topographical features of the zirconia-based samples were studied via multimode atomic force microcopy (AFM) (Nanoscope IIIa, Veeco-Digital Instruments, Santa Barbara, USA). Due to the limited size of the AFM device, the discs were sectioned before evaluation. One section was used for each surface treatment group ( n = 1) resulting in altogether two discs for the whole AFM analysis. On each section, nine separate surfaces with 10 μm × 10 μm surface area at a 2-μm z -range were examined and the standard descriptors of roughness such as the arithmetic roughness ( R a ), the root mean square roughness (RMS) and the mean peak to valley height ( R z ) were calculated.
The cantilever tip (SSS-NCLR, Nanosensors, Neuchatel, Switzerland) was used in a tapping mode and had a tip radius of approximately 2 nm. The scan rate was set at 0.3 Hz and the resonance frequency was in the range of 146–236 kHz. An image correction “planefit order 1” was conducted for every single record. The generated images had a resolution of 512 × 512 pixels/data points. All roughness parameters and surface data were collected and averaged according to their groups.
2.3
X-ray photoelectron spectroscopy (XPS)
To examine the chemical composition of untreated and UV-treated surfaces, Electron Spectroscopy for Chemical Analysis (ESCA) was conducted by X-ray photoelectron spectroscopy (XPS) (Perkin Elmer PHI 5600 ESCA System, Physical Electronics, Inc., Chanhassen, Minnesota, USA) under ultra-high vacuum (UHV) conditions (5 × 10 −8 mbar). This system uses Mg Kα X-ray source (X-ray voltage: 13 KV, Anode Power 300 W) at a take off angle of 45°. The XPS spectra were obtained by irradiating a circular area of 800-μm diameter per sample surface with mono-energetic x-rays achieving a depth of penetration of 3–10 nm. One disc was used for each surface treatment group ( n = 1). In addition to the survey spectra, further detailed spectra for O1s, C1s and Zr3d electron energy levels were performed on the same samples. All spectra were aligned based on the binding energy of the O1s peak (O1s, 531 eV BE). The identification and correction of peaks was based on the NIST Database of principal lines in XPS . Obtained data and changes in elemental composition were quantified by data interpretation software for XPS (MultiPak Version 9, Physical Electronics, Inc., Chanhassen, MN, USA).
2.4
X-ray diffraction (XRD)
An X-ray diffractometer (XRD) with a Cu-Kα source (D 8 Advance X-ray diffractometer, Bruker AXS, Karlsruhe, Germany) was used to determine the crystalline phases of the untreated and UV-treated surfaces. XRD spectra were collected on the different samples over a 2 θ range between 15° and 70° at a step size of 0.01° and 4 s per step. An accelerating voltage of 40 kV and a beam current of 40 mA were used. The monoclinic phase fraction was calculated by the Garvie-Nicholson method . The identification and correction of peaks (monoclinic/tetragonal/cubic) was based on the ICSD database . One disc was used for each surface treatment group ( n = 1).
2.5
Contact angle measurements
The surface wettability of the zirconia discs was examined by contact angle measurements of 1-μl H 2 O droplets using a contact angle meter (Dataphysics, Model OCA 10, Data-Physics Instruments, Filderstadt, Germany). The H 2 O contact angles on both sides of a drop and at four different areas ( n = 4) of one zirconia surface (1 disc) were measured and averaged.
The number of used discs and their distribution according to the different tests is presented in Table 1 .
SEM | AFM | XPS | XRD | CA | Total | |
---|---|---|---|---|---|---|
Zr1-m | 1 | ¼ | 1 | 1 | 1 | 4 ¼ |
Zr1-m-UV | 1 | ¼ | 1 | 1 | 1 | 4 ¼ |
Zr1-r | 1 | ¼ | 1 | 1 | 1 | 4 ¼ |
Zr1-r-UV | 1 | ¼ | 1 | 1 | 1 | 4 ¼ |
Zr2-m | 1 | ¼ | 1 | 1 | 1 | 4 ¼ |
Zr2-m-UV | 1 | ¼ | 1 | 1 | 1 | 4 ¼ |
Zr2-r | 1 | ¼ | 1 | 1 | 1 | 4 ¼ |
Zr2-r-UV | 1 | ¼ | 1 | 1 | 1 | 4 ¼ |
Total | 8 | 2 | 8 | 8 | 8 | 34 |
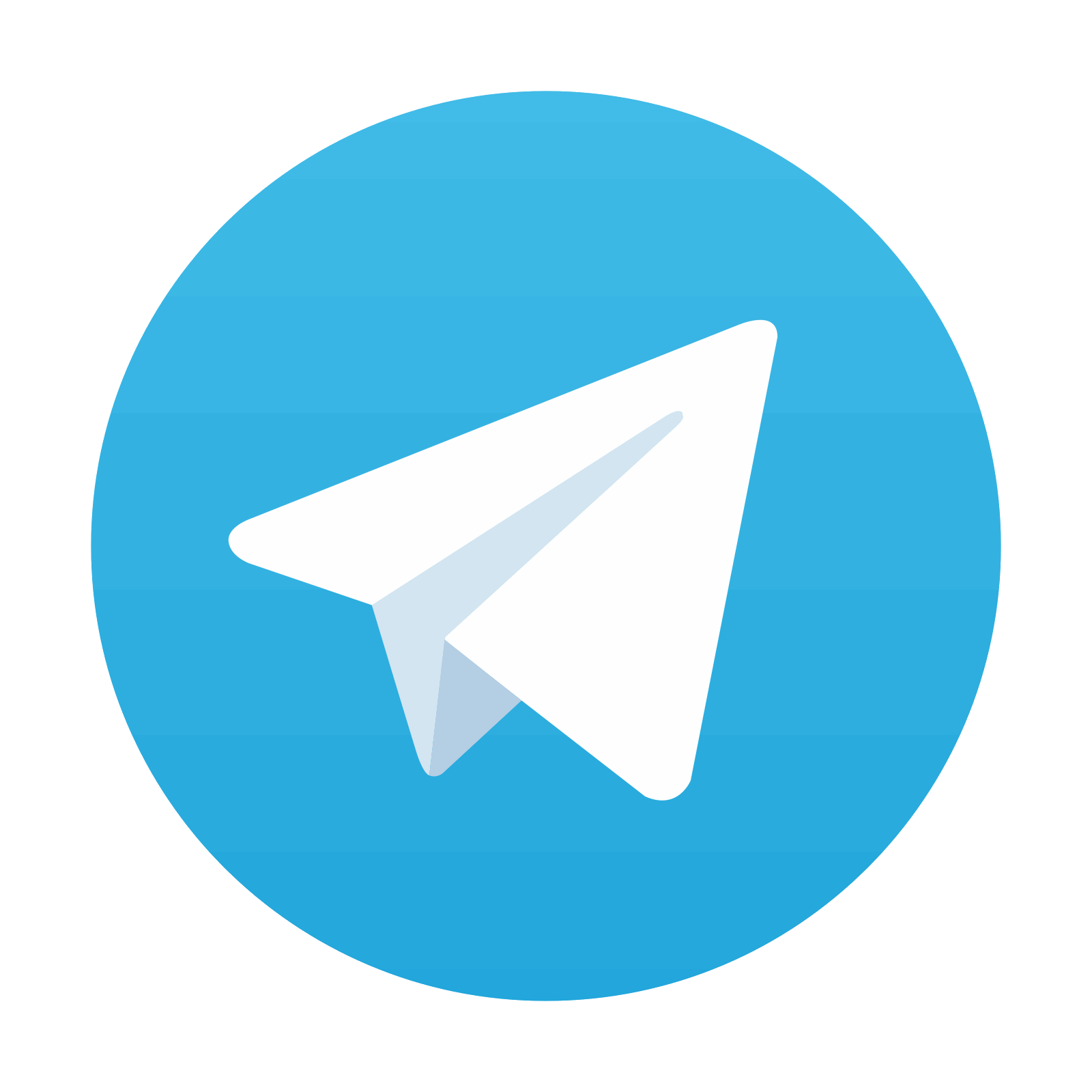
Stay updated, free dental videos. Join our Telegram channel

VIDEdental - Online dental courses
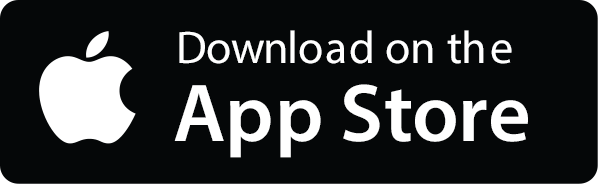
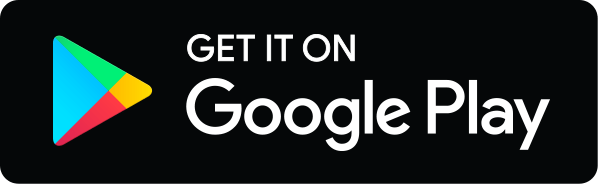
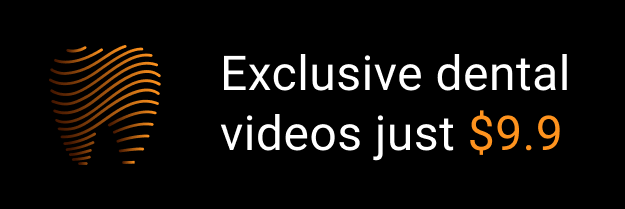