Abstract
Objective
To evaluate in vitro the pre-cementation resistance of CAD/CAM inlays subjected to functional occlusal tapping.
Methods
An extracted tooth model (molar and premolar) with simulated bone and periodontal ligament was used to make a medium-size mesio-occlusal inlay preparation (molar). Immediate dentin sealing was applied to the prepared tooth. The corresponding inlays were fabricated with Cerec either using composite resin (Paradigm MZ100) or ceramic (e.max CAD and Mark II) blocks ( n = 14). A high marginal ridge was designed in order to generate hyper-occlusion. Pre-cementation occlusal tapping was simulated using closed-loop servo-hydraulics at 2 Hz, starting with a load of 40 N, followed by 80, 120, 160, 200, 240, and 280 N (10 cycles each). All samples were loaded until fracture or to a maximum of 70 cycles. Groups were compared using the life table survival analysis ( p = 0.016, Bonferroni method).
Results
Survival probability was e.max CAD > MZ100 > Mark II. None of the specimens survived the 70 cycles except for two e.max CAD inlays (survival: 14%).
Significance
Material selection has a significant effect on the risk of Cerec inlay fracture during pre-cementation functional occlusal tapping.
1
Introduction
Bonded restorations have been widely recommended as a conservative and biomimetic treatment option, considering that significantly less tooth structure removal is needed when compared to conventional crown procedures . Prior to cementation, intracoronal indirect restorations should be tried in the patient’s mouth and assessed for interproximal contact, marginal fit, and occlusal contact . These delicate restorations resulting from minimally invasive preparations are fragile and brittle until bonded to the prepared tooth. It has been recommended therefore that those restorations not be subjected to occlusal forces before adhesive placement . There are significant drawbacks, however, of not being able to perform pre-cementation occlusal adjustments. Even in the case of optimal clinical conditions and the finest dental laboratory support, it is very likely that minor modifications of the restoration will be needed. While some changes can easily be done after cementation, other problems such as significant hyperocclusion or infraocclusion require substantial extraoral work, which cannot be carried out intraorally. In addition, major alterations of the restoration usually call for additional procedures such as staining, polishing and glazing. Both the patient’s and clinician’s discomfort can become substantial when major occlusion discrepancies are only detected after cementation. This will require lengthy corrective procedures resulting in altered anatomy, surface roughness, color discrepancies, as well as a stressful scheduling for the clinician.
Optimal patient comfort during the try-in process can be maintained by utilizing the immediate dentin sealing technique (IDS) , in which all the exposed dentin is etched, primed and resin-coated immediately after tooth preparation, before impression making. The resin coating acts as insulation so that anesthesia might not be needed during restoration delivery, allowing the patient to better control tapping forces and improve their ability to detect minor occlusal discrepancies. Studies have reported an increase in bite force development induced by anesthetization . Maximum bite force varies significantly and is in the range of 234–597 N for women and 306–847 N for men . De Boever et al. , however, demonstrated that the controlled bite force during tapping is much lower, approximately 22 N. Another aspect to consider is the maximum cementation force, approximately 25 N . Considering the development of stronger materials compared to traditional feldspathic porcelain, it calls into question whether those inlays/onlays require cautious handling during try-in and cementation. It can be hypothesized that most modern materials used to fabricate bonded restorations have flexural strength and toughness that will sustain tapping/cementation forces. This issue, however, has not been addressed in the literature.
The aim of the present study was to evaluate in vitro the pre-cementation resistance of CAD/CAM inlays subjected to functional occlusal tapping. The influence of different machinable materials was assessed: high-strength ceramic, composite resin, and feldspathic porcelain. The null hypothesis was that the try-in resistance of the inlays would not be different among the different inlay materials.
2
Material and methods
2.1
Specimen preparation
One freshly extracted maxillary first molar and one premolar, stored in solution saturated with 0.1% thymol, were used upon approval from the University of Southern California Institutional Review Board ( Fig. 1 A ). Two layers of a water-based liquid latex (Rubber-Sep; Kerr Corporation, Orange, CA) were applied on the roots in order to simulate the periodontal ligament ( Fig. 1 B) . Teeth were positioned in contact and the roots were embedded in acrylic resin (Palapress; Haereus Kulzer, Armonk, NY) up to 3.0 mm below the cement–enamel junction (CEJ).
The molar received a mesio-occlusal inlay preparation (using a round-ended tapered diamond bur (6856-027; Brasseler, Savannah, GA), including a narrow occlusal isthmus in order to predetermine the location where the fracture would occur detailed dimensions in Fig. 2 ). The dentin was sealed with 3-step etch-and-rinse dentin bonding agent (Optibond FL; Kerr, Orange, CA) immediately following tooth preparation. An air-blocking barrier (K-Y Jelly; Personal Products Company, Skillman, NJ) was applied and followed by 10 s of additional light exposure (Allegro; Den-Mat, Santa Maria, CA) to polymerize the oxygen-inhibition layer.
2.2
Restoration design and manufacturing
Standardized inlays were generated with the Cerec 3 CAD/CAM system (Cerec software v. 3.03, Sirona Dental Systems GmbH, Bensheim, Germany) ( Fig. 3 A ). All restorations were identical in size and anatomy because they were produced by the multiple milling of the same design. The latter included a marginal ridge slightly higher than the neighboring premolar marginal ridge. This intentional flaw was included to simulate hyperocclusion. Fourteen inlays were milled for each restorative material: e.max CAD (Ivoclar; Schaan, Liechtenstein), Paradigm MZ100 (3 M/ESPE, Saint Paul, MN), and Vita MarK II Blocks (Vident; Brea, CA). The restorations milled with lithium disilicate blocks were crystallized in a ceramic furnace (Austromat D4, DEKEMA Dental-Keramiköfen GmbH, Freilassing, Germany) following the manufacturer’s instructions (Ivoclar Vivadent AG). The surface polishing of the Mark II and e.max CAD inlays was performed mechanically using diamond ceramic polishers (Dialite, Brasseler), while the Paradigm MZ100 inlays were finished with brushes (Jiffy Composite Polishing Brushes, Ultradent, South Jordan, UT). A stone replica of the preparation was used for holding the inlay during finishing procedures.
2.3
Occlusal tapping test
The inlay was positioned inside the wet prepared tooth. An artificial mouth using closed-loop servohydraulics (Mini Bionix II; MTS Systems, Eden Prairie, MN) was used to simulate occlusal tapping forces. The try-in cycle was replicated by an isometric contraction (load control) applied through a 7-mm-diameter composite resin sphere (Filtek Z100, 3M/ESPE) post-cured at 100 °C for 5 min. Due to their identical occlusal anatomy, all specimens could be positioned in the same reproducible location with the sphere contacting the inner slope of the mesial marginal ridge ( Fig. 3 B). Cyclic occlusal tapping was applied at a frequency of 2 Hz, starting with a load of 40 N for 10 cycles followed by stages of 80, 120, 160, 200, 240, and 280 N at a maximum of 10 cycles each. The specimens were loaded until fracture or to a maximum of 70 cycles. The failure load was recorded.
2.4
Statistical analysis
The fracture resistance of the three groups was compared using the life table survival analysis. At each time interval (defined by each load step), the number of inlays starting the interval intact and the number of inlays that fractured during the interval were counted, allowing the calculation of survival probability at each interval. The influence of the restorative material on the fracture resistance was determined by comparing the survival curves using the log rank test at a significance level of 0.05. Differences were identified using pairwise post hoc comparisons with the same test at a significance level of 0.016 (Bonferroni correction for 3 comparisons). The statistical analysis was carried out with MedCalc Version 11.0.1 (Mariakerke, Belgium).
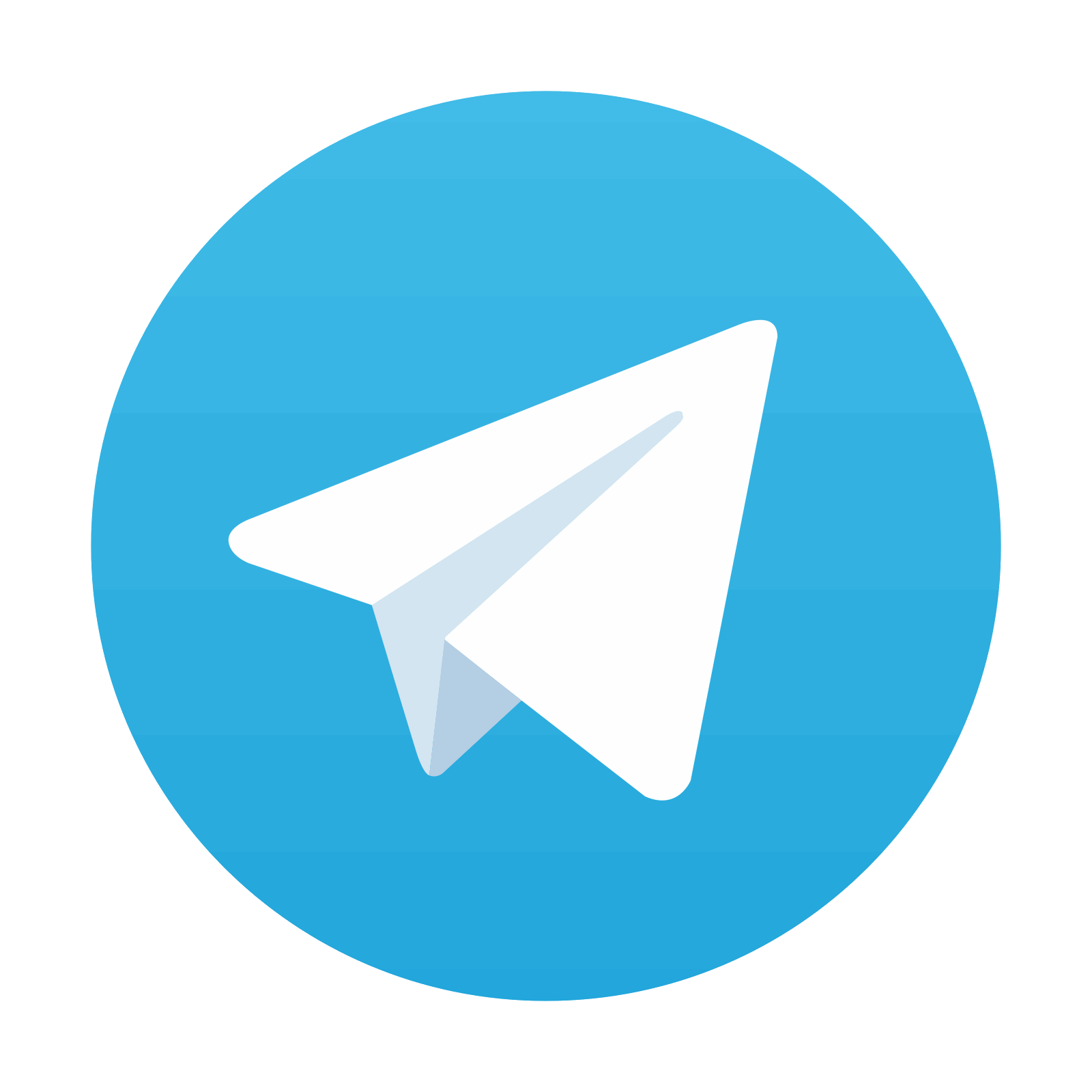
Stay updated, free dental videos. Join our Telegram channel

VIDEdental - Online dental courses
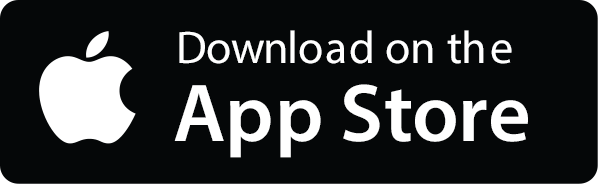
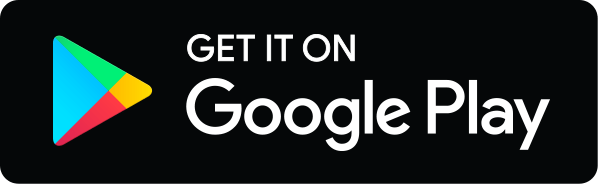