Abstract
Previously, an in vitro cellular carcinogenesis model of oral squamous cell carcinoma (OSCC) was established with a line of human immortalized oral epithelial cells (HIOECs), a line of cancerous HB96 cells and another type of cell (HB56 cells) at the early stage of carcinogenesis. In this study, comparative proteomic analysis identified a panel of differentially expressed proteins among these cells. Cathepsin B was one of the significantly up-regulated proteins accompanying cellular transformation. Cathepsin B was further validated for its expression in the three cell lines and in clinical samples of tumour tissues and their adjacent normal epithelia from 30 primary OSCC patients. Western blot analysis and real-time PCR detected increased Cathepsin B protein and mRNA levels in the cancerous HB56 and HB96 cells over HIOECs. Immunohistochemistry and real-time PCR showed elevated Cathepsin B protein and mRNA levels in the tumour tissues over the adjacent non-malignant epithelia from OSCC patients. The results presented here suggest that the expression of Cathepsin B increases along with the cancerisation in OSCC both in vitro and in vivo , and it may serve as a candidate biomarker of OSCC.
Oral squamous cell carcinoma (OSCC) is the most common malignant tumour in the oral and maxillofacial region, with about 300,000 new cases worldwide each year . The 5-year survival rate of patients with OSCC has remained unchanged in recent years, at approximately 50–60% .
Tumour markers that can distinguish differences in tumour condition and behaviour in vitro or in vivo are expected to be useful for diagnosis and prognostic monitoring. Tissue samples from different subjects generally have individual variations, and their availability is also limited. In contrast, cell lines offer a stable supply of homogeneous samples, therefore, in vitro cell models play an important role in understanding cellular events related to pathophysiological conditions in humans . Previously, the authors successfully established the first in vitro cellular carcinogenesis model of OSCC in China , including a human immortalized oral epithelial cell (HIOEC) line, immortalized from normal epithelial cells of oral mucosa by transfection of HPV16 E6/E7 gene, a cancerous cell line HIOEC-B(a)P-96 (HB96) induced by benzo(a)pyrene from the HIOEC line, and cells (HB56) at the early stage of carcinogenesis between HIOEC and HB96. The biological characters of HIOEC, HB56 and HB96 have been previously described including cell morphology, cell ultrastructure, cell growth, cell circle analysis, immunocytochemistry, in vitro invasion ability and tumourigenicity. In mice injected subcutaneously with HIOECs, no neoplasm forms. In mice injected with HB56 cells, the neoplasm formed has an intact envelope with two to three layers of epithelial cells under the envelope, and keratinized massive keratinocytes under the epithelial cells, parakeratosis could also be found in some keratinocytes with a few atypical hyperplasia. In mice injected with HB96 cells, the neoplasm formed is typical squamous cell carcinoma; the differentiation of tumour cells was good to moderate with obvious atypical hyperplasia and pathological mitosis. These three types of cells (HIOEC, HB56 and HB96) constitute the major components of the in vitro cellular carcinogenesis model of OSCC .
Proteomics is a new biochemical technology for cancer research, aiming to discover differential protein expression associated with cancer development and progression . Comparative proteomic analysis has been used as an innovative method to investigate different protein expressions between cancerous and normal tissues and cells. It is thought to have several advantages in protein research, and it has been successfully employed in many kinds of cancers, including OSCC in clinical tissue samples and cell lines .
In this study, we firstly identified the differentially expressed proteins using the three kinds of cells from our in vitro cellular carcinogenesis model of OSCC by comparative proteomics. Among the panel of differentially expressed proteins identified, we further validated several significantly up-regulated proteins both in vitro and in vivo , for our potential usefulness as clinical markers of OSCC.
Cathepsin B (CTSB) was chosen for such validation. It is a lysosomal cysteine protease of the papain superfamily with endo- and exo-peptidase activities . It is thought to participate in the intracellular degradation and turnover of proteins . In malignant tumours and premalignant lesions, the expression of CTSB is highly up-regulated and the enzyme is secreted and becomes associated with the cell surface . Close correlations have been observed between CTSB levels and the invasive potential of tumour cells . CTSB has been reported to be overexpressed in various malignant tumours, including OSCC . Despite this, the validation of CTSB expression in OSCC, both in vitro and in vivo , has seldom been reported. The aim of this study was to confirm the increased CTSB protein expression and gene transcription during the carcinogenesis process of OSCC, as well as its clinical value.
Materials and methods
Cell cultures
HIOECs, HB56 cells and HB96 cells were previously described as the three major components of our in vitro cellular carcinogenesis model of OSCC , and they were all maintained in a cell culture incubator under humidified atmosphere of 5% CO 2 at 37 °C. HIOECs were cultured in the defined keratinocyte-SFM (Gibco, USA); HB56 and HB96 cells were cultured in DMEM (Dulbecco’s modified Eagle’s medium, Gibco, USA) supplemented with 10% foetal bovine serum, 1% glutamine and 1% penicillin-streptomycin. The biological characters of HIOEC, HB56 and HB96 have been previously described including cell morphology, cell ultrastructure, cell growth, cell circle analysis, immunocytochemistry, in vitro invasion ability and tumourigenicity. In mice injected subcutaneously with HIOECs, no neoplasm forms. In mice injected with HB56 cells, the neoplasm formed has an intact envelope with two to three layers of epithelial cells under the envelope, and keratinized massive keratinocytes under the epithelial cells, parakeratosis could also be found in some keratinocytes with few atypical hyperplasia. In mice injected with HB96 cells, the neoplasm formed is typical squamous cell carcinoma; the differentiation of tumour cells was good to moderate with obvious atypical hyperplasia and pathological mitoschisis .
Clinical tissue samples
From February 2007 to July 2007, 30 primary OSCC patients without prior radiotherapy or chemotherapy were enrolled in this study. There were 21 males and 9 females, aged from 31 to 84 years with a mean age of 53.8 years. After signing informed consent forms, they underwent radical surgery. Surgical tissue samples, including cancerous tissue and adjacent non-malignant epithelium were collected by procedures previously described . The adjacent non-malignant epithelia were collected at sites at least 2 cm away from the edge of tumour mass, with best efforts being made to avoid contamination by tumour cells. The sites of primary carcinoma were tongue ( n = 17), buccal mucosa ( n = 4), retromolar region ( n = 3), floor of mouth ( n = 3), gingiva ( n = 2) and palatoglossal arch ( n = 1). The stage of disease was determined according to the tumour–node–metastasis staging (TNM) system of the International Union Against Cancer . The histological grade of the tumour was determined according to the degree of differentiation using the WHO histological criteria . The authors scored a well-differentiated grade as number 1 (less malignant); moderately differentiated grade for number 2; and poorly differentiated grade for number 3 (more malignant).
Two-dimensional gel electrophoresis (2-DE) and liquid chromatography–tandem mass chromatography (LC–MS/MS)
HIOECs and HB cells at 80% confluency were lysed in a 300 μl ice-cold lysis buffer containing 8 M Urea, 65 mM DTT, 4% (w/v) CHAPS, 40 mM Tris and freshly prepared 100 μg/ml PMSF. They were sonicated 10 times (15 s each) and centrifuged at 12,000 × g for 90 min. The concentration of crude proteins in the supernatant was determined with the Bradford protein assay reagent (B radford et al., 1976; Bio-Rad protein Dye assay reagent, USA). The 17 cm pH3–10 IPG strips (Bio-Rad Cat. No. 163-2009) were firstly rehydrated in sample buffer at 17 °C, and then subjected to IEF following the manufacturer’s instructions (Bio-Rad, USA) with modifications. IEF was run at 250 V for 30 min at linear mode, followed by 1000 V for 60 min at rapid mode, 10,000 V for 5 h at linear mode, 10,000 V for 6 h at rapid mode and 500 V at linear mode. After IEF, strips were equilibrated for 2 × 15 min at room temperature in equilibration buffers (6 M urea, 30% glycerin, 2% SDS, 375 mM Tris–HCl pH8.8), firstly with supplemented 2% DTT and then with 2.5% iodoacetamide to replace DTT. Equilibrated IPG strips were then transferred onto 12% uniform polyacrylamide gels, and the second-dimensional SDS-PAGE was performed with a Hofer SE 600 Ruby System (Amersham Biosciences, Sweden). The constant current was firstly 5 mA/gel and then 30 mA/gel until the bromophenol blue frontier reached the bottom of the gels. Two-dimensional standards were added to the protein samples as internal markers to determine the isoelectric point (p I ) and molecular weight (Mr). The gels were stained by AgNO 3 for 20 min, and scanned by the Bio-Rad GS710 scanner (Bio-Rad, USA). Spot detection and matching were performed using PDQuest software version 7.3.0 (Bio-Rad, USA).
Following the automated protein localisation and quantification, gel spots carrying the proteins were excised by a spot cutter (Bio-Rad, USA) controlled by the PDQuest software (Bio-Rad). Excised gels were then triturated and washed with ammonium bicarbonate and acetonitrile. Trypsin was used to digest the protein. The peptide solutions were then dried by vacuum centrifugation and desalted and cleaned using a C18 Ziptip (Millipore, USA). Peptide mixtures were separated and identified by a Finnigan LTQ mass spectrometer coupled with a Surveyor HPLC system (ThermoQuest, USA). Protein identification using MS/MS raw data was performed with Sequest program in the BioWorks 3.1 software suite (University of Washington, licensed to Thermo Finnigan) based on the IPI Human database version 3.15.1. Trypsin was selected as protein cleavage specificity. Protein identification results were filtered with the Xcorr (≥1.9 for a 1+ tryptic peptide, ≥2.2 for a 2+ tryptic peptide, ≥3.75 for a 3+ tryptic peptide) and ΔCn (≥0.1).
Real-time PCR
The total RNA of each tissue sample and the cultured cells was isolated using TRIzol reagent (Invitrogen, USA) according to the manufacturer’s instructions. RNAse-free DNase I was used to remove DNA contamination. RNA concentration and purity were assessed at 260 nm using a spectrophotometer (DU 530, Beckman-Coulter Inc., Fullerton, CA, USA). M-MLV (Promage, USA) and random primers (Amersham Biosciences, USA) were used for reverse transcription of 2 μg total RNA into first strand cDNA. Real-time PCR was performed with Thermal Cycler DiceTM Real Time System (TaKaRa, Japan). cDNA diluted 1:4 was mixed with SYBR Premix Ex Taq (TaKaRa, Japan). The primer sequences of CTSB ( NM_001908 ) were designed by primer premier 5.0 (Premier Biosoft International, CA, USA). The primer sequences of CTSB were: forward 5′-GGCCCCCTGCATCTATCG-3′, reverse 5′-AGGTCTCCCGCTGTTCCACTG-3′, and the length of product was 136 bp. The primer sequences of β-actin were: forward 5′-TCACCCACACTGTGCCCATCTACGA-3′, reverse 5′-CAGCGGAACCGCTCATTGCCAATGG-3′, and the length of product was 100 bp. The condition for PCR reaction was: 95 °C for 10 s followed by 40 cycles of denaturation at 95 °C for 5 s, annealing elongation at 60 °C for 30 s. The relative quantification of CTSB mRNA level compared with internal control gene β-actin was calculated according to the 2(−Delta Delta C(T)) method . All samples were run in duplicates and the relative quantification of each target gene expression was carried out twice.
Western blot analysis
Cultured cells grown to 80% confluence were lysed in ice-cold 2× lysis buffer containing 125 mM Tris–HCl (pH6.8), 5% (w/v) SDS and 24.75% glycerol, and subjected to total protein extraction according to the standard procedures. After concentration determination by the Bradford assay (BCA™, Pierce, USA), protein samples (50 μg/lane) were separated by 12% SDS-PAGE (sodium dodecyl sulphate polyacrylamide gel), and then electrophoretically transferred onto polyvinylidene difluoride membranes using a wet transfer system (Invitrogen, USA). The membranes were blocked with blocking buffer containing 5% dry milk in PBS with 0.1% Tween 20 and incubated overnight with rabbit anti-CTSB polyclonal antibody (ABcam, Cambridge, UK) at 1:1000 dilution. After washing, the blot was then incubated with fluorescent-conjugated anti-rabbit IgG secondary antibody (Fermentas, Vilnius, Lithuania) at 1:1000 dilution for 1 h. Finally, the immunoreactive bands were scanned and analysed using the Odyssey Infrared Imaging System (LI-COR Biosciences, USA).
Immunohistochemistry
Thirty pairs of tissue samples from each patient, including cancerous tissue and adjacent non-malignant epithelium, were detected using immunohistochemical staining for CTSB. The immunohistochemistry procedure followed the method previously described . In brief, after deparaffinisation and endogenous peroxidase blocked, the sections were heated by water bath at 98 °C with 0.01 M citrate buffer solution (pH6.0) for 20 min, then incubated with rabbit anti-CTSB polyclonal antibody (Abcam, Cambridge, UK) at 1:500 dilution overnight at 4 °C, and visualized using 3,3′-diaminobenzidine (DAB) detection kit (Dako Cytomation, Denmark). The 1:500 dilution was the best dilution compared with 1:100, 1:200 and 1:1000. Negative control was prepared using PBS instead of antibody. Microscopic examination was performed by two pathologists and all samples were blinded. The CTSB positive grade was determined based on the proportion of stained cells on a scale of negative to strong as follows: negative, 0% of stained cells with the grade of 0; weak, 1–25% of stained cells with grade 1; moderate, 26–50% of stained cells with grade 2; and strong, more than 50% of stained cells with grade 3.
Statistical analysis
All data were analysed using the statistical software of SPSS11.0 for Windows (SPSS Inc., USA). The statistical difference of the initial data was analysed using nonparametric tests. When the P -value was less than 0.05, the difference was regarded as statistically significant.
Results
2-DE and LC–MS/MS
Using the 2-DE, the authors compared the protein expression profiles of the HIOECs, HB56 cells and HB96 cells. There were 54 spots in the 2-DE gels representing proteins up- or down-regulated among these cells, and 29 unique spots in the HIOECs, three unique spots in the HB56 cells and 22 unique spots in the HB96 cells. Using the LC–MS/MS, the authors identified 45 differentially expressed proteins. Of those, 26 different proteins were in the HIOECs, three proteins in the HB56 cells and 19 proteins in the HB96 cells (detail in Table 1 ). Among them, one protein significantly up-regulated in the HB56 and HB96 cells was marked by the circles in Fig. 1 A–C , and later identified as CTSB protein. By searching the IPI Human database version 3.15.1 with Sequest program, its peptide fingerprint matched the known peptides, as shown in Fig. 1 D and E.
Name of cells | Abbreviations of gene names according to the identified differentially expressed proteins |
---|---|
HIOECs | ACP1, ANXA1, ECH1, ETHE1, GLO1, GRB2, HCC1, ISG15, KRT7, NDUFV2, PCBP2, PFN2, PRDX1, PSMB3, Q8N849, RANBP1, RPS12, SOD2, S100A6, S100A8, TPD52L2, TPI1, TXNL4A, VBP1, ZYX, 44 kDa protein-ENSP00000319797 |
HB56 cells | ANXA2, CFL, KRT17 |
HB96 cells | ANXA2, CAPZA1, CTSB, EEF2, ERH, GAPDH, GNB1, LASP1, LGALS1, M6PRBP1, PDHB, PFN2, RANBP1, RPP2, SERPINB5, STMN1, TUBB2C, TUFM, UCHL1 |
CTSB protein and mRNA levels in the in vitro carcinogenesis model of OSCC
Western blot analysis revealed that CTSB protein expressions increased in both HB56 and HB96 cells compared with HIOECs on normalisation against β-actin protein signal ( Fig. 2 A and B ). The expression pattern was consistent with that obtained from the comparative proteomic analysis. Real-time PCR showed that CTSB mRNA level also increased in both HB56 and HB96 cells compared with HIOECs after normalisation against β-actin ( Fig. 2 C).
CTSB protein and mRNA levels in the tissue samples from OSCC patients
By immunohistochemistry, non-malignant epithelia adjacent to tumour showed negative reactivity to CTSB antibody in 47% (14/30) cases, positive reactivity of grade 1 in 50% (15/30) cases and grade 3 in 3% (1/30). The positive rate in the adjacent non-malignant epithelia was 53% (16/30), but that in the cancerous tissues was 100% (30/30). As shown in Table 2 , the average positive grade of CTSB protein in cancerous tissues was 1.97 ± 0.76, significantly higher than that in the paired adjacent non-malignant epithelia (0.60 ± 0.67) (Wilcoxon signed ranks test, Z = −4.532, P < 0.001). The positive grade of CTSB protein in the adjacent epithelia was relatively weaker ( Fig. 3 A ) than that in the cancerous tissues, including well ( Fig. 3 B), moderately ( Fig. 3 C) and poorly differentiation grade ( Fig. 3 D); but the difference of positive grade was not significant according to the classification of pathological differentiation grade ( Table 3 ). No correlation was found between the positive grade of CTSB protein and the T stage ( P = 0.131), N stage ( P = 0.824), clinical stage ( P = 0.342) of the patients, or the patient’s exposures to smoking ( P = 0.502) and drinking ( P = 0.248) ( Table 3 ).
Types of tissues | Case number | Cathepsin B positive grade | |||
---|---|---|---|---|---|
0 | 1 | 2 | 3 | ||
Adjacent non-malignant epithelium | 30 | 14 | 15 | 0 | 1 |
Cancerous tissue | 30 | 0 | 9 | 13 | 8 |
Classification | Case | mRNA (relative level) | P -value | Protein positive grade | P -value | ||
---|---|---|---|---|---|---|---|
Sex | |||||||
Male | 21 | 2.397 ± 2.456 | Z = −0.520 | 0.603 | 2.10 ± 0.77 | Z = −1.404 | 0.160 |
Female | 9 | 1.900 ± 1.621 | 1.67 ± 0.71 | ||||
Smoking | |||||||
Yes | 13 | 1.790 ± 1.200 | Z = −0.314 | 0.754 | 2.08 ± 0.86 | Z = −0.672 | 0.502 |
No | 17 | 2.599 ± 2.751 | 1.88 ± 0.70 | ||||
Drinking | |||||||
Yes | 12 | 2.511 ± 2.784 | Z = −0.381 | 0.703 | 2.17 ± 0.83 | Z = −1.155 | 0.248 |
No | 18 | 2.073 ± 1.824 | 1.83 ± 0.71 | ||||
T stage | |||||||
T1 | 6 | 2.497 ± 1.651 | χ 2 = 1.888 d.f. = 3 |
0.596 | 1.33 ± 0.52 | χ 2 = 5.635 d.f. = 3 |
0.131 |
T2 | 13 | 2.139 ± 1.904 | 2.08 ± 0.76 | ||||
T3 | 4 | 1.148 ± 0.694 | 2.00 ± 0.82 | ||||
T4 | 7 | 2.867 ± 3.572 | 2.29 ± 0.76 | ||||
N stage | |||||||
N0 | 16 | 2.485 ± 2.550 | Z = −0.665 | 0.506 | 1.94 ± 0.77 | Z = −0.222 | 0.824 |
N1–2 | 14 | 1.978 ± 1.835 | 2.00 ± 0.78 | ||||
Clinical stage | |||||||
I | 5 | 2.314 ± 1.777 | χ 2 = 1.287 d.f. = 3 |
0.732 | 1.40 ± 0.55 | χ 2 = 3.344 d.f. = 3 |
0.342 |
II | 8 | 1.700 ± 1.225 | 2.13 ± 0.83 | ||||
III | 2 | 4.272 ± 4.049 | 2.00 ± 0.00 | ||||
IV | 15 | 2.248 ± 2.578 | 2.07 ± 0.80 | ||||
I + II | 13 | 1.936 ± 1.423 | Z = −0.188 | 0.851 | 1.85 ± 0.80 | Z = −0.761 | 0.447 |
III + IV | 17 | 2.487 ± 2.701 | 2.06 ± 0.75 | ||||
Pathologic differentiation grade | |||||||
Well | 12 | 1.761 ± 0.977 | χ 2 = 1.009 d.f. = 2 |
0.604 | 1.83 ± 0.72 | χ 2 = 0.613 d.f. = 2 |
0.736 |
Moderately | 15 | 2.266 ± 2.585 | 2.07 ± 0.80 | ||||
Poorly | 3 | 4.227 ± 3.476 | 2.00 ± 1.00 |
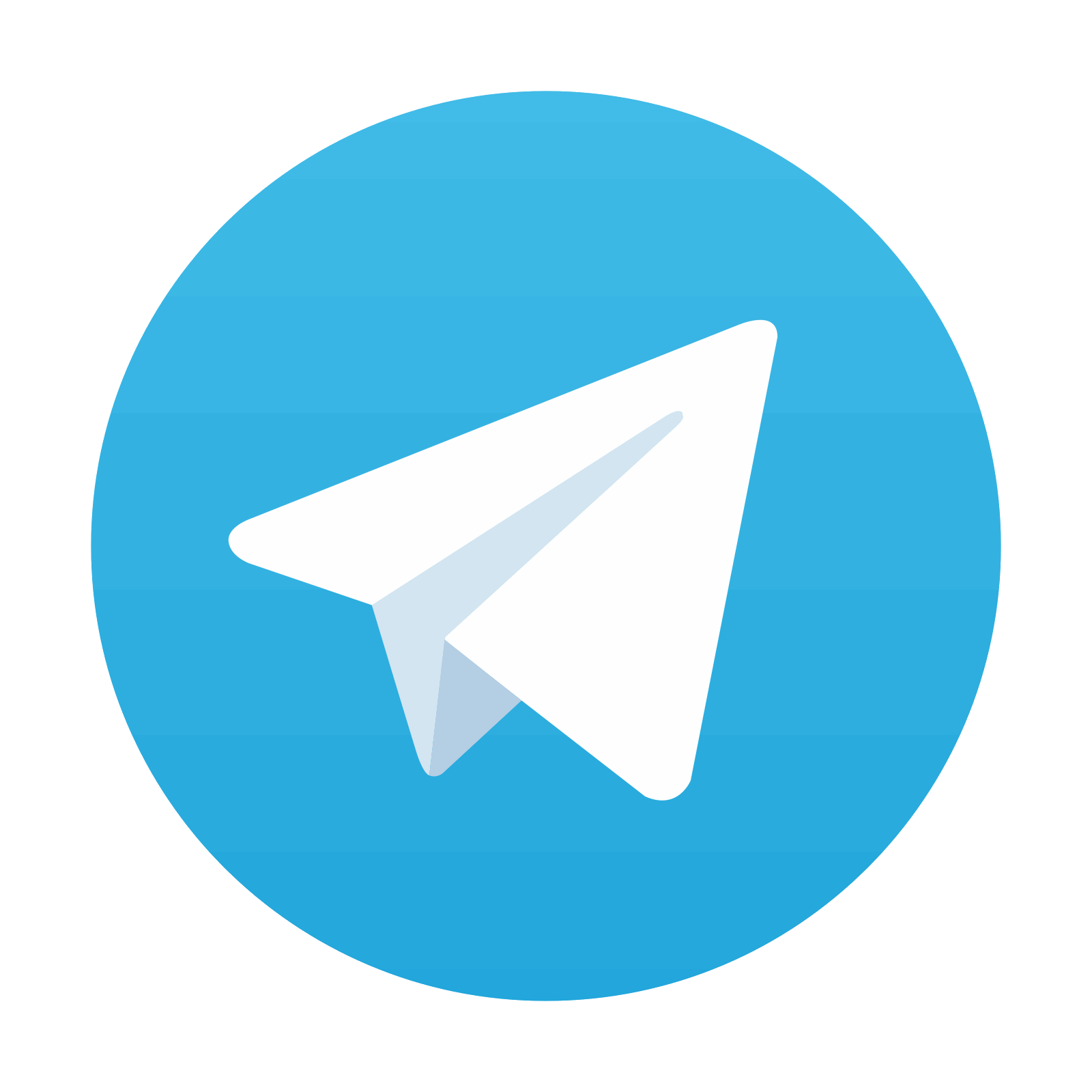
Stay updated, free dental videos. Join our Telegram channel

VIDEdental - Online dental courses
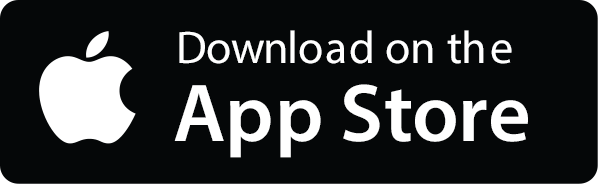
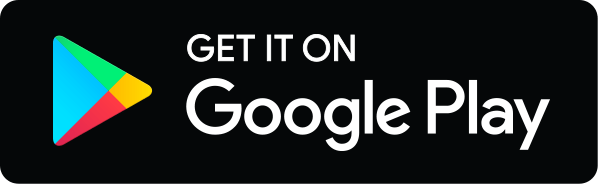
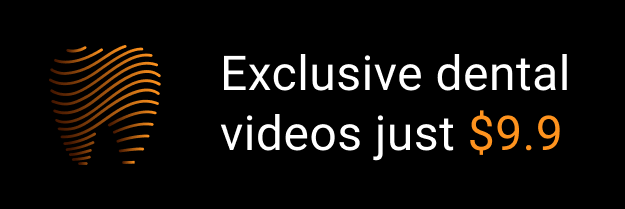