Abstract
Objectives
The in-vitro dissolution of bioactive glass-based toothpastes and their capacity to form apatite-like phases in buffer solutions have been investigated.
Materials and methods
The commercial toothpaste samples were tested on immersion in artificial saliva, Earle’s salt solution and Tris buffer for duration from 10 min to four days. The powder samples collected at the end of the immersion were studied using solid-state 31 P and 19 F nuclear magnetic resonance spectroscopy (NMR), X-ray powder diffraction and Fourier transform infrared (FTIR) spectroscopy. The fluoride concentration in the solution remained after the immersion was measured.
Results
In artificial saliva and in presence of sodium monofluorophosphate (MFP), the bioactive glass and bioactive glass-based toothpastes formed fluoridated apatite-like phases in under 10 min. A small amount of apatite-like phase was detected by 31 P NMR in the toothpaste with MFP but no bioactive glass. The toothpaste with bioactive glass but no fluoride formed an apatite-like phase as rapidly as the paste containing bioactive glass and fluoride. By contrast, apatite-like phase formation was much slower in Earle’s salt solution than artificial saliva and slower than Tris buffer.
Conclusions
The results of this lab-based study showed that the toothpaste with MFP and bioactive glass formed a fluoridated apatite in artificial saliva and in Tris buffer, as did the mixture of bioactive glass and MFP.
Clinical significance
The presence of fluoride in bioactive glass-containing toothpastes can potentially lead to the formation of a fluoridated apatite, which may result in improved clinical effectiveness and durability. However, this should be further tested intra-orally.
1
Introduction
Enamel, the outer layer of tooth, is formed of 96% (by weight) of an inorganic calcium phosphate mineral called apatite , whereas the tooth structure underneath enamel called dentine is less mineralized and consists of by weight 70% hydroxyapatite (HA), 20% organic matter and 10% water . Enamel loss can occur due to a combination of the processes and process of demineralization in the oral conditions is one of them. For example intrinsic and extrinsic acids can cause enamel erosion, whereas acids produced by bacterial action can result in caries . Complete depletion of enamel as a result of aggressive demineralization conditions can expose dentinal tubules and causes tooth sensitivity typically triggered by thermal, osmotic or tactile stimuli including for example hot and cold foods or drinks.
Re-mineralization of enamel occurs by the deposition of ions on the surface from saliva, since enamel has no capability to repair itself as it has no cells. The presence of phosphate and calcium ions in the saliva helps re-mineralization of the enamel . In addition, fluorides are well known for their potential to inhibit and repair caries, since fluoridated apatite (FAP) is more resistant to acids. Various toothpastes with added sources of these ions have been developed to help restore partially the lost enamel. Calcium carbonate has been used as an abrasive in toothpaste since the 1850s. It was found that toothpaste with calcium carbonate and fluoride from sodium monofluorophosphate reduces the caries by elevating the level of plaque calcium and hence fluoride . Tschoppe et al. have demonstrated that toothpastes containing nano sized HA have higher re-mineralization capacity than amine fluoride toothpaste. Hornby et al. have shown that toothpaste containing HA and fluoride in the form of sodium monofluorophosphate reduces acid mediated enamel demineralization and increases re-mineralization compared to fluoride free toothpaste. Vanichvatana et al. have reported the re-mineralization effect of fluoride-containing Casein Phosphopeptide Amorphous Calcium Phosphate (CPP-ACP) and beta tricalcium phosphate (β-TCP) and sodium lauryl sulfate. Recent studies have shown that calcium silicate/phosphate-containing toothpaste reduces enamel demineralization and enhances re-mineralization compared to the fluoride and non-fluoride controls . This has been attributed to the deposition of calcium silicate on the tooth surface, which releases calcium ions into oral fluids to help nucleate hydroxyapatite. In addition, calcium silicate also buffers the oral fluid by absorbing protons.
Bioactive glass (BAG) was originally invented by Hench as a bone regenerative material. Bioactive glasses form hydroxycarbonated (HCA) apatite following immersion in body fluids. It was discovered later that incorporation of BAG into dentifrices occludes the dentinal tubules and can be useful for the treatment of demineralized teeth and prevent further demineralization . A recent report demonstrated the formation of apatite from the commercial bioactive glass-based toothpaste in re-mineralizing media. However, no attempt was made to clarify the type of the apatite formed in presence of a source of fluoride. It is thought that bioactive glass containing toothpaste can form fluoride-substituted apatite in the presence of soluble fluoride, since fluoride-containing bioactive glasses have been shown to form fluorapatite (FAP) following immersion in Tris buffer and simulated body fluid (SBF) . Therefore, the selection of the immersion media in this study was determined by the previous studies of bioactive glass powders, where typically Tris buffer, SBF or artificial saliva were used for testing. Instead of the SBF solution, the Earl’s salt solution with the defined concentration of (bi)carbonate was used. Thus, this selection of the immersion media enabled us to make a direct comparison of the results on the bioactive glass dissolution on its own, in presence of fluoride source and as a part of the toothpaste samples.
The objective of the present study was to investigate the type of apatite formed from the commercial bioactive glass-based toothpaste using the techniques of X-ray diffraction (XRD), Fourier Transformed Infrared (FTIR), 31 P and 19 F Magic Angle Spinning Nuclear Magnetic Resonance (MAS-NMR) spectroscopy and Inductively Coupled Plasma – Optical Emission Spectroscopy (ICP-OES). This is a lab based study conducted in several immersion media. However, the findings of the study are thought to have potential implications for the processes occurring intra-orally on application of the toothpaste. The null hypothesis tested in this lab-based study was that a fluoride free apatite forms as a result of the immersion of the bioactive glass and fluoride containing toothpaste.
2
Materials and methods
Fluorapatite formation capacity of Sensodyne Repair and Protect ® (SR&P, GlaxoSmithKline, UK) toothpaste (TP) was investigated in-vitro using three different buffer solutions; artificial saliva (AS), Tris buffer and Earle’s salt solution (ES). This commercial toothpaste contains bioactive glass (NovaMin™) as one of the therapeutic ingredients. Parallel experiments have been carried out for control experimental toothpastes, containing NovaMin™ but no fluoride (NovaMin™ Placebo, TP-BAG) and containing sodium monofluorophosphate (MFP) only (Fluoride Placebo, TP-MFP). The toothpaste samples studied are given in Table 1 . In addition to toothpaste samples, NovaMin™ powder (BAG) and a mixture of NovaMin™ powder and MFP (Na 2 FPO 3 , Sigma Aldrich) (BAG + MFP) were also investigated under the same conditions as the toothpaste samples.
Sample code | Sample description | Fluoride concentration, ppm | Novamin™ concentration, % w/w |
---|---|---|---|
TP | SR&P | 1450 | 5 |
TP-BAG | NovaMin™ Placebo | 0 | 5 |
TP-F | Fluoride Placebo | 1450 | 0 |
The composition of the AS solution (pH 6.87) is given in Table 2 ; the solution was supplied by Modus Laboratories Ltd. (Reading, UK). A fresh solution was prepared weekly and stored in the fridge before the use.
Ingredients | AS | ES |
---|---|---|
g/L | g/L | |
KCl | 2.236 | 0.400 |
CaCl 2 ·2H 2 O | 0.441 | 0.265 |
KH 2 PO 4 | 1.361 | – |
NaH 2 PO 4 ·H 2 O | – | 0.122 |
NaCl | 0.759 | 6.800 |
MgSO4 | – | 0.098 |
NaHCO3 | – | 2.200 |
Mucin (type II) | 2.200 | – |
D-Glucose | – | 1.000 |
Phenol Red(Na) | – | 0.011 |
Earle’s salt solution was prepared by diluting 100 ml of Earle’s balanced salt solution (Sigma Aldrich) 10 x to 1 L with distilled water and supplementing it with 2.2 g of sodium hydrogen carbonate (NaHCO 3 , Sigma Aldrich). The composition is given in Table 2 and a fresh solution was prepared daily.
Tris buffer was prepared by dissolving 15.090 g of tris(hydroxylmethyl)aminomethane (Sigma Aldrich) in 800 ml of distilled water followed by the addition of 44.2 ml of 1 M hydrochloric acid (HCl, Sigma Aldrich). Solution was kept at 37 °C overnight after which pH was adjusted to 7.30 using HCl and a pH meter (Oakton ® pH 11 m; 35811–71 pH electrode) and solution was filled to 2 L.
In order to hydrolyse the P-F bond of MFP and release free fluoride-ions to solution each buffer solution was supplemented with 5U/ml of bovine alkaline phosphatase (Sigma Aldrich). This concentration of the alkaline phosphatase was used in a previous study .
In immersion experiments, 150 mg of BAG powder or 3 g of toothpaste were dispersed in 100 ml of buffer solution (equivalent to 1.5 g/L powder to volume ratio) containing 5U/ml phosphatase. Samples were placed in an orbital shaker at an agitation rate of 60 rpm for various time points from 5 min to 4 days. After removing from the shaker samples were filtered through filter paper on a Buchner funnel using a water vacuum pump. A small volume of solution was filtered through 0.2 μm syringe filters for ion release analysis. Filtered samples were kept at 4 °C. Solid residues were dried in an oven at 37 °C and were analysed by 31 P and 19 F magic angle spinning nuclear magnetic resonance (MAS NMR), X-ray diffraction (XRD) and Fourier transform infrared (FTIR) spectroscopy.
31 P and 19 F MAS NMR spectra were recorded on a Bruker Avance 600 MHz spectrometer at resonance frequencies of 242.9 MHz and 564.7 MHz respectively. 31 P MAS NMR spectra were acquired in a 4 mm o.d. zirconia rotor at a spinning speed of 12 kHz, with a recycle delay of 60 s. A total of 16 or 32 scans were acquired. The concentrated solution of the orthophosphoric acid H 3 PO 4 (85%) was used as reference. 19 F MAS NMR spectra were acquired in a 2.5 mm rotor at a spinning speed of 21 kHz. Sodium fluoride 1 M aqueous solution producing a sharp signal at −120 ppm was used as a secondary reference against CFCl 3 .
The solid residue X-ray diffraction data were collected on a PANalytical X’Pert Pro diffractometer using Ni filtered Cu-Kα radiation (λ = 1.5418 Å). Data were recorded in a flat plate θ/θ geometry over the 2θ range 2 to 70° at a step width of 0.0334° with a count time of 200 s per step. Data were calibrated using an external LaB 6 standard. FTIR spectra were recorded on a Perkin Elmer spectrum 65 with an ATR attachment over a frequency range of 500–2000 cm −1 .
Solutions were analysed by inductively coupled plasma optical emission spectroscopy (ICP-OES, Varian Vista-Pro). The amount of F − -ion released into solutions was measured with fluoride-ion selective electrode (Orion pH/ISE meter 710, Orion 9609BNWP electrode). Individual calibration was performed using standard solutions diluted with respective buffer solutions to account for ionic strength. Ion release experiments were done in duplicates, though solid residues of duplicates were combined together to provide sufficient quantity of the samples for the characterisation.
2
Materials and methods
Fluorapatite formation capacity of Sensodyne Repair and Protect ® (SR&P, GlaxoSmithKline, UK) toothpaste (TP) was investigated in-vitro using three different buffer solutions; artificial saliva (AS), Tris buffer and Earle’s salt solution (ES). This commercial toothpaste contains bioactive glass (NovaMin™) as one of the therapeutic ingredients. Parallel experiments have been carried out for control experimental toothpastes, containing NovaMin™ but no fluoride (NovaMin™ Placebo, TP-BAG) and containing sodium monofluorophosphate (MFP) only (Fluoride Placebo, TP-MFP). The toothpaste samples studied are given in Table 1 . In addition to toothpaste samples, NovaMin™ powder (BAG) and a mixture of NovaMin™ powder and MFP (Na 2 FPO 3 , Sigma Aldrich) (BAG + MFP) were also investigated under the same conditions as the toothpaste samples.
Sample code | Sample description | Fluoride concentration, ppm | Novamin™ concentration, % w/w |
---|---|---|---|
TP | SR&P | 1450 | 5 |
TP-BAG | NovaMin™ Placebo | 0 | 5 |
TP-F | Fluoride Placebo | 1450 | 0 |
The composition of the AS solution (pH 6.87) is given in Table 2 ; the solution was supplied by Modus Laboratories Ltd. (Reading, UK). A fresh solution was prepared weekly and stored in the fridge before the use.
Ingredients | AS | ES |
---|---|---|
g/L | g/L | |
KCl | 2.236 | 0.400 |
CaCl 2 ·2H 2 O | 0.441 | 0.265 |
KH 2 PO 4 | 1.361 | – |
NaH 2 PO 4 ·H 2 O | – | 0.122 |
NaCl | 0.759 | 6.800 |
MgSO4 | – | 0.098 |
NaHCO3 | – | 2.200 |
Mucin (type II) | 2.200 | – |
D-Glucose | – | 1.000 |
Phenol Red(Na) | – | 0.011 |
Earle’s salt solution was prepared by diluting 100 ml of Earle’s balanced salt solution (Sigma Aldrich) 10 x to 1 L with distilled water and supplementing it with 2.2 g of sodium hydrogen carbonate (NaHCO 3 , Sigma Aldrich). The composition is given in Table 2 and a fresh solution was prepared daily.
Tris buffer was prepared by dissolving 15.090 g of tris(hydroxylmethyl)aminomethane (Sigma Aldrich) in 800 ml of distilled water followed by the addition of 44.2 ml of 1 M hydrochloric acid (HCl, Sigma Aldrich). Solution was kept at 37 °C overnight after which pH was adjusted to 7.30 using HCl and a pH meter (Oakton ® pH 11 m; 35811–71 pH electrode) and solution was filled to 2 L.
In order to hydrolyse the P-F bond of MFP and release free fluoride-ions to solution each buffer solution was supplemented with 5U/ml of bovine alkaline phosphatase (Sigma Aldrich). This concentration of the alkaline phosphatase was used in a previous study .
In immersion experiments, 150 mg of BAG powder or 3 g of toothpaste were dispersed in 100 ml of buffer solution (equivalent to 1.5 g/L powder to volume ratio) containing 5U/ml phosphatase. Samples were placed in an orbital shaker at an agitation rate of 60 rpm for various time points from 5 min to 4 days. After removing from the shaker samples were filtered through filter paper on a Buchner funnel using a water vacuum pump. A small volume of solution was filtered through 0.2 μm syringe filters for ion release analysis. Filtered samples were kept at 4 °C. Solid residues were dried in an oven at 37 °C and were analysed by 31 P and 19 F magic angle spinning nuclear magnetic resonance (MAS NMR), X-ray diffraction (XRD) and Fourier transform infrared (FTIR) spectroscopy.
31 P and 19 F MAS NMR spectra were recorded on a Bruker Avance 600 MHz spectrometer at resonance frequencies of 242.9 MHz and 564.7 MHz respectively. 31 P MAS NMR spectra were acquired in a 4 mm o.d. zirconia rotor at a spinning speed of 12 kHz, with a recycle delay of 60 s. A total of 16 or 32 scans were acquired. The concentrated solution of the orthophosphoric acid H 3 PO 4 (85%) was used as reference. 19 F MAS NMR spectra were acquired in a 2.5 mm rotor at a spinning speed of 21 kHz. Sodium fluoride 1 M aqueous solution producing a sharp signal at −120 ppm was used as a secondary reference against CFCl 3 .
The solid residue X-ray diffraction data were collected on a PANalytical X’Pert Pro diffractometer using Ni filtered Cu-Kα radiation (λ = 1.5418 Å). Data were recorded in a flat plate θ/θ geometry over the 2θ range 2 to 70° at a step width of 0.0334° with a count time of 200 s per step. Data were calibrated using an external LaB 6 standard. FTIR spectra were recorded on a Perkin Elmer spectrum 65 with an ATR attachment over a frequency range of 500–2000 cm −1 .
Solutions were analysed by inductively coupled plasma optical emission spectroscopy (ICP-OES, Varian Vista-Pro). The amount of F − -ion released into solutions was measured with fluoride-ion selective electrode (Orion pH/ISE meter 710, Orion 9609BNWP electrode). Individual calibration was performed using standard solutions diluted with respective buffer solutions to account for ionic strength. Ion release experiments were done in duplicates, though solid residues of duplicates were combined together to provide sufficient quantity of the samples for the characterisation.
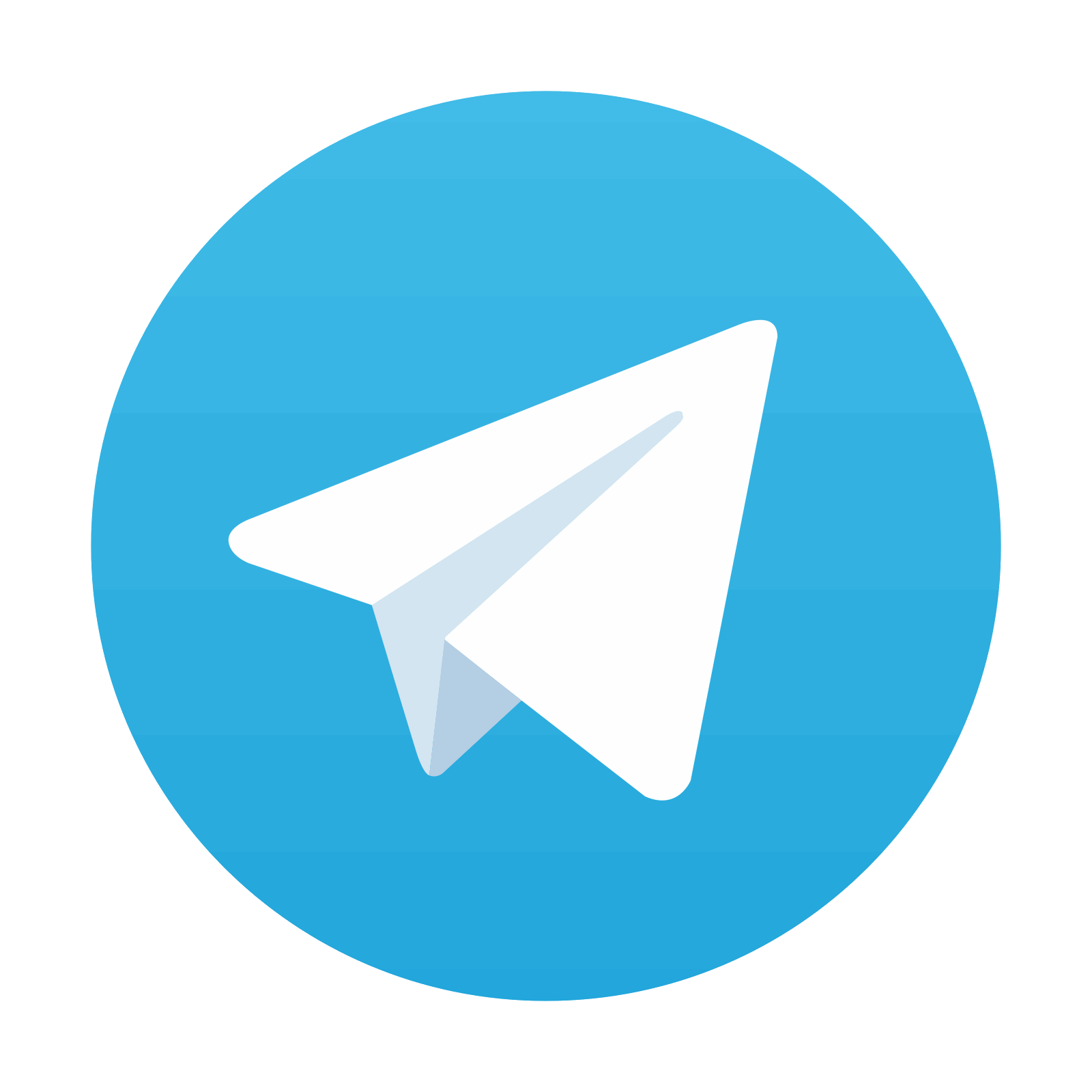
Stay updated, free dental videos. Join our Telegram channel

VIDEdental - Online dental courses
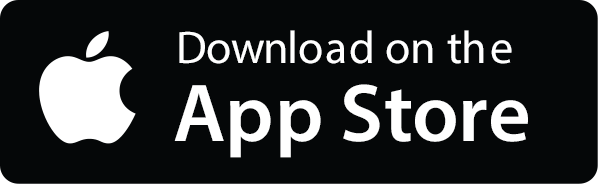
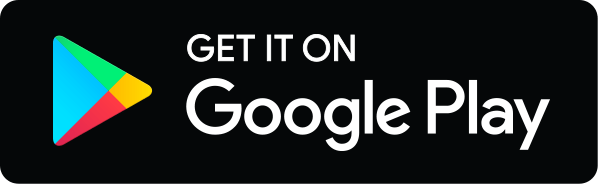