Introduction
This experiment was undertaken to assess the primary stability of orthodontic miniscrews inserted at different sites in human cadaveric palatal bone for temporary skeletal anchorage, and to determine the effect of bone quality and quantity on their primary stability using microcomputed tomography imaging.
Methods
A total of 10 cadaveric maxillary hard palates were used for insertion of 130 orthodontic miniscrews (VectorTAS; Ormco, Orange, Calif; length, 6 mm; diameter, 1.4 mm). Upon insertion, maximal insertion torque (IT) was recorded. Imaging (microcomputed tomography) was performed before and after insertion for assessment of bone quality and quantity parameters (bone mineral density [BMD], bone thickness [BT], and length of screw engagement [LSE]). Differences in each parameter were assessed at the various insertion sites. Correlations between IT and measurements of BMD, BT, and LSE were evaluated.
Results
Significant differences ( P < 0.001) were found among insertion sites for IT, BT, and LSE, but not for BMD ( P = 0.004). Correlations were found between IT and BMD ( r s = 0.42; P < 0.001), IT and BT ( r s = 0.58; P < 0.001), and IT and LSE ( r s = 0.58; P < 0.001). Most perforations of miniscrews into the nasal cavity occurred posterior to the permanent second premolars.
Conclusions
The primary stability of orthodontic miniscrews in the palate is affected by bone quality and quantity, with higher primary stability obtained anterior to the second premolars and parasagittally at the level of the permanent first molars.
Highlights
- •
Primary stability of orthodontic miniscrews depends on hard palate insertion site.
- •
Primary stability was highest parasagitally and anterior to second premolars.
- •
Primary stability was moderately affected by both bone quality and quantity.
- •
An increased risk of nasal cavity perforation was found posterior to second premolars.
The use of orthodontic miniscrew implants as temporary anchorage devices (TADs) has expanded the possibilities in orthodontic treatment. With the objective of facilitating difficult tooth movements and potentially avoiding orthognathic surgery, miniscrews are used temporarily during treatment to achieve skeletal anchorage and subsequently removed. For that purpose, their surface is designed to prevent osseointegration, and they are primarily retained through mechanical interlocking in bone.
The clinical success of miniscrews is directly related to their stability after placement, or “primary stability,” and depends on local bone properties, the engineering design of the screw, and the placement technique used. Different tests and devices are available to assess the stability of miniscrews. Among the most common indirect measurements for primary stability is the moment of the force required to screw the miniscrew into the bone, referred to as insertion torque (IT). The IT is influenced by bone properties, whereby denser and thicker bone is associated with increased torque required for screw insertion.
Orthodontic miniscrews are commonly inserted into the buccal cortical plate in the maxilla and mandible, the palatal aspect of the maxillary alveolar process, the hard palate of the maxilla, and the retromolar area in the mandible. However, the hard palate has significant mechanical and anatomic advantages over the other insertion sites. It is surgically accessible, offers excellent peri-implant conditions due to the attached mucosa, provides reduced hindering of tooth movement during treatment, and allows versatility in appliance design. The associated disadvantages are the possible lack of vertical bone thickness and its anatomic variability among patients. Other cited concerns include perforation into the nasal or sinus cavity, interference with the incisive canal, possible effect on the midpalatal suture in growing patients, and the need for more profound anesthesia due to the tightness of the soft tissue.
Palatal bone quantity for miniscrew placement was investigated in a systematic review by Winsauer et al. It was suggested that the region 3 to 4 mm behind the incisive foramen and 3 to 9 mm lateral to the midpalatal suture should provide sufficient vertical bone height for anchors. Current knowledge regarding bone mineral density (BMD) in the maxillary hard palate is limited, with 1 study noting that it decreases from anterior to posterior and from medial to lateral. Ludwig et al considered both palatal hard and soft tissues, as well as blood vessels and nerves, with the objective of providing placement guidelines with regard to anatomic limitations. They suggested a safety zone that was a T shape, where the horizontal portion lies along the lingual cusps of the permanent first premolar and the vertical portion extends posteriorly to the level of the permanent first molars.
Overall, the maxillary hard palate appears to be an attractive site for miniscrew insertion, where good primary stability might be expected. However, to our knowledge, there are currently no reported data on the primary stability of palatal miniscrews. Therefore, the purpose of this study was to measure the primary stability (IT) of inserted orthodontic miniscrews at different regions of the maxillary hard palate in human cadavers and to determine the effects of bone quality (BMD) and quantity (bone thickness [BT]) and length of screw engagement (LSE) on primary stability using microcomputed tomography (μCT) imaging.
Material and methods
A grid system was created for identification of palatal sites used for bone analysis and stability testing ( Fig 1 ). The rationale was to include the most commonly reported palatal insertion sites, as well as those that could be used for miniscrew insertion, without encroaching on important anatomic structures such as nerves and blood vessels. The midpalatal suture was excluded from analysis due its variability and possible effect on growth in young patients. In the custom-designed grid system, the green region represents the currently recommended areas for safe placement ( Fig 1 ).

The 0,0 coordinate of the grid system represents the posterior border of the incisive foramen at the midpalatal suture. X and y axes were constructed from this reference point. The y-axis ran through the midpalatal suture, and the x-axis was tangent to the posterior border of the incisive foramen, perpendicular to the y-axis. The x-axis was coincident with the distal aspect of the maxillary permanent canines as described previously.
Parallel to both the x and y axes, the anteroposterior and mediolateral directions were created. In the anteroposterior direction, the initial line was 3 mm posterior to the x-axis, with subsequent lines in increments of 6 mm from the initial line, to correspond with dental landmarks ( Fig 1 ). A numeric order (1-5) was given to each line according to its proximity to the incisive foramen. For the mediolateral direction, the initial lines were 2 mm lateral to the y-axis, with subsequent lines located in 4-mm increments from the initial lines. These lines were named according to their proximity to the midpalatal suture, with the closest termed the parasagittal line, the middle termed the sagittal line, and the farthest termed the lateral line.
To test all intersection points of the grid system and to preserve an acceptable distance between the miniscrews for testing, the insertion sites were staggered between the right and left sides of each specimen ( Fig 1 ). This technique was deemed acceptable, since Gracco et al demonstrated no significant difference in BT between contralateral sides of the palate for the same subject. A total of 13 sites were tested on each specimen.
All palatal specimens were obtained with permission from the body-bequeathal program at Western University, London, Ontario, Canada, in accordance with the Anatomy Act of Ontario and Western’s Committee for Cadaveric Use in Research. A total of 10 embalmed cadaveric heads were used ( Table I ). Sex of the specimens was not a specific requirement because Gracco et al showed no statistically significant difference in palatal BT between sexes. Age at death was also not a specific criterion due to the limited number of specimens available. For inclusion in this study, the specimens were required to have an intact and complete (right and left) maxillary palatal bone, full or partial dentition with at least 4 anterior teeth (to ensure comparable incisive canal outlines), and no palatal torus and bony pathology involving the maxilla.
Specimen | Sex | Age (y) | Number of teeth | Torus | Cause of death | ||
---|---|---|---|---|---|---|---|
Total | Anterior | Posterior | |||||
1513 | M | 78 | 7 | 4 | 3 | N | Cardiac arrest, pulmonary edema, Cardiogenic shock, myocardial infarction, chronic renal failure |
1517 | M | 86 | 9 | 6 | 3 | N | Myocardial infarction, CAD, CHF, acute renal failure |
1576 | M | 57 | 14 | 6 | 8 | N | Prostate cancer |
1589 | M | 98 | 12 | 6 | 6 | N | ASHD, atrial fibrillation, CHF, peripheral vascular disease |
1605 | M | 54 | 13 | 6 | 7 | N | Aspiration pneumonia, Huntington’s chorea |
1615 | M | 80 | 6 | 5 | 1 | N | Complications of lung injury from MVC, Pneumonia, ARDS, rib fracture, Pneumothorax, pulmonary embolus, CHF, CAD |
1672 | F | 93 | 7 | 5 | 2 | N | End stage dementia, CVA, hypertension |
1683 | F | 93 | 12 | 6 | 6 | N | Cardiorespiratory failure, CHF |
1706 | M | 61 | 6 | 6 | 0 | N | Hepatic failure, alcoholic liver cirrhosis |
1719 | F | 76 | 13 | 6 | 7 | N | Pneumonia, pulmonary fibrosis, Methotrexate usage, giant cell arteritis |
The embalmed heads were cut so that the maxilla was separated from the rest of the skull. All soft tissues were dissected to eliminate specimen variability and standardize the LSE during miniscrew placement. The crowns of the teeth were subsequently removed, eliminating any metal substance from dental fillings that could interfere with x-ray imaging.
The specimens were stabilized by embedding in standardized acrylic blocks with the teeth facing superiorly for miniscrew insertion testing. The acrylic level was carefully controlled, leaving approximately 1 cm of clearance below the nasal floor to allow assessment of miniscrew penetration into the nasal cavity and to prevent interferences during insertion. A stencil with the predesigned grid pattern (described above) was custom fabricated and used for accurate reproduction of the grid system onto the specimens.
One hundred thirty VectorTAS orthodontic miniscrews (Ormco, Orange, Calif; length, 6 mm; diameter, 1.4 mm) were used for insertion at the predetermined sites (n = 10). All miniscrews were self-drilling; thus, predrilling of the cortical bone was not required.
To replicate the average palatal soft tissue thickness as determined by Vu et al, a 1-mm silicone spacer was installed over the soft tissue collar of each miniscrew before insertion ( Fig 2 ), leaving 5 mm of the body length available for insertion into bone. The miniscrews were manually inserted by the first author (C.B.), simulating the typical clinical technique. The corresponding peak torque value reached during miniscrew insertion was recorded in Newton-centimeters (Ncm).

The insertion device consisted of a screwdriver with a chuck, which secured the manufacturer’s specific driver adaptor and engaged the miniscrew head ( Fig 2 ). The screwdriver handle was held in place by a stabilizing bar, which was specifically designed to support the driver shaft and prevent oblique forces during manual screw placement. This allowed the miniscrews to be inserted vertically, without introducing off-axis loading along their length.
For insertion of the miniscrews, the embedded specimens were fixed at the base of the insertion device within a custom adaptor and secured onto the torque sensor (6 DOF load cell; Advanced Mechanical Technology, Watertown, Me). A multiaxis clamp was used to position the specimens at the center of a torque sensor and adjusted as required to ensure perpendicular insertion of the miniscrew to the bone surface. The torque sensor and associated software program (WaveMatrix Software; Instron, Norwood, Mass) measured and recorded torque values during miniscrew insertion. Calibration of the sensor was completed before insertion of each miniscrew to reduce any systematic errors in the setup.
Specimens were imaged using a cone-beam μCT scanner (eXplore Locus Ultra; GE Healthcare, Milwaukee, Wis) before and after miniscrew insertion. The scanner acquires 1000 projection images (1024 × 680 matrix size), over a single 16-second rotation at 120 kV(p) and 20 mA. These projection images were back-projected to reconstruct a 1024 × 1024 × 680 voxel 3-dimensional volume, with 150-μm isotropic voxel spacing, and scaled in Hounsfield units (HU) using known water and air regions. Du et al previously described the scanner characteristics. Included with each scan were 2 known density calibrators (water [0 mgHA/cm −3 ] and a bone-simulating material SB3 [1073mgHA/cm −3 ]).
Image analysis was performed using 3-dimensional analysis software (MicroView 2.2; GE Healthcare, London, Ontario, Canada). BMD and BT were calculated using coregistered scans and the miniscrew trajectory into bone, as described by Sabo et al. Custom software was used to create 2-mm diameter cylindrical regions of interest within the bone and around the miniscrew trajectory, with length specified by the screw length. The cylindrical regions of interest were superimposed onto the original (preinsertion) scans ( Fig 3 , A ) and used for BMD analysis. Voxels with values of −200 HU or less were excluded from BMD calculations and assumed to represent air. Voxels with values between –200 and –50 HU were forced to have a value of 0 mgHAcm −3 , since these values would correspond to water, fat, or preservation medium.

For BT measurements, grayscale line profiles were created following each longitudinal miniscrew trajectory (described above) and ensured to go through the entire bone length. These profiles generated plots of grayscale values (HU) as a function of line position (mm) and allowed for thickness measurements. Using a full width at half maximum technique, grayscale transitions from air to bone and bone to air were used to identify the entrance and exit of each miniscrew ( Fig 3 , B ).
Miniscrew perforations into the nasal cavity were identified and confirmed by visual inspection of the specimens ( Fig 4 ). Perforations were only observed in the nasal cavity and not in the maxillary sinus, since the miniscrews were inserted into the hard palate proper and not the alveolar bone where the sinus cavities are located ( Fig 3 , A ). Using the μCT data, subtraction of the miniscrew threaded body length (5 mm) from the BT showed the magnitude of the perforation (negative value). Perforations of more than 1 mm were judged to be clinically significant and recorded.

Statistical analysis
Descriptive and inferential statistics were determined for the different insertion sites using the Statistical Package for the Social Sciences software (version 22.0; IBM, Armonk, NY). The Kruskal-Wallis nonparametric test ( P < 0.05) was used to compare the differences in the median IT, BMD, BT, and LSE values at the different insertion sites. Pairwise comparisons were performed using the Dunn procedure with the Bonferroni correction for multiple comparisons (differences were significant at P < 0.004). The association between the individual insertion sites and the frequency of perforations was assessed using the Fisher exact test ( P < 0.05). Finally, the influence of BMD, BT, and LSE on the measures of IT was calculated using the Spearman rank-order correlation test.
Results
Descriptive statistics representing the IT, BMD, BT, LSE, and perforation values for each insertion site are given in Table II . Statistical analysis showed that IT, BT, and LSE data were not normally distributed. BMD was normally distributed, but outliers were detected. The median values were therefore interpreted ( Fig 5 ).
Insertion site | n | BMD (mg/cc) | BT (mm) | LSE (mm) | IT (Ncm) | Perf (n/10) | ||||||||
---|---|---|---|---|---|---|---|---|---|---|---|---|---|---|
Median | Mean | SD | Median | Mean | SD | Median | Mean | SD | Median | Mean | SD | |||
PS1 | 10 | 318.77 | 338.03 | 104.1 | 7.58 | 7.88 | 3.17 | 5.00 | 4.63 | 1.17 | 13.05 | 16.48 | 8.15 | 1 |
S1 | 10 | 303.12 | 283.88 | 84.10 | 9.35 | 8.82 | 2.74 | 5.00 | 4.68 | 1.02 | 15.45 | 15.53 | 4.41 | 1 |
PS2 | 10 | 381.14 | 373.67 | 109.94 | 4.42 | 4.68 | 1.27 | 4.42 | 4.33 | 0.69 | 9.75 | 9.39 | 3.94 | 4 |
S2 | 10 | 408.68 | 410.50 | 103.99 | 3.73 | 3.65 | 1.69 | 3.73 | 3.45 | 1.37 | 15.15 | 14.68 | 7.25 | 6 |
L2 | 10 | 300.43 | 321.39 | 91.29 | 6.16 | 5.58 | 2.07 | 5.00 | 4.41 | 1.20 | 14.50 | 14.44 | 6.42 | 1 |
PS3 | 10 | 416.41 | 426.59 | 123.32 | 3.15 | 3.42 | 0.98 | 3.15 | 3.42 | 0.98 | 13.10 | 13.83 | 3.91 | 7 |
S3 | 10 | 422.77 | 441.99 | 134.13 | 1.96 | 1.78 | 0.81 | 1.96 | 1.78 | 0.81 | 9.00 | 12.27 | 6.99 | 10 |
L3 | 10 | 384.29 | 393.18 | 150.65 | 2.46 | 2.64 | 1.54 | 2.46 | 2.59 | 1.43 | 8.55 | 11.16 | 7.43 | 8 |
PS4 | 10 | 519.49 | 482.88 | 135.96 | 3.50 | 3.71 | 1.18 | 3.50 | 3.64 | 1.07 | 14.55 | 13.86 | 5.57 | 6 |
S4 | 10 | 337.69 | 319.02 | 144.33 | 1.31 | 1.31 | 0.52 | 1.31 | 1.31 | 0.52 | 8.10 | 8.63 | 4.86 | 10 |
L4 | 10 | 256.67 | 271.01 | 134.14 | 1.04 | 1.27 | 0.67 | 1.04 | 1.27 | 0.67 | 7.05 | 7.05 | 3.60 | 10 |
PS5 | 10 | 435.01 | 421.42 | 103.70 | 2.54 | 2.47 | 0.94 | 2.54 | 2.47 | 0.94 | 6.75 | 8.19 | 5.13 | 10 |
S5 | 10 | 314.04 | 311.93 | 95.48 | 0.96 | 1.12 | 0.44 | 0.96 | 1.12 | 0.44 | 4.65 | 5.38 | 2.84 | 10 |
P value | 0.004 | <0.001 ∗ | <0.001 ∗ | <0.001 ∗ | <0.001 ∗ |
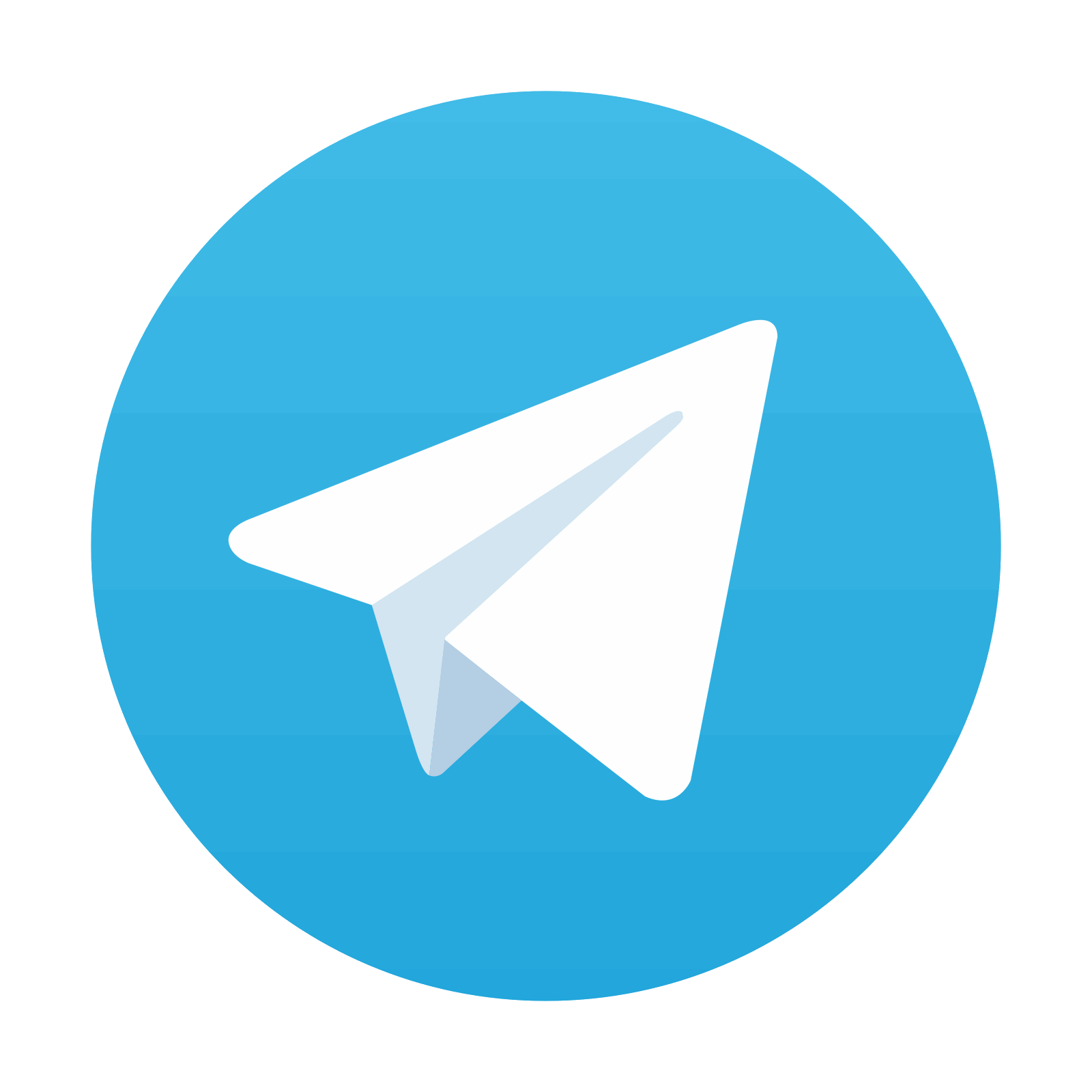
Stay updated, free dental videos. Join our Telegram channel

VIDEdental - Online dental courses
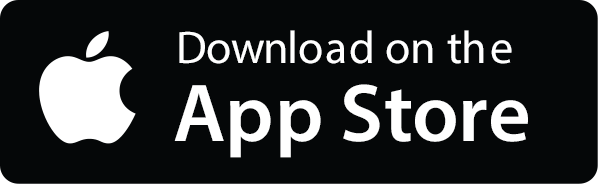
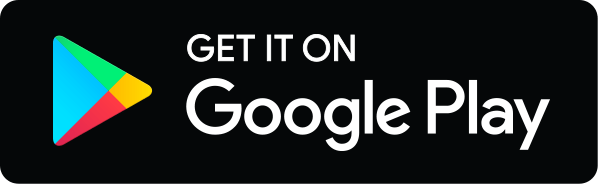
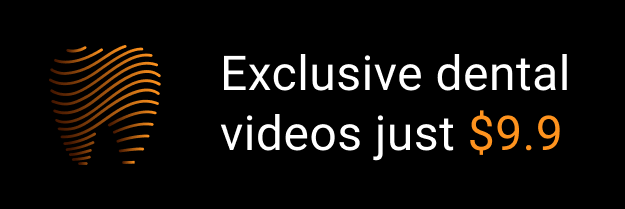