Abstract
Objectives
The aim of the present study was to compare, in situ , the initial dental plaque formation on a recently developed silorane-based composite resin, Filtek Silorane, and on a widely used methacrylate-based composite resin, Synergy D6, and to relate possible differences to surface free energy, hydrophobicity and type of organic matrix.
Methods
Discs of Filtek Silorane and Synergy D6 were prepared and polished equally in order to attain the same surface roughness. Water, formamide and 1-bromonaphthalene contact angles were determined and the surface free energy and the hydrophobicity of the materials calculated. Two discs of each material were mounted in individual oral splints and exposed to the oral cavity of 20 participants for 4 h. After this period the microbial adhesion to both materials’ surface was measured by two different approaches, the DAPI staining and the plate count. Statistical analysis was performed using non-parametric tests.
Results
The surface roughness ( R a parameter) was similar between the two materials and lower than 0.2 μm. Mean water and formamide contact angles were significantly higher for Filtek Silorane, which presented significantly lower surface free energy and greater degree of hydrophobicity in comparison to Synergy D6. The bioadhesion potential evaluated by either DAPI staining or plate count did not differ between the two materials.
Significance
In contrast to previous in vitro studies, the present in situ study found no statistically significant differences with respect to bacterial adhesion between Filtek Silorane and Synergy D6, despite the differences found for surface free energy and hydrophobicity.
1
Introduction
Dental composite resins are an esthetic alternative to dental amalgam introduced in the 1960/1970s and have been successfully used for the last decades . Despite the innovative improvements along the years, methacrylate-based restorations can still fail for a varied number of reasons, but most frequently by secondary caries . Accordingly, 50% of direct tooth restorations are replacements , which emphasize the clinical relevance of bacterial adherence to dental restorations. In this sense, bioadhesion properties of dental restorative materials are considered to be of great importance for their longevity in the oral cavity . The polymerization shrinkage of the widely used methacrylate-based composites is stated to be their main drawback given that the volumetric contraction occurring during polymerization results in marginal gaps between the material and the cavity walls . The biofilm developed in such fissures is very difficult to control and may be the primary cause of composite-associated caries. In addition, it has been stated that low roughness and low-energy surfaces are two fundamental properties of restorative materials in reducing bioadhesion in the oral cavity . Recently, a new monomer that combines siloxane and oxirane, silorane, was introduced . Silorane-based composite resins exhibit similar or better mechanical and physical properties than conventional methacrylate-based composite resins , namely, low polymerization shrinkage (less than 1.5%) , lower polymerization shrinkage stress which induces less cuspal displacement , lower water sorption combined with decreased water solubility , stability and insolubility in various aqueous systems , good biocompatibility characteristics with low mutagenic potential , low potential to absorb the dyes of daily nutrition due to its hydrophobic nature , better overall color stability over time , higher ambient light stability , pleasing short-term optical properties and better cavity adaptation .
Two studies evaluated silorane-based composite susceptibility to bacterial adhesion in vitro , suggesting that these novel composites have lower susceptibility to adhere oral streptococci than conventional methacrylate-based composite resins. Generally, in in vitro studies, there is a great influence of different physical surface properties on bioadhesion, being surface roughness the main responsible for bacterial adhesion and biofilm formation . However, in vitro studies do not always reflect bioadhesion in vivo , given that in the oral cavity, the oral biofilm formation starts by bacterial adhesion to the acquired pellicle, which covers all oral surfaces, including dental restorations, and the pellicle formation masks the physicochemical surface properties of dental materials to a certain extent . Notwithstanding, in in situ or in vivo studies, it should be taken into account the high intra-individual and inter-individual variability and different modulating factors such as varying salivary flow, nutrition and oral bacteria .
Given the above mentioned, the aim of the present study was to compare, in situ , the initial dental plaque formation on a recently developed silorane-based composite resin and on a widely used methacrylate-based composite resin, and to relate possible differences to surface free energy, hydrophobicity and type of organic matrix. The null hypothesis was that there are no differences between the dental restorative materials studied regarding oral microorganisms adherence susceptibility.
2
Materials and methods
2.1
Materials
The commercially available and widely used methacrylate-based composite resin, Synergy D6 (Coltène/Whaledent AG, Genf, Switzerland, Lot nr. 0206616) and the new silorane-based composite resin, Filtek Silorane (3M ESPE, St. Paul, MN, USA, Lot nr. 131585) were used in this study. Table 1 lists general composition of the tested materials. Sixty disc-shaped specimens (10 mm in diameter and 2 mm in height) were made from each material. Each disc was prepared similarly to what has been previously described , and according to the manufacturer’s instructions, using metallic molds with calibrated circular holes. The materials were inserted in the mold and then covered with a glass slide to minimize the formation of an oxygen-inhibited layer. All specimens were light-polymerized for 40 s through the glass slide using a LED (Light Emitting Diode) polymerization light (R&S Easylight, Litdent, Kedainiai, Lithuania, 1000 mW cm −2 ). Each disc was polished using a polishing machine (DAP-8, Struers, Cleveland, USA), wet abrasive paper discs (Wetordry, 3M ESPE, St. Paul, MN, USA) with grit of 1000 and 2000 and polishing diamond spray (Kemet Diamond Spray, Kemet International Ltd., Kent, United Kingdom, 3 μm) in order to achieve a similar degree of surface roughness in all specimens. After preparation of the discs they were disinfected by ultrasonication for 15 min in 70% ethanol, washed twice in sterile distilled water and stored in aseptic environment before exposure in the oral cavity. The discs used for contact angle determination were stored overnight in a dessicator to improve the dryness of the material, as described in previous studies .
Composite resin | Organic matrix | Filler |
---|---|---|
Filtek Silorane | Silorane (3,4-epoxycyclohexylethylcyclopolymethylsiloxane, bis-3,4-epoxycyclohexylethylphenylmethylsilane) | Silanized quartz, yttrium fluoride, 76 wt% |
Synergy D6 | Bis-GMA, TEGDMA, UDMA | Barium glass, amorphous silica, 80 wt% |
2.2
Contact angles, surface free energy and hydrophobicity determination
The determination of the contact angles of at least three different liquids with known surface tension components is needed to determine the surface free energy of a solid surface . So, the contact angles of distilled water, formamide and 1-bromonaphthalene were measured in both materials using a computerized contact angle system (OCA 15, Dataphysics Instruments, Filderstadt, Germany) and an adequate computer software for image analysis and contact angle determination (SCA 20, Dataphysics Instruments) as described previously . The contact angles were determined at 20 °C and according to the sessile drop technique. Five discs of each material were used with each liquid. Two calibrated droplets (4.0 μL) of distilled water and one calibrated droplet of formamide and 1-bromonaphthalene were analyzed on each of the respective discs. An image of each droplet was taken immediately after contact with the disc’s surface and two measures for each droplet were performed (right and left contact angle).
The mean values of the three liquids’ contact angles ( ) were used to calculate the surface free energy of each material, using the approach of van Oss et al. . Using the Lifshitz–van der Waals acid–base (LWAB) equation (Eq. (1) ) and the data of surface tension components of the test liquids ( γ l ) given in Table 2 it was possible to determine the materials’ Lewis acid ( γ + ) and Lewis base ( γ − ) surface free energy components, non-polar ( γ LW ) and polar (γAB=2√γ−γ+)(γAB=2γ−γ+−−−−−√)
( γ AB = 2 γ − γ + )
components, as well as the total surface free energy ( γ TOT = γ LW + γ AB ).
( 1 + cos ) γ 1 = 2 [ γ s LW γ 1 LW + γ s + γ 1 − + γ s − γ 1 + ]
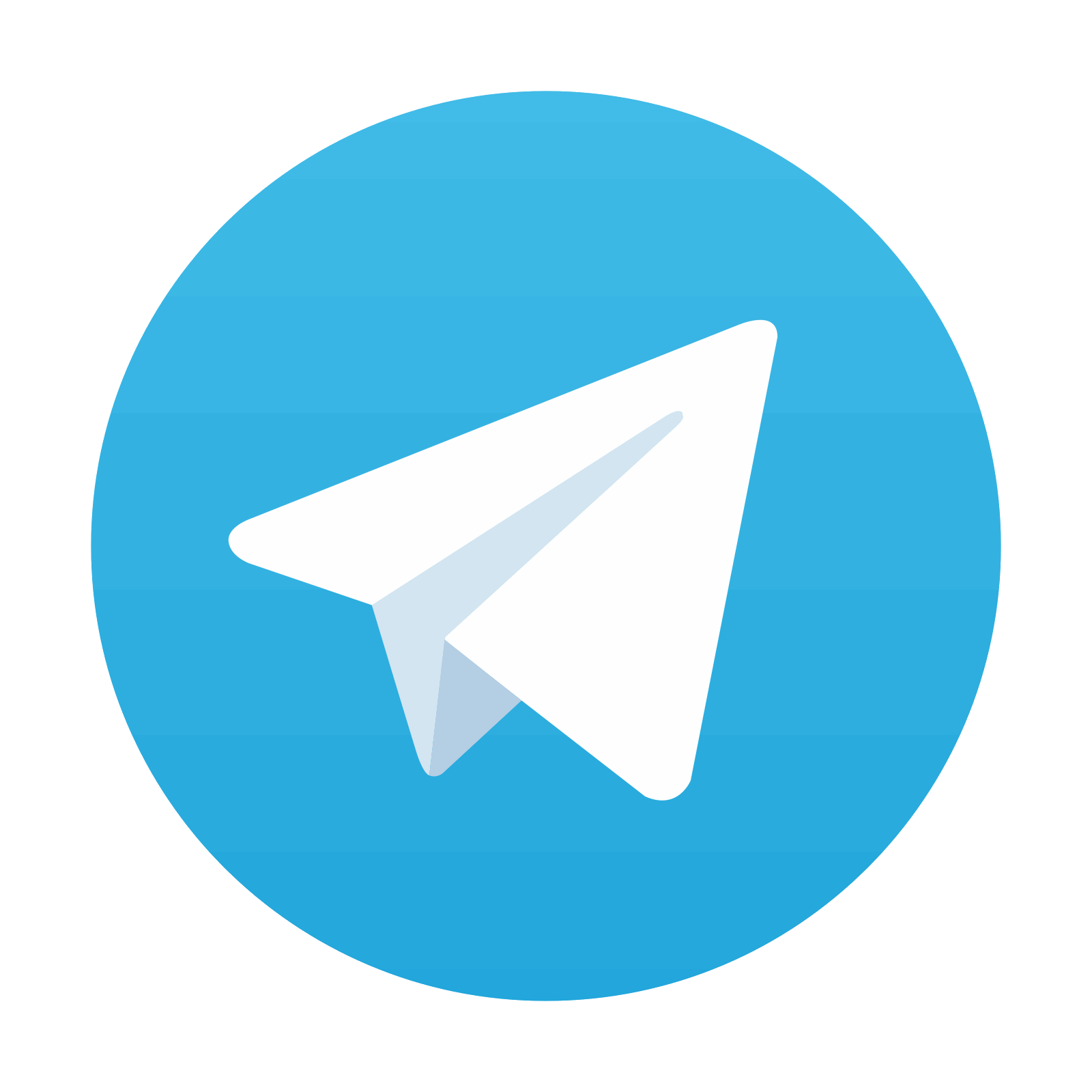
Stay updated, free dental videos. Join our Telegram channel

VIDEdental - Online dental courses
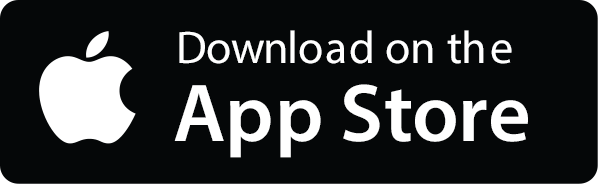
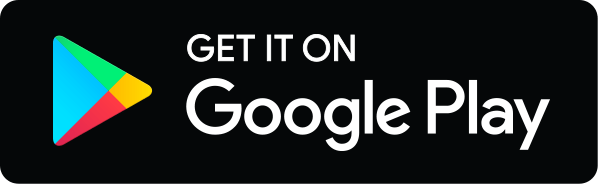