div epub:type=”chapter” role=”doc-chapter”>
P. Jain, M. Gupta (eds.)Digitization in Dentistryhttps://doi.org/10.1007/978-3-030-65169-5_12
12. Dental Implants and Digitization
Dental implantsNavigationCAD/CAM
12.1 Introduction
The last couple of decades have seen a tremendous increase in the use of computer-driven technology to automate several processes in the dental industry. The use of computer-aided design (CAD) and computer-aided manufacturing (CAM) have transformed the process of design, manufacture, and delivery of many dental services [1]. Restorative dentistry has probably been the largest gainer of this transformation. Dental implantology however comes a close second and digital technology has brought dental radiology, implant surgery, and implant prosthodontics together in a manner that few could have envisioned possible.
12.2 History and Development
In the late 1980s, the osseointegrated root-form implant began to see extensive clinical use as a modality to replace teeth. Professor Branemark had completed several years of clinical research that saw the modality become popular clinically [2]. The root-form implant held several geometric advantages over other forms of previously practiced endosseous implants such as blade implants and ramus frames. A cylindrical shape with a screw thread of definitive diameter and length quickly became the most popular. The geometry of this type of implant lent itself very easily to large-scale commercial manufacturing.
Several companies began to manufacture implants using computer numeric controlled (CNC) lathes which are still the mainstay of the industry. These CNC lathes themselves became extremely accurate and in time were able to manufacture extremely high accuracy tolerances. This allowed for excellent componentry fit and restorative predictability (R). Once endosseous root-form implants had established themselves as an accepted modality of treatment, the industry began to face challenges with clinical demands for restorative customization. A growing esthetic demand for implant restorations to be as natural as possible drove this increased demand for customization as well. The industry stepped up to the challenge and invested in CNC manufacturing of customized restorative componentry. The early 2000s saw the creation of large facilities dedicated to CAD/CAM design of custom abutments, restorations, and other restorative componentry such as bars [3]. These facilities provided multilocation high-end computer-aided manufacturing and served to eliminate expensive investment in the equipment required.

Customized angled overdenture design
The development of cone beam computerized tomography at around the same time also made three-dimensional radiographic visualization of anatomy a reality [8, 9]. It remained up to the industry to marry these digital technologies together and develop a protocol for more accurate surgery.
Much research in the late 90s and early 2000s eventually saw the development of guided implant surgery. The development of surgical guides and instrumentation that allowed accurate preparation of bone for a dental implant became very popular over time [10, 11]. Several dentists with a moderate surgical skill set as well as clinicians demanding greater planning accuracy for complex cases, became supporters of guided implant surgery. The surgical guides themselves were made at the time in centralized CAM facilities that employed rapid prototyping technology [12, 13]. The gradual erosion of the cost of computer-aided manufacturing, both in milling and rapid prototyping, eventually made the consideration of chair-side use of these technologies a reality.

A safe guide with lateral access from Simplant
Software business models that had once focused on centralized manufacturing for a fee, have now largely become low cost or even free, sometimes allowing a cost to be incurred only when needed. We are therefore at a very exciting juncture with regard to the digitization of implant dentistry and the future is bright.
This chapter seeks to chronologically elaborate on several aspects of this digitization process that have come to be a reality today. The idea is to educate the reader about multiple digital technologies that are currently available with regard to multiple aspects of the delivery of clinical implant dentistry.
12.3 Implant Manufacturing

Azento concept all-inclusive implant solution
12.4 Restorative Componentry
The late eighties saw the development of restorative CAD and CAM technologies in Europe. Companies began to be able to manufacture custom restorations in ceramics and custom componentry in titanium. Large industry players moved to acquire this technology and the nineties saw the introduction of either centralized manufacturing facilities for restorations or their componentry or the advent of early chair-side milling solutions [14, 26–28]. Computer-aided designing came to start to replace what was traditionally handcrafted by dental technicians. The quality of custom implant componentry actually began to enhance restorative outcomes. Dental technicians themselves moved to automate their own design manufacturing processes and sought to outsource much of what they produced to large centralized manufacturing facilities that invested in multimillion-dollar multiaxis milling machines. Manufacturing capabilities of these machines began to include full arch restorations in zirconia and titanium as well, including full-arch bridges, titanium substructures, and titanium bars.
The quality and passivity of fit even in cross-arch situations were often exemplary and computer-aided manufacturing helped address many of the weaknesses of conventional restorative manufacturing. Many cross-arch cast restorative solutions were heavy, expensive, and sometimes inaccurate [29, 30]. Milled single- and multiple-unit restorative componentry slowly began to become the mainstay of the implant industry [31, 32]. The real advances in the manufacture of restorative componentry however began to come when manufacturing began to become decentralized. Computer-aided manufacturing technologies gradually became more portable and the industry began to develop desktop milling solutions that returned control to the dental technician. Dental technicians themselves needed to adapt to technological change and move to learn and use digital software to replace conventional manufacturing. Multiaxis manufacturing lathes are now easily available as desktop solutions and mill ceramics, zirconia, titanium, cobalt chrome, wax, techno polymers, acrylics, and composite [33, 34]. The wide range of capability of these machines has also meant that dental laboratories have become extensively digitized.
Chair-side milling technology has also seen significant change over the last decade. Dentists are able to provide chair-side milling solutions in ceramics, composite, and zirconia that often see them able to deliver restorations in one appointment. Newer milling machines are also capable of milling titanium abutments and non-precious metal restorations, giving today’s digital dentist many more additions to his clinical repertoire [35–37]. Chair-side design technologies have also put the clinician more in power of single-unit restorations, in particular, giving a dentist the ability to design crowns, veneers, implant abutments, implant restorations, and inlays and onlays. The sheer range of materials that have become available for chair-side milling is also impressive with materials such as composites, feldspar ceramics, leucite reinforced ceramics, ceramic-composite hybrids, zirconia, acrylics, non-precious alloy and lithium silicates/disilicates/zirconia reinforced silicates, all being available in the form of blocks that are accepted by chair-side mills. Advances have also included the ability of these machines to dry mill and wet mill, thus reducing processing times for post milling sintering or crystallization [38, 39].
Technologies in furnace development have also kept pace and provided dentists with chair-side solutions that are capable of an extremely rapid rise in sintering/crystallization temperatures as well as rapid cooling. The fastest mills are capable of milling some materials in less than 5 min with sintering/crystallization taking only another 10 min [40, 41]. These technologies have therefore resulted in the creation of chair-side restorations while the patient waits. The expansion of the envelope of single appointment dentistry is a reality of dental practice today and dentists have no alternative but to embrace computer-aided design and manufacturing to be able to offer it.
12.5 Digital Impression in Implant Dentistry

STL wireframes that form the basis of most 3D images
It is realistically possible today to consider the elimination of conventional impressions, at least in most dentate cases if not all cases. Intraoral scanners of today are also able to accurately image to a depth of 20 millimeters, allowing a large envelope for the size of restorations possible and their sub-gingival extent. Some advanced intraoral scanners are also capable of accounting for fluids such as blood and saliva and compensating for refractive error that may otherwise result.
More information on the types of scanners and their descriptions/uses is given in Chap. 11.

Integrated facial and intraoral scans
Integration of this nature allows substantial cross over between the digital and analog worlds. Clinicians and technicians are therefore able to choose workflows that they prefer and still manage continuity of flow from a digital workspace to an analog workspace and vice versa. Data sizes of intraoral scans have also become far more efficient with the evolution of newer file formats that allow greater mesh compression. This directly translates into ease of digital transfer and allows clinicians and technicians and other providers in various parts of the world to come together in one workspace.
The laboratory equivalent of intraoral scans is the model scanner that uses largely similar scanning principles. Model scanners typically scan impressions or poured-up dental models. In general, the direct scanning of impressions has always presented difficult challenges, with undercuts in an impression allowing limited access to a scanner. This is still best addressed by pouring up an analog model that can be scanned. It however continues to compel an analog step in a workflow that can otherwise be completely digital. The best digital solution offered for this problem continues to be the CBCT scanning of impressions and their inversion using 3D rendering software [46–48]. To some degree, the access of intraoral scanner heads does limit the amount of data that can be acquired by an intraoral scanner. It is expected that the size of intraoral scanners and their field of view will continue to see the improvement that will overcome these obstacles. The power consumption of intraoral scanners has also meant the development of models that can be battery supported, thus allowing greater portability.
This is a tremendous advantage in multioperatory dental offices and can serve to reduce a practice’s investment. The weight of intraoral scanners has also come down with time, despite the incorporation of heating of fans to reduce fogging. If anything, the pace of development continues to pose a challenge for rapid technological redundancy, and this can be an issue if the investment is not a well-thought-out decision. New generation intraoral scanners are also attempting to integrate other emerging technologies such as subtractive superimpositional techniques that allow for assessment of changes in a dentition such as wear, migration, or recession. Some scanners have also incorporated caries detection and transillumination modalities that allow for illuminative structural assessment of teeth.
12.5.1 Scan Bodies

Acuris conometric concept

Custom abutments from Atlantis
These custom abutments are often fabricated with a high degree of accuracy on either 5 axis lathes or computer numeric controlled lathes that are capable of generating custom abutments with a quality that is similar to prefabricated stock implant componentry. Some of these manufacturers even allow dentists to mill their restorations from core restoration files supplied by them(R). This ensures that costs are further curbed, and dentists are able to have well designed implant restorations that offer a quality fit on custom implant abutments.
12.5.2 Photogrammetry

Photogrammetry scan markers in an All on 4 (Courtesy of Dr Han Choi, Auckland)
12.6 Guided Implant Surgery

Planning image with color intraoral scan import
The earliest software packages were developed in Belgium and were tested extensively in multi-center clinical trials. At the time, most guided surgical stents were developed using stereolithographic techniques for rapid prototyping in centralized facilities. The cost of these stents was prohibitive, but they added significantly to the accuracy of implant placement. Companies also moved to develop surgical instrumentation that was specific to guided surgery and surgical protocols that incorporated these stents quickly became popular with strong marketing.
The development of intraoral scanning and new software for cone beam computed tomography saw integration taken to a whole new level. Dentists were able to plan implant restorations chair-side on digital models, although they were limited to single restorations and short edentulous spans. These planned implant restorations were easily incorporated into guided surgery software resulting in very accurate implant planning. Recent Software packages include libraries for multiple implant systems, thus broadening the scope for use of guided surgery. Software packages have now become increasingly intuitive as well, allowing users to slice data in a variety of custom planes that went beyond conventional multi-planar radiology [55–57]. This allowed users to place implants at any angle of their choice while still being able to assess distances, angles, and parallelism between multiple implants. Clinicians were also offered the ability to clearly delineate anatomical landmarks such as nerves in order to allow for accurate planning of implants in proximity to them. The ability to detect some of these landmarks has become increasingly more accurate with time as well, given high-resolution voxel sizes in current cone beam computed tomography machines. Some software today also allow volumetric planning of grafts in deficient sites [58–60]. It has also become possible to generate accurate three-dimensional models from radiographic data and integrate this with data from intraoral scans, facial scans, and soft tissue scans. The generation of new file formats is also opening up possibilities for incorporation of full color in these data sets, adding a whole new dimension to aesthetically driven digital treatment planning that involves implants.

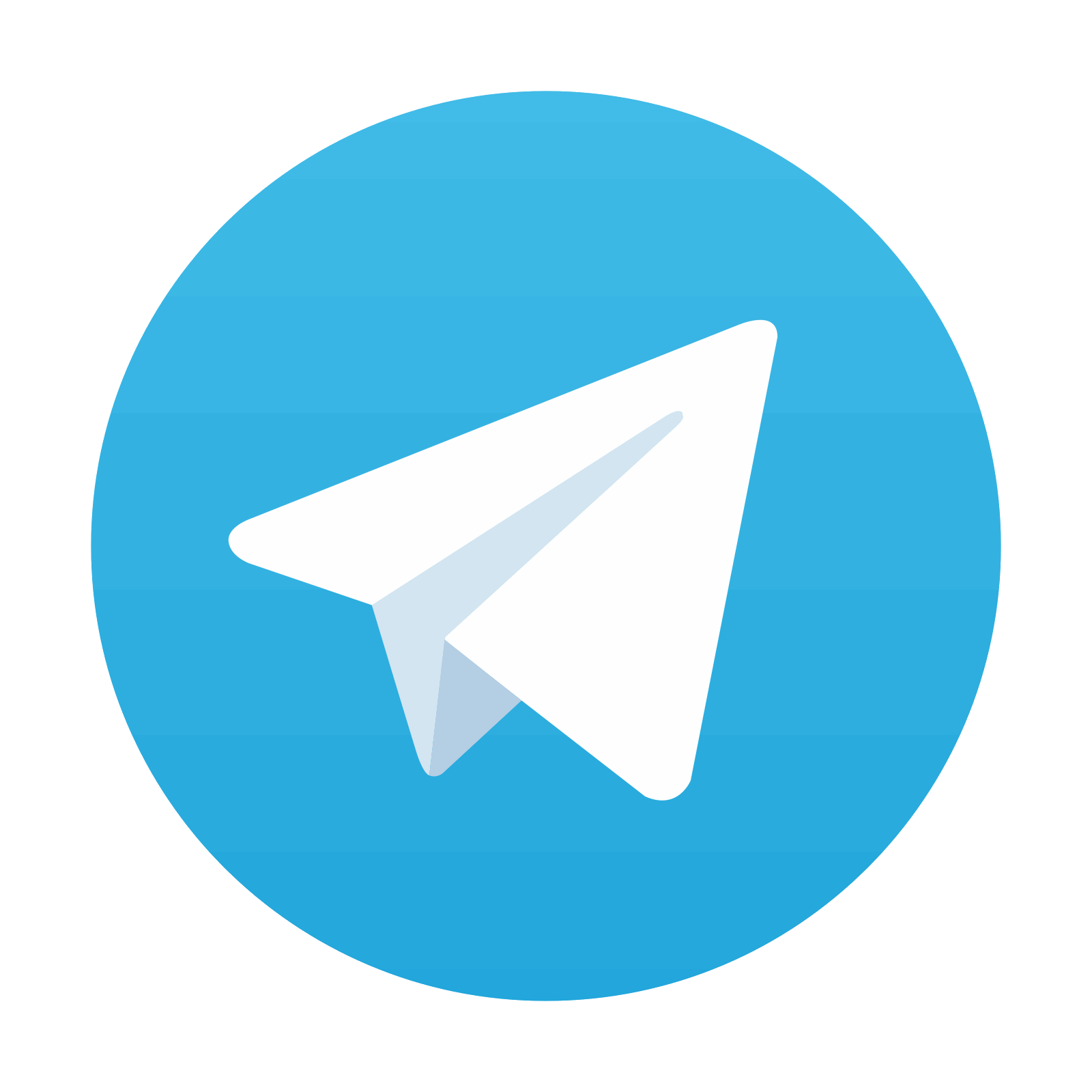
Stay updated, free dental videos. Join our Telegram channel

VIDEdental - Online dental courses
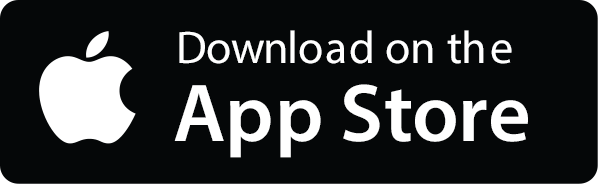
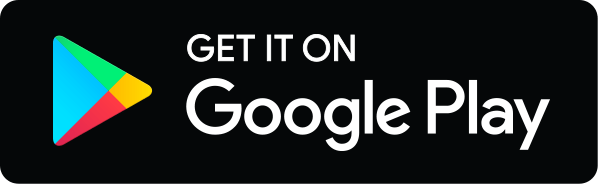