18
Implant Supportive Maintenance for Fixed Prosthetic Rehabilitations: The Patient with the Complete Arch Fixed Implant–supported Rehabilitation: Prosthetic Concepts to Optimize Maintenance Protocols
K. Michalakis1, S. Misci1, A. Abdallah1, D. Vasilaki2, and H. Hirayama1
1 Department of Restorative Sciences and Biomaterials, Boston University Henry M. Goldman School of Dental Medicine, Boston, MA, USA
2 Division of Prosthodontics, University of Connecticut School of Dental Medicine, Farmington, CT, USA
The introduction of dental implants as a treatment modality in dentistry has helped millions of edentulous people to overcome the need for removable complete dentures by making fixed implant–supported prosthetic reconstructions possible. Implants have thus offered a great service to edentulous patients with maladaptive denture experiences. While the success of dental implants has been reported to be outstanding, early and late implant failures, as well as failure of implant prostheses, are well‐documented and considered to be serious issues [1–3]. That is especially true for patients with advanced cases of bone resorption and limited bone quantity and quality for implant placement. Soft and hard tissue ridge deficiencies not only hinder the surgical and prosthetic success of implant therapy, but subsequent implant and/or prosthetic failure will cause further tissue loss, complicating treatment or leaving the patient with traditional removable prosthetics as the only remaining option. Maintenance is a critical component of dental care, and implant therapy is no exception. As such, it is the aim of the authors to present an evidence‐based discussion of factors influencing the short‐ and long‐term maintenance of fixed Implant–supported prosthetic rehabilitations.
The complications associated with complete arch fixed implant–supported rehabilitations are numerous as discussed in this chapter, but most of these complications can be summarized by the following etiologies: bacterial adhesion and load, host response, mechanical overload, and physical deterioration. In analyzing these etiologies, the authors have found that the choice of prosthetic materials is a key factor in prognosis affecting biocompatibility, occlusal surface, and color stability. Additional factors include the prosthesis design, especially at the intaglio surface, location of the prosthetic platform, and the mode of retention influencing the presence of residual cement.
Restorative treatment involving dental implants presents multiple interfaces, which impact the maintenance and prognosis of the restoration. Some of those interfaces exist within the prosthetic apparatus: implant to abutment, abutment to prosthesis framework, and prosthetic framework to veneering material, if any (Figures 18.1–18.2); some are between the prosthetic components and the surrounding hard and soft tissues: implant to bone, implant, and abutment to emergence soft tissue (non‐keratinized), intaglio surface of the prosthetic framework to the attached mucosa, polished surface of the prosthesis to the buccal and lingual mucosa, lips, and tongue, and occlusal surface to the opposing dentition or prosthesis (Figures 18.3–18.5). The topography of interfaces between the prosthetic components and the surrounding tissues, the properties of the materials, and the biology of those tissues in contact with the prosthesis would all influence proper hygiene, bacterial adhesion, and biological response. Passivity of fit, the use of screw or cement retention, the presence of micro‐gaps, as well as occlusal stability would additionally influence bacterial and mechanical factors, and thus, long‐term success.
The materials used in the fabrication of complete arch fixed implant prostheses include framework materials such as gold, silver‐palladium, titanium, zirconia, and high‐strength polymers, and veneering materials including acrylic resin, composite resin, and ceramics. These materials all have different physical and mechanical properties which influence the performance of the prosthesis and long‐term success. On the other hand, surface properties, such as roughness, hydrophobicity, and electric charge, can significantly modify the extent of bacterial adhesion [4]. Biological complications will then follow the increase in pathogenic bacterial load adjacent to a prosthesis. While oral bacteria can adhere to hydrophobic and hydrophilic surfaces, fluctuating shear conditions that exist in vivo prevent much biofilm from accumulating on hydrophobic surfaces. As such, biofilm tends to accumulate on hydrophilic surfaces, particularly those that are more rough than smooth. Multiple bacterial strains make up oral biofilms, but their character and impact can be material‐specific. For example, Candida species tend to attach to acrylic resin. Gold has been found to harbor thick biofilms in vivo, but they are barely viable. Biofilms on ceramics, on the other hand, are thin and highly viable. As for composites, biofilms cause surface deterioration, which bolsters the process of further biofilm formation and attachment [5].


Figures 18.1–18.2 Interfaces of prosthetic apparatus. (a) implant to abutment. (b) abutment to prosthesis. (c) framework to veneer.
The Metal‐acrylic Hybrid Prosthesis
Understanding the current advances in implant–supported prosthetic rehabilitation requires a historical understanding of the development of prosthetic materials used in this domain. This no doubt goes hand‐in‐hand with the earliest applications of implantology in dentistry. Immediately after comprehending the biologic process of osseointegration, Professor Brånemark and his co‐workers realized the therapeutic value of implants and foresaw the great impact implants would make in the dental community [6]. Therefore, based on their long‐time scientific research and observations on tissue repair and osseointegration of commercially pure titanium implants in jaw bones of dogs they started further experimental work aiming at the development of surgical and prosthetic protocols for the rehabilitation of mandibular completely edentulous patients [7]. Their primary aim was the prosthetic reconstruction of severely resorbed mandibular alveolar processes. Today, more than 50 years since the first placement of implants for the rehabilitation of an edentulous patient, it is known that complete arch implant–supported fixed dental prostheses have very high long‐term success rates [8–10]. The first prostheses were supported by five implants placed between the mental foramina and were fabricated by a metal framework with beads and other retentive elements, so as to retain the heat‐processed polymethyl methacrylate (PMMA) resin and ten to twelve denture teeth. As the implants were placed between the mental foramina, the posterior segments on each side were cantilevered. A biomechanical analysis was attempted at that time, without however taking into consideration differences in arch shapes, interarch distance, length of implants, and length of cantilevers (Figures 18.6–18.7) [6].



Figures 18.3–18.5 Interfaces of prosthetic components to tissues. (a) implant to bone (subcrestal). (b) abutment/prosthesis to soft tissue (subgingival), (c) prosthesis to soft tissue (intaglio/pontic), (d) prosthesis to buccal and lingual mucosa (polished), (e) occlusal surface to opposing dentition/prosthesis.
This type of prosthesis has been widely known as a hybrid prosthesis, as it has the characteristics of two types of prostheses. It is made of multiple materials used in a traditional removable complete denture, while at the same time, it is fixed on the osseointegrated implants through a metal framework and abutments, and therefore can only be removed by the dentist. According to the 9th edition of the Glossary of Prosthodontic Terms (GPT‐9), the term “hybrid prosthesis” is a nonspecific term applied to any prosthesis that does not follow conventional design; frequently used to describe a dental prosthesis that is composed of different materials, types of denture teeth (porcelain, plastic, composite resin), variable acrylic denture resins, differing metals or design, etc.; may refer to a fixed dental prosthesis, removable dental prosthesis, or maxillofacial prosthesis [11]. Most often, a hybrid prosthesis consists of an alloy framework with retentive elements (i.e. beads, rods, loops, etc.), supported by the implants. These retentive elements are necessary, because acrylic polymers cannot bond to metal, at least without surface treatment. This fact necessitates the incorporation of the retentive elements to mechanically interlock the processed acrylic. The denture teeth that are used in these restorations are chemically bonded with the PMMA resin retained on the alloy framework. This type of prosthesis is also known as the “Toronto bridge,” due to a series of clinical studies that Professor George Zarb and his colleagues conducted on that subject in the 1980s and 1990s, at the University of Toronto [12–14].


Figures 18.6–18.7 The metal framework of a mandibular hybrid prosthesis with beads and rods for the retention of PMMA resin. The hybrid prosthesis intraorally.
Removable overdentures supported by dental implants have also been utilized for the treatment of complete edentulism, as they are less expensive than their fixed counterparts, due to the smaller number of implants required for their support and the lower cost of the involved materials and laboratory procedures needed for their fabrication [15–17]. Additionally, they are preferred when patients have limited bone volume at the host sites, and they are unwilling to proceed with bone grafting procedures, due to financial or other restrictions. Moreover, a consensus conference in the early 2000s has advocated that implant–supported overdentures should be considered as the first prosthetic treatment of choice in case of complete edentulism in the mandible and should always be presented to edentulous patients as a better alternative, given that there are no contraindications for implant placement [18] (Figures 18.8–18.13).


Figures 18.8–18.9 Loss of facial support due to extensive residual alveolar ridge resorption in an elder patient.


Figures 18.10–18.11 Two implants placed in the mandibular canine regions and an implant–supported complete denture fabricated to restore function and facial support.
It should also be mentioned that the selection of a removable complete denture as a treatment option, is many times necessitated because of the substantial loss of volume of the residual alveolar ridges and loss of lip support, which can be better served by removable prostheses. Finally, this type of prosthesis is many times indicated in cases of geriatric patients and/or patients with diminished manual dexterity, due to Parkinson’s disease, stroke, arthritis, peripheral neuropathy, etc., which prevent the application of proper oral hygiene measures [19]. These measures, in the cases of hybrid prostheses, are quite demanding, involving the use of interproximal brushes, floss threaders, and oral irrigator units.
Prognosis and Maintenance of Metal‐acrylic Hybrid Prostheses
In the initial era of implant prosthodontic therapy, the use of PMMA acrylic resin in combination with denture teeth was considered as an advantage, due to the absorption of occlusal forces, which would not be transmitted to the supporting implants. To compensate for the stark difference in expected biomechanical behavior between the periodontal ligament compared to implants, Brånemark recommended that implants and natural teeth not be used as abutments for the same fixed prosthesis, that implants be restored with resilient restorative materials, and that prostheses be planned with a retrievable design [20]. However, acrylic denture teeth wear down much faster than enamel and porcelain (Figures 18.14–18.17). As a result, long‐term occlusal stability is very often compromised, with deleterious effects in the function of the stomatognathic system and the prognosis of the restoration. A clinical study by Ohlmann et al. found that after a period of six months, the mean wear of acrylic teeth was 20.5 μm. The wear was significantly higher in males than in females. Moreover, this study has shown that the wear was higher when the acrylic teeth had natural teeth as antagonists [21]. A very recent systematic review has concluded that even new‐generation denture acrylic teeth wear substantially. Factors that play a role in this wear include the antagonists, the age and the sex of the patient, the type of the prosthesis, and the amount of the occlusal superficial layer removed during equilibration, at the delivery appointment [22]. Several studies in the past have also demonstrated similar results [23–25]. These studies have also shown that it is always better to use the same restorative materials in opposing arches.


Figures 18.12–18.13 Maxillary complete denture and implant–supported mandibular overdenture in place. Restored lip support and vertical dimension of occlusion.


Figures 18.14–18.15 Extensive wear of acrylic denture teeth after many years of service.
The cumulative implant survival rates for metal‐acrylic hybrid prostheses range between 87.9 and 100% for follow‐up periods of 5–10 years and 78.3–98.9% for 10 years or more. Cumulative prosthetic survival rates are 93.3–100% and 82–100%, for periods of 5–10 years and 10 years or more, respectively [26]. Bone loss around implants supporting hybrid prostheses has been reported to be greater than 2 mm in 20.1% of the cases after a 5‐year period, and in 40.3% of the cases after 10 years [27]. Prosthetic complications in these prostheses usually include loosening or fracture of fastening screws, fracture of the PMMA denture acrylic resin, as well as fracture or debonding of acrylic resin teeth (Figures 18.18–18.19) [26]. A systematic review has reported that screw loosening or fracture occurs at rates of 10.4 and 20.8% after periods of 5 and 10 years, while chipping or fracture of veneering materials occurs at a rate of 30.3% after 5 years and 66.6% after 10 years [27]. Framework fractures have also been reported, but at a rate of 8.8% after a period of 15 years [28]. The highest incidence of prosthetic incidences was reported in a retrospective study by Krennmair et al., where 256 complications were recorded in 42 individuals, after five years of service [29]. As already mentioned, phonetic and esthetic issues have also been reported by patients treated with hybrid prostheses [30]. Esthetic deficiencies due to progressive deterioration of the resin material of the prosthetic base and of the denture teeth were reported at a 9% rate [28].


Figures 18.16–18.17 Hybrid metal‐acrylic bar‐retained removable implant prosthesis with gold occlusal surfaces to provide stable occlusal support and circumvent high wear and fracture rate of acrylic resin.
Bacterial Adhesion to Metal‐acrylic Hybrid Prostheses
Apart from the fact that denture teeth wear down, classic hybrid prostheses are associated with inherent problems of PMMA denture acrylics, namely weak strength, residual monomer content, low dimensional stability, water absorption, as well as color changes. Internal and external porosity in heat‐processed acrylic resins is one additional disadvantage. Porosity usually results from the presence of residual monomer, which may boil if the peak temperature of the resin rises, due to the exothermic reaction of the polymerization, above the boiling point of monomer [31]. Then, the evaporation of the unreacted monomer may produce porosity, which can compromise esthetic properties and physical characteristics, adversely affecting hygiene. Retention of fluids and harboring of micro‐organisms in the porous surface of PMMA acrylics may negatively impact the health of the peri‐implant tissues [32]. Recent studies have demonstrated that acrylic resins are prone to microbial colonization, with anaerobe species prevailing over aerobes. This research has shown that the most predominant bacteria in acrylic resins were actinomyces, oral streptococci, and Enterobacteriaceae [33, 34]. Bacteria present possible threats to treatment success, as they can lead to peri‐implant mucositis and peri‐implantitis. Peri‐implant mucositis has been found to be present in 80% of the cases, while peri‐implantitis was reported to be present in 56% of the cases [35]. Previous research has shown that surface irregularities, voids, or pits can be colonized as fast as 30 minutes from the moment a prosthesis is placed in the oral cavity [36, 37]. Surface roughness significantly increases the available surface area for the attachment of bacteria and offers support for adhesion [38].


Figures 18.18–18.19 Fractured and debonded acrylic resin denture teeth of metal acrylic hybrid fixed implant prosthesis with limited prosthetic space, replaced with new denture teeth.
Bacterial adhesion starts at sites protected from shear forces and is driven by hydrophobic, electrostatic, and van der Waals forces, which can take bacteria in a proximity with the acquired pellicle on implant surfaces [39, 40]. Bacteria’s direct adhesion to the proteins of the acquired pellicle leads to the establishment of an irreversible attachment. This is a critical point as next, upregulation of bacterial activity and migration on implant surfaces is noticed. From that moment on, a maturation of dental plaque and an increase in the diversity of the bacterial community is observed [41–43]. In the initial stages of biofilm formation, gram‐positive cocci, non‐motile bacilli, as well as a limited number of gram‐negative anaerobic species dominate supra‐ and sub‐gingival plaque [44, 45]. Different bacteria have been identified by numerous studies in the dental literature. It has also been observed that bacterial species have some dynamic specific interrelationships which may produce shift patterns in the colonization process [46]. That explains the fact that a discrepancy both in the quality and quantity of peri‐implant microbiota is very often noticed between screened populations [47].
Besides material’s surface roughness, other surface properties may contribute to a stronger or weaker adhesion too. These primarily include: (i) surface topography, (ii) surface charge density, (iii) surface wettability. Other environmental factors, such as the fluid dynamics and bacterial mobility also affect bacterial adhesion on dental material surfaces [48]. (i) Surface topography is a term that is related to surface roughness, as it refers both to surface roughness and the profile shape. Bacteria can sense mechanical cues related to surfaces. Generally, surface microscale topography, in the size order of bacteria can impact their adhesion via hydrodynamics. Surface topography characteristics in the nanoscale affect bacterial attachment through deformation of cell membrane, physicochemical forces, and chemical gradients [49]. Furthermore, it has been demonstrated that the expression of bacterial adhesions can be modified when the surface topography is altered [50, 51]. Research has shown that bacterial attachment is enhanced when material surface has characteristic features larger than a single bacterium, as the latter can find shelter and greater available contact area, [52, 53] (ii) surface charge density of the dental material affects bacterial adhesion, as electrostatic interactions and van der Waals forces are contributing greatly in this process [54]. According to published data, bacterial adhesion is easier on surfaces that are positively charged, as usually bacteria have a negative charge on their cell wall surfaces, due to the presence of amino, carboxyl, and phosphate groups [55–59]. Specifically, for PMMA acrylics which are positively charged, it was shown that adhesion and growth of Pseudomonas aeruginosa was twice as much, compared to negatively charged surfaces [60]. Similar results regarding Pseudomonas, Staphylococcus aureus, and Escherichia coli have been reported by other researchers too [61]. It should be mentioned, however, that there is evidence showing that some bacteria have the ability to adhere to negatively charged surfaces through the help of lipopolysaccharides (LPS) or appendages, like fimbriae, existing on their surfaces [62, 63], (iii) surface wettability is defined by how much a liquid phase is spread over a solid surface. Therefore, an increased contact area between a liquid and a solid shows enhanced surface wettability, and it is one of the major properties determining solid–liquid interactions in biological systems [48]. Material’s surface energy and roughness affect wettability, and several models have been developed to demonstrate the association between bacterial adhesion and surface energy. For that purpose, a thermodynamic approach has shown that bacteria with hydrophilic cell surfaces tend to favor hydrophilic materials, while bacteria with hydrophobic cell surfaces show a preference to hydrophobic materials [64]. As mentioned previously, van der Waals and Coulomb interactions affect bacterial adhesion to material surfaces. In that sense, the distance between bacteria and surfaces, as well as the ionic strength of the surrounding fluid is very important. Generally, as ionic strength increases, so does the bacterial adhesion to materials [65]. The fact that PMMA denture acrylic presents increased porosity and favors bacterial adhesion combined with the difficult access for hygiene under hybrid prostheses has led to the use of highly polished metal at the gingival surface (Figures 18.20–18.21).
Dental alloys can be highly polished and therefore present minimal surface roughness, which favors bacterial adhesion. Research has shown that resin and synthetic materials are more prone to bacterial adhesion than metals, especially chrome–cobalt [4]. Therefore, if a hybrid prosthesis is chosen as a treatment modality, a proper convex design with metal finishing should be selected. Moreover, space between the prosthesis and the residual alveolar ridge should be provided to facilitate oral hygiene measures. This space, which is required for dental hygiene, may pose a problem if used in the maxilla, as it is many times associated with speaking problems, due to the air passage between the residual alveolar ridge and the prosthesis. Some esthetic concerns have also been reported in the literature, due to the aforementioned space [30].


Figures 18.20–18.21 Implant supported hybrid prosthesis with denture teeth and PMMA acrylic resin at the coronal surface and highly polished metal at the gingival surface.
Although highly polished metal presents smaller bacterial adhesion than acrylic resin, dental ceramics demonstrate an even better behavior than dental alloys. An in vitro study has demonstrated that artificial plaque synthesized of Streptococcus sobrinus and glucans was retained significantly less on dental ceramics than on metals and resins [66].
The Shift to Metal–Ceramics
With the advent of new methods and materials in the early 1990’s, full‐arch implant–supported prostheses shifted from denture acrylic PMMA to metal–ceramics in an effort to avoid the shortcomings of acrylic resin.
Although there is a lot of published evidence on the clinical outcome of hybrid prostheses and their supporting implants, there is not enough data on full arch metal–ceramic implant‐retained restorations. However, as metal–acrylic restorations are currently rarely in use, it is very important for clinicians to know the technical and biologic complications of metal–ceramic implant–supported fixed complete dentures. This information is critical for treatment planning and design of the prostheses. There is a need for clinical studies with large sample sizes and long follow‐up times, in order to draw some meaningful conclusions. A recent retrospective study that included 52 patients with both metal‐acrylic and metal‐ceramic full‐arch fixed complete dentures found that while the 5‐year and the 10‐year failure rate for the metal‐acrylic was 7.1 and 58.2% respectively, the corresponding failure rates for metal‐ceramic full‐arch prostheses were 0% [67].
Technical complications occur quite often in full‐arch fixed implant–supported prostheses. These can be divided into two broad categories: minor and major complications. Minor complications include all these complications which are easily managed and can be usually fixed chairside. These include minor wear or chipping of the veneering material, loss of the screw access filling, and abutment‐ or prosthetic‐screw loosening. On the contrary, major complications include those complications that are quite serious and cannot be easily managed chairside. These include fracture of a big part of the veneering material, fracture of the framework, fracture of the abutment, fracture of a fastening screw, and fracture of an implant (Figures 18.22–18.26).
It should be indicated that the same retrospective study has found that more than 40% of the full‐arch fixed implant–supported prostheses (for both the hybrid and the metal‐ceramic) presented a complication in the first 5 years, while the estimated complication rates for the 10‐year period was 98% [67]. Although a bigger number of complications was identified in that study in the metal–ceramic group (78.6%) than in the metal–resin (hybrid) group (42.3%), it should be mentioned that more metal‐ceramic (55) than metal–resin (16) prostheses were included.
Modern CAD/CAM Framework Materials
By the start of the twenty‐first century, framework materials had been traditionally metal‐based. This was to provide implant–supported fixed dental prostheses with sufficient rigidity [68]. Gold alloy was considered the benchmark material for frameworks of implant–supported fixed dental prostheses. Due to the high cost associated with gold, a demand for less expensive options developed. This included titanium, which presented with a technically difficulty manufacturing process and higher framework dimension requirements, which subsequently affected the level of patient comfort [68]. The arrival of computer‐aided design/computer‐aided manufacturing (CAD/CAM) provided an alternative manufacturing process that bypassed the shortcomings of the traditional process (waxing/investing/casting) and the associated procedural errors. With CAD/CAM technology, large frameworks could now be manufactured precisely from homogeneous blanks of various high‐strength materials [68]. This included zirconia, which has now been in use in dentistry for about two decades, dominating the market owing to its high strength, low cost, and natural white color [69].


Figures 18.22–18.23 (Top) Multiple fractures of the veneering material in a non‐esthetic area. This can be polished with fine‐grit diamonds and abrasive points. (Bottom) Fracture of the framework is one of the most serious technical complications. Although the occlusogingival and buccolingual size of the connector is appropriate (>3 mm), the fact that the soldering metal did not reach the central part of the connector caused the fracture.


Figures 18.24–18.25 Fracture of an abutment screw. In some cases, these screws can be retrieved by a counterclockwise motion with an explorer. If this is not possible, an ultrasonic scaler can be employed, taking care not to approach the internal threads of the implant, as they can be damaged. Some retrieval systems are also available from implant companies.
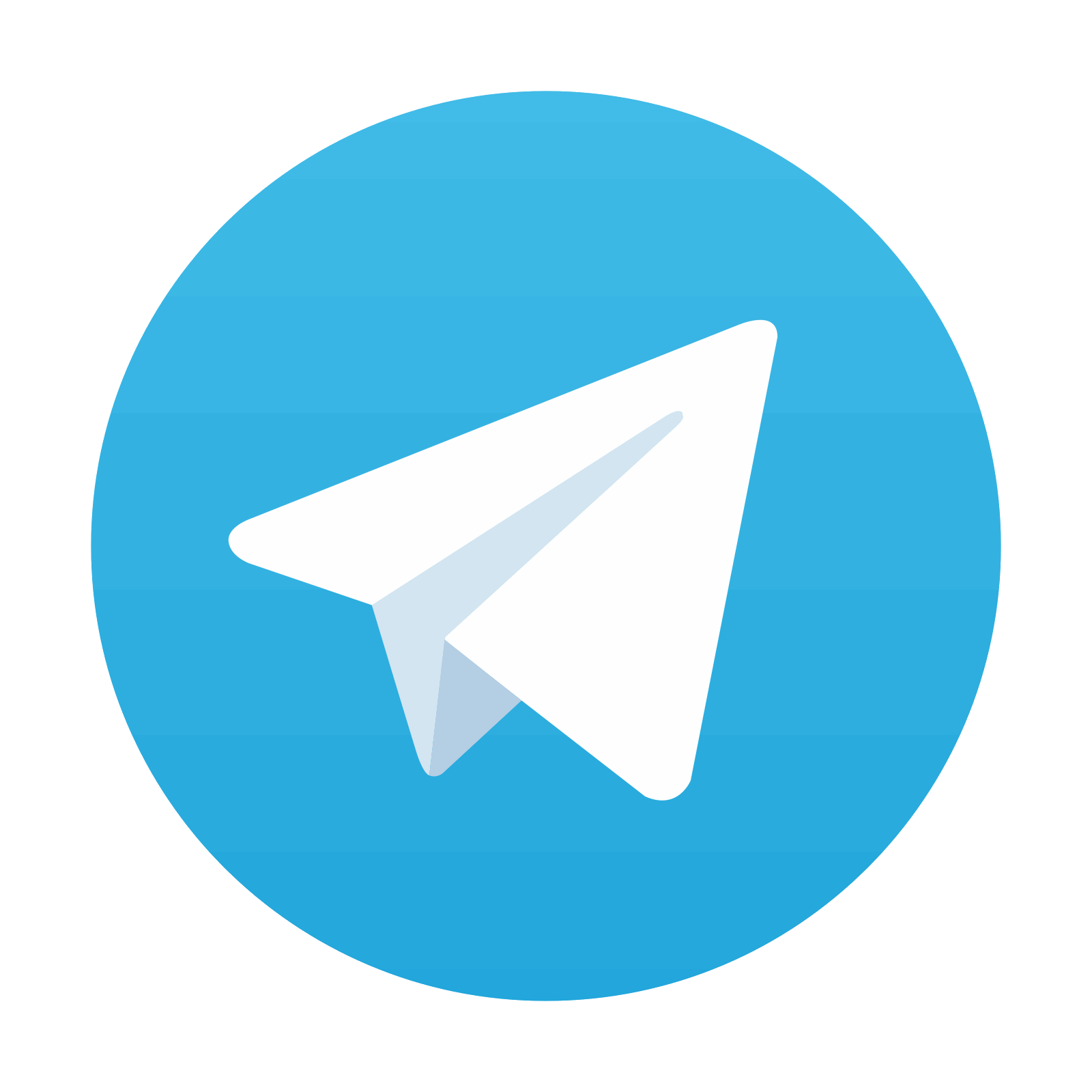
Stay updated, free dental videos. Join our Telegram channel

VIDEdental - Online dental courses
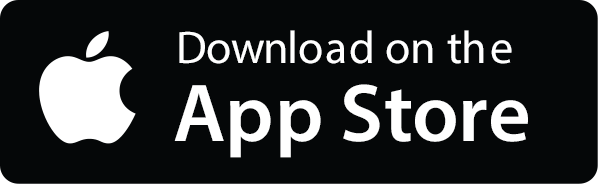
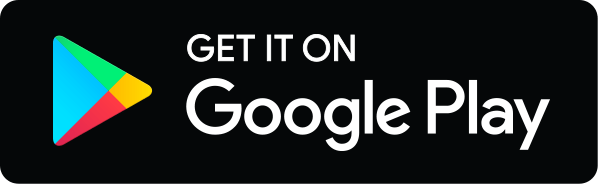