Abstract
Objectives
10-Methacryloyloxy-decyl-dihydrogen-phosphate (10-MDP) is currently considered as one of the most effective functional monomers for dental bonding, this in part thanks to its long and relatively hydrophobic spacer chain, adequately separating the polymerizable from the phosphate functionalities. This study compared functional monomers with different spacer chains’ length and hydrophilicity to 10-MDP on their dentin and enamel bonding performance.
Methods
Atomic absorption spectroscopy (AAS) was used to characterize the chemical interaction. Micro-tensile bond strength (μTBS) and fractographic analyses were performed after 24 h and one year. Confocal micro-permeability and SEM nanoleakage assessments were also undertaken. The tested functional monomers were 2-MEP (2-carbon spacer), 10-MDP (10-carbon), 12-MDDP (12-carbon), MTEP (high hydrophilic polyether spacer chain) and CAP-P (intermediate hydrophilic ester spacer).
Results
AAS revealed clear differences ( p < 0.05) in monomer-calcium salt formation in this order: 12-MDDP = 10-MDP > CAP-P > MTEP > 2-MEP. The highest initial dentin μTBS was obtained using 10-MDP or 12-MDDP. After 1-year aging, a significant drop ( p < 0.05) in μTBS was observed for the adhesives with MTEP (enamel and dentin), 2-MEP (enamel) and CAP-P (enamel). MTEP presented the highest micro-permeability, while 2-MEP, CAP-P and MTEP showed increased nanoleakage after aging.
Significance
These outcomes showed that more hydrophilic and shorter spacer chains may compromise the chemical interaction with calcium and the dentin/enamel bonding performance.
1
Introduction
The longevity of composite restorations using contemporary dental bonding agents (DBAs) can be compromised over time due to hydrolytic degradation . Following the etch-and-rinse approach, incomplete monomer infiltration in 30–40% H 3 PO 4 -etched dentin may affect the bonding performance of DBAs . Dentin/enamel demineralization (200 nm to 2 μm) along with simultaneous infiltration of monomers is feasible following the self-etch approach using acidic functional monomers . Their twofold bonding mechanism relies on micromechanical interlocking (after in situ polymerization) and ionic interaction .
The chemistry behind the formulation of functional monomers represents the key factor influencing the initial and long-term bonding effectiveness of self-etch adhesives . The functional monomer 10-methacryloyloxy-decyl-dihydrogen-phosphate (10-MDP) is currently one of the most effective functional monomers in terms of chemical interaction and clinical longevity . This is generally explained by its long and relatively hydrophobic spacer, adequately separating the polymerizable methacrylate from the phosphate functional group . Nonetheless, very little information is present in literature regarding the length and hydrophilicity of the spacer chain on the self-etch bonding performance.
Therefore, the goal of this investigation was to characterize the chemical interaction and determine the dentin/enamel bonding effectiveness of experimental adhesives based on 10-MDP or four other functional monomers containing spacers with different length and polarities, this using atomic absorption spectroscopy (AAS), micro-tensile bond strength (μTBS), micro-permeability and nanoleakage assessments. The two null hypotheses to be tested were that the spacer chains with different length (number of carbons) and hydrophilicity (presence of ether or ester groups) (1) did not influence the chemical interaction of the tested functional monomers, and (2) did not influence the initial and long-term enamel/dentin bonding effectiveness and their respective interface integrity.
2
Materials and methods
2.1
Syntheses of functional monomers
The functional monomers were synthesized following the protocol of Ogliari et al. . Briefly, 1,10-decanediol [HO(CH 2 ) 10 OH], 1,12-dodecanediol [HO(CH 2 ) 12 OH] and tetra-ethylene-glycol [HO(CH 2 CH 2 O) 3 CH 2 CH 2 OH] (all from Sigma Aldrich, St. Louis, USA) were esterified using methacrylic acid (Sigma) to attach the polymerizable methacrylate functionality. Caprolactone 2-methacryloyloxy-ethyl ester [HO(CH 2 ) 5 CO 2 CH 2 CH 2 O 2 CC(CH 3 ) CH 2 ] was used after that as it is available as the methacrylate-functionalized intermediate. The synthesis of 10-methacryloyloxy-decyl-dihydrogen phosphate (10-MDP), 12-methacryloyloxy-dodecyl-dihydrogen phosphate (12-MDDP), methacryloyloxy-tetraethylene-glycol-dihydrogen phosphate (MTEP) and methacryloyloxy-caprolactone-dihydrogen phosphate (CAP-P) was accomplished by reaction with phosphorus pentoxide in methylene chloride. After purification , the isolated products (high purity: >95%, as determined by chromatography) were characterized as described previously to confirm the synthesis of 10-MDP, 12-MDDP, MTEP and CAP-P . The monomer 2-methacryloyloxy-ethyl-dihydrogen phosphate (2-MEP) was donated by Esstech (Essington, PA, USA). The chemical structures of monomers studied are shown in Fig. 1 a .
The hydrophilicity of each acidic functional monomer was assessed by a Chemical Structure Drawing software (Chem Draw Ultra version 6.0.2, Perkin Elmer, Waltham, USA) which calculated the estimated hydrophobicity of each structure by means of Viswanadhan’s fragmentation method . Higher logarithms of partition coefficient indicate more hydrophobic structures.
2.2
Atomic absorption spectroscopy
Pure monomers were mixed with water/ethanol (50/50 vol%) in 0.1 mM. Free calcium (Ca 2+ ) was prepared by dissolution of CaCl 2 in deionized water. The monomers were mixed with calcium as previously described except for the initial Ca 2+ concentration which was set to 3 ppm, enabling synthesis of the monomer-calcium salts. The resultant solutions were analyzed using atomic absorption spectroscopy (AAS) with the spectrophotometer AA240FS (Varian, Palo Alto, USA) to detect the absorbance related to the presence of free-calcium. The observed absorbance of Ca 2+ was inversely proportional to the formation of monomer-calcium salts. Five solutions were prepared for each monomer ( n = 5); the data were analyzed with one-way ANOVA and Tukey’s test ( α = 0.05).
2.3
Preparation of experimental adhesives
A resin blend was prepared by mixing 30 wt% urethane-dimethacrylate, 10 wt% bisphenol-A-diglycidyl-dimethacrylate, 7 wt% triethylene-glycol-dimethacrylate, 5 wt% hydroxyethyl-methacrylate, 15 wt% deionized water, 30 wt% ethanol, 0.5 wt% camphoroquinone, 1 wt% ethyl 4-dimethylaminebenzoate and 1.5 wt% diphenyliodonium hexafluorophosphate. The acidic functional monomers (15 mol%) were next added to the resin blend to create a series of experimental one-step self-etch adhesives (1-SEAs).
2.4
Micro-tensile bond strength
Extracted human third molars were used after approval of the Institutional Review Board (protocol 127/2011) from Piracicaba Dental School and stored in 0.5% chloramine/water solution at 4 °C for no longer than two months.
The occlusal part of the crowns was removed to expose flat mid-coronal dentin surfaces using a slow-speed water-cooled diamond saw (Isomet-1000, Buehler, Lake Bluff, USA), followed by a second parallel cut at the cemento-enamel junction to remove the roots. For the enamel specimens, the roots were removed as described and the crowns were cut in halves mesio-distally. The buccal and lingual enamel were ground flat using 600-grit SiC paper to produce flat enamel surfaces. The dentin and enamel surfaces were then wet-abraded (60 s) using 600-grit SiC papers to produce standardized smear-layers and immediately bonded using the experimental 1-SEAs. They were actively applied for 30 s, gently air-dried and light-cured for 20 s using the halogen light-curing unit XL-2500 (600 mW/cm 2 ; 3M-ESPE, St. Paul, MN, USA). The composite Filtek Z250 (3M-ESPE) was used for build-ups.
All bonded teeth ( n = 10/group) were immersed in distilled water for 24 h at 37 °C and subsequently sectioned into 0.9 ± 0.02 mm 2 sticks using the Isomet saw. Half of the sticks from each tooth were tested immediately, whilst the remaining half was stored for one year in distilled water that was monthly refreshed. The sticks were fixed to jigs using cyanoacrylate glue and tested to failure under tension in a universal testing machine EZ-test (Shimadzu, Kyoto, Japan) with a crosshead speed of 1.0 mm/min. Bond strengths of sticks from the same tooth were averaged and the mean was used as statistical unit. Sticks that failed prematurely were included as 0 MPa. The data were statistically analyzed for enamel and dentin separately using two-way ANOVA (functional monomer and storage period) and Tukey’s test ( p < 0.05).
2.5
Analysis of fracture mode
The fractured sticks were imaged to determine the failure mode by stereomicroscopy at 60× magnification. Representative de-bonded sticks were processed for scanning electron microscopy (SEM). The parts of the fractured sticks were paired and mounted on aluminum stubs. Thereafter, they were dehydrated in silica gel, gold-sputter coated and examined using SEM, JSM-5600LV (JEOL, Tokyo, Japan). The fractures were classified as ‘adhesive’, ‘cohesive’ or ‘mixed’.
2.6
Micro-permeability assessment
Three teeth per experimental group ( n = 3) were bonded as previously described with the 1-SEAs doped with 0.1 wt% rhodamine-B (Sigma) and assessed by confocal laser scanning microscopy (CLSM) . Briefly, the micro-permeability of resin–dentin interfaces was evaluated using a 0.3 wt% aqueous fluorescein (Sigma) solution. This dye was perfused for 3 h under 20 cm H 2 O simulated pulpal pressure to trace the water-filled spaces and test the sealing ability of the experimental 1-SEAs. The specimens were subsequently cut into 1-mm thick slabs, slightly polished with 2000-grit polishing paper and ultra-sonicated for 5 min.
The specimens were next examined using CLSM (Leica SP2 CLSM, Heidelberg, Germany) equipped with a 63×/1.4 NA oil immersion lens using 488-nm and 568-nm laser illumination. CLSM reflection and fluorescence images were obtained with a 1-μm z-step to section optically the specimens up to 20 μm below the surface. The z-stack scans were compiled into single projections. Each resin–dentin interface was completely characterized and images were randomly captured representing the micro-permeability observed along the bonded interfaces.
2.7
Nano-leakage evaluation
Ten dentin-bonded sticks from each group were analyzed for nano-leakage, as previously described , using a 50 wt% ammoniacal silver nitrate solution [Ag(NH 3 ) 2 NO 3 ]. The specimens were immersed in the tracer solution for 24 h and then immersed in photo-developing solution for 8 h under a fluorescent light to reduce silver ions into metallic silver grains. Thereafter, the specimens were rinsed with distilled water, embedded in epoxy resin stubs and polished using successively 600-, 1200- and 2000-grit wet SiC papers, and 3, 1 and 0.25 μm diamond pastes (Buehler); they were ultrasonically cleaned (5 min) after each abrasive/polishing step. The specimens were dehydrated in silica gel, coated with carbon and examined using SEM in backscattered electron mode.
2
Materials and methods
2.1
Syntheses of functional monomers
The functional monomers were synthesized following the protocol of Ogliari et al. . Briefly, 1,10-decanediol [HO(CH 2 ) 10 OH], 1,12-dodecanediol [HO(CH 2 ) 12 OH] and tetra-ethylene-glycol [HO(CH 2 CH 2 O) 3 CH 2 CH 2 OH] (all from Sigma Aldrich, St. Louis, USA) were esterified using methacrylic acid (Sigma) to attach the polymerizable methacrylate functionality. Caprolactone 2-methacryloyloxy-ethyl ester [HO(CH 2 ) 5 CO 2 CH 2 CH 2 O 2 CC(CH 3 ) CH 2 ] was used after that as it is available as the methacrylate-functionalized intermediate. The synthesis of 10-methacryloyloxy-decyl-dihydrogen phosphate (10-MDP), 12-methacryloyloxy-dodecyl-dihydrogen phosphate (12-MDDP), methacryloyloxy-tetraethylene-glycol-dihydrogen phosphate (MTEP) and methacryloyloxy-caprolactone-dihydrogen phosphate (CAP-P) was accomplished by reaction with phosphorus pentoxide in methylene chloride. After purification , the isolated products (high purity: >95%, as determined by chromatography) were characterized as described previously to confirm the synthesis of 10-MDP, 12-MDDP, MTEP and CAP-P . The monomer 2-methacryloyloxy-ethyl-dihydrogen phosphate (2-MEP) was donated by Esstech (Essington, PA, USA). The chemical structures of monomers studied are shown in Fig. 1 a .
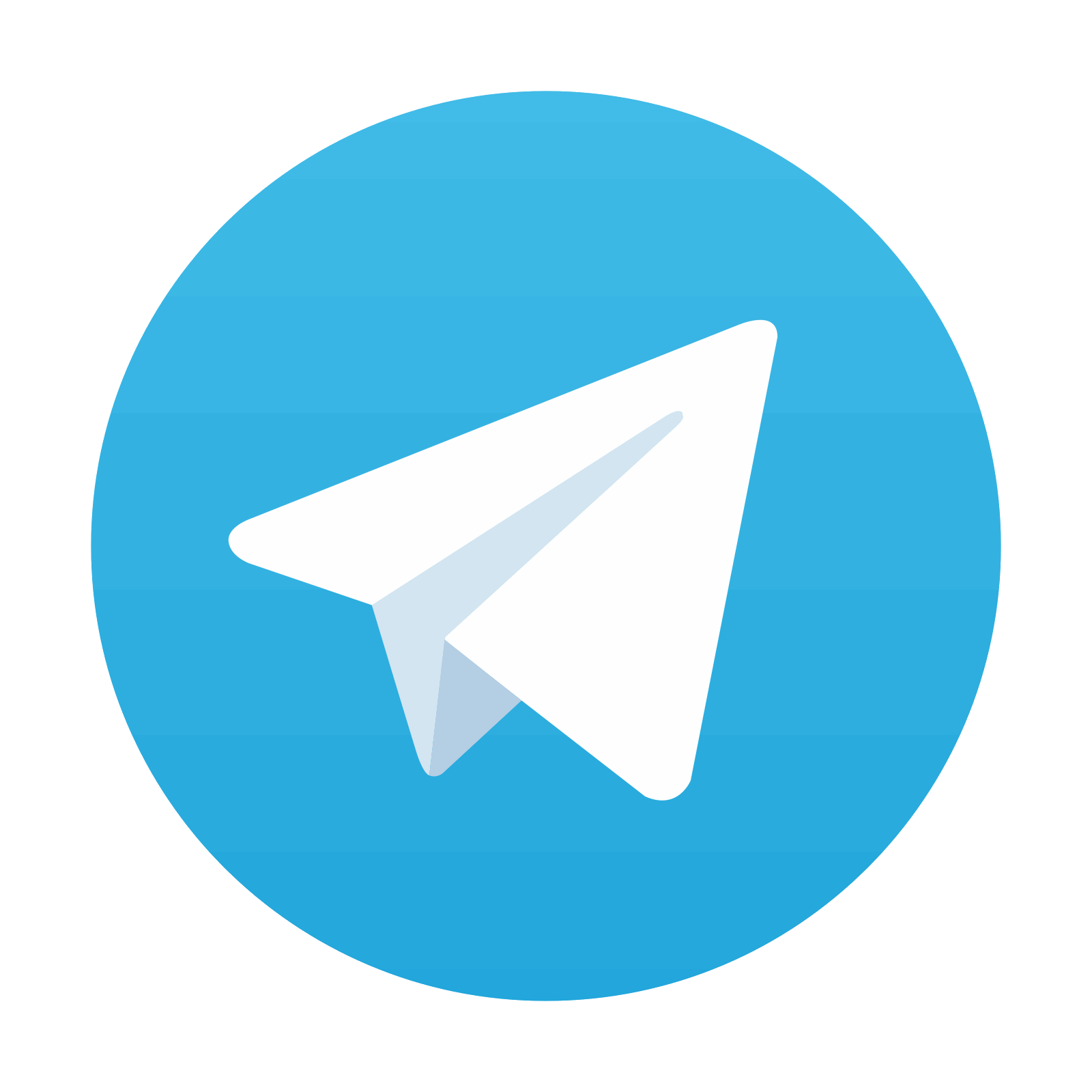
Stay updated, free dental videos. Join our Telegram channel

VIDEdental - Online dental courses
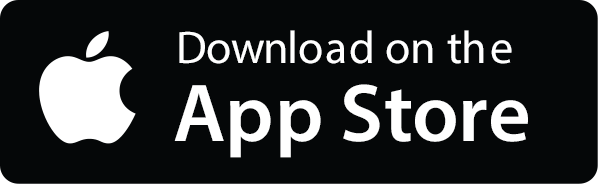
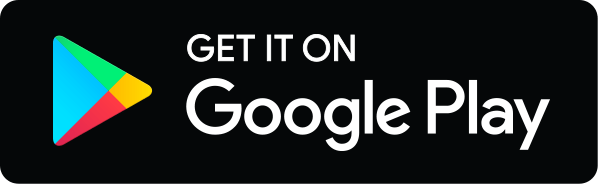
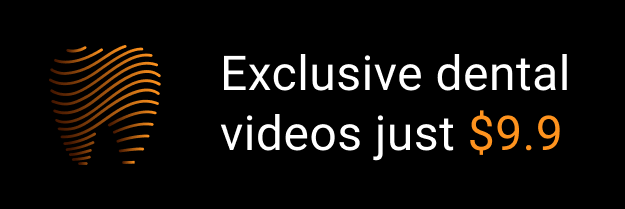