Abstract
The immunohistochemical characteristics of wound healing following application of a biphasic calcium phosphate or a collagen coated natural bone combined with a native collagen membrane in a dog model was assessed. Standardized buccal dehiscence-type defects were surgically created following implant bed preparation in 6 dogs. Following implant placement, defects were randomly filled with a collagen coated natural bone mineral (GBO), or a biphasic hydroxyapatite/beta tricalcium phosphate (SBC), and covered with a native collagen membrane. After 1, 4, and 9 weeks’ submerged healing, dissected blocks were processed for immunohistochemical (collagen type I (CI), osteocalcin (OC), angiogenesis (TG)) analysis. At 1 week, GBO and SBC granules were homogeneously surrounded by a well vascularized, non-mineralized tissue (NMT). CI and OC antigen reactivity was commonly observed adjacent to both bone graft substitutes. At 4 and 9 weeks, SBC and GBO granules were completely integrated into a secondly formed network of spongiosa. At 9 weeks, dissolution of some granules was observed in the SBC group. Adjacent to these granules, NMT was significantly increased and revealed a pronounced CI, OC and TG antigen reactivity. The initial pattern of bone regeneration and graft integration was comparable in both groups; bone remodelling was more pronounced with SBC.
Xenogenic derived type I and III collagen barrier membranes have been proven to predictably support guided bone regeneration (GBR) at dehisced implant sites . Some of the potentially advantageous properties of collagen over synthetic materials are related to: its hemostatic function, allowing early wound stabilization; chemotactic properties to attract fibroblasts; and semipermeability, facilitating nutritient transfer . A major drawback, particularly of native collagen, is fast biodegradation, resulting in a poor ability to maintain space thus compromising the secluded wound area . Accordingly, native collagen is commonly used in combination with either bone grafts or bone graft substitutes to support the membrane preserving its original position . Owing to its osteogenic, osteoinductive and osteoconductive properties , particulate autograft must still be considered to be a gold standard grafting material for the rehabilitation of dental implant-related defects . Some potential drawbacks are related to its quantity at specific donor sites, an increased morbidity and patient discomfort, as well as its potential resorption . In order to overcome some of these problems, various bone graft substitutes, ranging from alloplastic to coral, algae, or xenogenic derived materials have been introduced . The ideal bone graft substitute requires at least some physicochemical properties such as biocompatibility, osteoconductivity, resorbability, and steadiness. The most common source of xenogenous hydroxyapatite (HA) is bovine bone, which has also been studied extensively for bone augmentation in combination with the GBR technique . These grafting materials are also referred to as natural bone minerals, since proprietary processes are suggested to remove all cells and proteinaceous material, leaving behind an inert bone scaffold exhibiting a specific three-dimensional surface structure . Recently, a natural bone mineral was coated with porcine derived collagen to render its surface even more attractive for cell adhesion (GBO, BioOss Collagen ® ) . Amongst the alloplastic materials, HA and tricalcium phosphate (TCP) were most commonly used for bone regeneration in combination with GBR and well accepted due to lack of possible disease transmission . Porous and non-porous HA materials reveal a high volume stability but are considered to be nonresorbable or slowly resorbable. In contrast, materials consisting of TCP were reported to be bioabsorbable but subsequently lack sufficient steadiness . Recently, a new biphasic calcium phosphate (Straumann Bone Ceramic ® ; SBC) consisting of 60% HA and 40% β-TCP was introduced as an alternative bone graft substitute, combining the favourable properties of both components. Preliminary experimental animal studies have pointed to an intimate contact between newly formed bone and the remaining graft material . After 8 weeks of healing, J ensen et al. reported a significantly higher amount of bone formation (BF) in defects treated with autografts or β-TCP. Similarly, at 9 weeks, SBC exhibited a significantly higher amount of non-mineralized tissue (NMT) within the regenerated area compared with GBO . Since there was no difference noted in BF at 24 weeks , it could be suggested that initial mineralization varied between both types of bone graft substitutes. Conventional histology is not appropriate to further elucidate the complex biological pattern of wound healing during the initial stages of graft integration.
A recent experimental animal study investigated the histological and immunohistochemical characteristics of a GBO and SBC supported GBR procedure in dehiscence-type defects at titanium implants . Immunohistochemical labelling of non-decalcified tissue sections merely involved a qualitative assessment of the osteocalcin (OC) antigen reactivity within the area of newly formed mineralized tissue (MT) at 1, 4 and 9 weeks. Based on this evaluation, a distinctive difference between both groups could not be observed. The aim of the present study was to further elucidate the immunohistochemical characteristics of wound healing in both groups employing a quantitative assessment of angiogenesis, collagen type I (CI) and OC within the complete defect area including NMT and MT compartments.
Material and methods
The animals, used in this experiment, have been described previously . Briefly, 6 beagle dogs (age 20–24 months, mean weight 14.8 ± 0.6 kg) exhibiting a fully erupted permanent dentition were used. During the experiment, the dogs were fed once per day with a soft food diet and water. Animal selection, management, and surgery protocol were approved by the Animal Care and Use Committee of the Heinrich Heine University and the Bezirksregierung Düsseldorf. The experimental segment of the study started after an adaptation period of 4 weeks.
The study design has been described previously . In brief, extraction of the mandibular and maxillary second, third and fourth premolars and the first and second molars (P2–M2) was performed bilaterally in all dogs. After a healing period of 4 months, standardized buccal dehiscence-type defects were bilaterally created following implant site preparation in the upper jaws ( n = 4 defects per animal). Subsequently, 4 titanium implants were inserted per animal, and the defects randomly assigned to either a GBO or SBC supported GBR procedure. Accordingly, each animal received both types of bone graft substitutes in the upper jaws. Randomization was based on a computer generated list (RandList ® , DatInf GmbH, Tübingen, Germany). Two animals were killed after 1, 4, and 9 weeks of submerged healing.
Surgical procedure
The surgical procedure has been described previously . Briefly, intramuscular sedation was performed with 0.17 mg/kg acepromazine (Vetranquil 1%, CevaTiergesundheit, Düsseldorf, Germany), and anaesthesia was initiated using 21.5 mg/kg thiopental sodium (Trapanal 2.5%, Altana GmbH, Konstanz, Germany). Inhalation anaesthesia for all surgical procedures was oxygen and nitrous oxide and isoflurane. To maintain hydration, all animals received a constant rate infusion of lactated Ringer’s solution whilst anaesthetized. For intraoperative analgesia, intravenous injection of 0.4 mg/kg piritramid (Dipidolor ® , Janssen-Cilag GmbH, Neuss, Germany) and 4.5 mg/kg carprofene (Rimadyl ® , PfitzerPharma GmbH, Karlsruhe, Germany) was given. For postoperative analgesia, piritramid and carprofene were applied subcutaneously for 3 days in the same dose as described above. In addition, prophylactic clindamycine (Clerobe ® , Pharmacia Tiergesundheit, Erlangen, Germany) (11.0 mg/kg body weight) was administered intra- and postoperatively for 3 days.
P2–M2 were carefully removed bilaterally in both jaws after reflection of mucoperiosteal flaps and tooth separation during the first surgery. Wound closure was achieved using mattress sutures and the sites were allowed to heal for 4 months.
In the second surgery, midcrestal incisions were performed to reflect mucoperiosteal flaps at the respective sites for implant insertion in the upper jaws. Preparation of surgical implant sites was performed bilaterally, at a distance of 20 mm apart, using a low-trauma surgical technique under copious irrigation with sterile 0.9% physiological saline.
Standardized buccal dehiscence-type defects (4 mm in height from the crestal bone, 2 mm in depth from the surface of the buccal bone, and 3 mm in width mesio-distally) were created with a straight fissure carbide bur. All osteotomy procedures were performed under copious irrigation with sterile 0.9% physiological saline. A periodontal probe (PCP12, Hu-Friedy Co., Chicago, Illinois, USA) was used to ensure standardization of the defect size. Chemically modified sand-blasted, large grit and acid-etched titanium implants (SLActive ® , Standard Plus Implant, Institut Straumann AG, Basel, Switzerland) (diameter 3.3 mm, length 8 mm) were inserted with good primary stability (lack of clinical implant mobility) so that the borderline between the bony and transmucosal part of the implant coincided with the bone crest (BTB) ( Fig. 1 a ).

According to a split-mouth design, the defects were randomly augmented with either GBO (Geistlich BioOss ® Collagen, Geistlich, Wolhusen, Switzerland) (pore diameters 300–1500 μm, particle size 0.25–1 mm, total porosity 70–75%), or SBC (Straumann Bone Ceramic ® , Institut Straumann AG, Basel, Switzerland) (pore diameters 100–500 μm, particle size 0.5–1 mm, total porosity 90%). Both GBO and SBC particles were moistened in sterile saline for 5 min before placement into the defect. Following grafting, each defect site was covered by a non-cross-linked porcine derived type I and III bilayered collagen membrane (Geistlich BioGide ® , Geistlich, Wolhusen, Switzerland) (BG). Each membrane was trimmed and adapted over the entire defect so as to cover 2–3 mm of the surrounding alveolar bone and to ensure stability of the graft materials. Neither sutures nor pins were used for membrane fixation or stabilization ( Fig. 1 b).
Following periosteal-releasing incisions, the mucoperiosteal flaps were advanced, repositioned coronally and fixed with consecutive as well as vertical or horizontal mattress sutures (Resorba ® , Nürnberg, Germany) to ensure submerged healing conditions ( Fig. 1 c). All surgical procedures were performed by the same experienced operator.
Retrieval of specimens
After healing periods of 1, 4, and 9 weeks, 2 animals each were killed by an overdose of sodium pentobarbital 3%. The oral tissues were fixed by perfusion with 10% buffered formalin administered through the carotid arteries. Dissected blocks containing the experimental specimens were obtained and fixed in 10% neutral buffered formalin solution for 4–7 days.
Histological preparation
Histological preparation of the tissue biopsies has been described previously . In brief, dehydration was performed using ascending grades of alcohol and xylene. Tissue specimens were infiltrated and embedded in methylmethacrylate (Technovit 9100 NEU, Heraeus Kulzer, Wehrheim, Germany) and prepared for non-decalcified sectioning. During this procedure, any negative influence of polymerization heat was avoided by controlled polymerization in a cold atmosphere (−4 °C). The specimens were completely polymerized after 20 h. Each titanium implant was cut in the bucco-oral direction along with its long axis using a diamond wire saw (Exakt ® , Apparatebau, Norderstedt, Germany). Serial sections were obtained from the central aspect of each defect site, resulting in 3–6 sections of approximately 300 μm in thickness each . All specimens were glued with acrylic cement (Technovit 7210 VLC, Heraeus Kulzer, Wehrheim, Germany) to silanized glass slides (Super Frost, Menzel GmbH, Braunschweig, Germany) and ground to a final thickness of approximately 40 μm. One part of the sections was stained with Masson Goldner Trichrome (MG) , whilst the other part was prepared for immunohistochemical labelling.
Immunohistochemical labelling
For immunohistochemistry all tissue section were deplasted in xylol (2× 30 min) followed by treatment with 2-methoxyethylacetate (2× 20 min) and acetone (2× 5 min). After rehydration in phosphate buffered saline (PBS), antigen unmasking was performed by incubating the slides for 15 min in trypsin (PAA Laboratories GmbH, Pasching, Austria) (0.05% in PBS) at 37 °C. After washing with PBS the activity of endogenous peroxidase was quenched with 0.9% hydrogen peroxide in PBS for 10 min at room temperature, the specimens were washed and non-specific binding sites were blocked with a blocking solution for 30 min (Dako Cytomation, Hamburg, Germany). The primary mouse monoclonal antibody to CI (1:60 dilution) (Acris Antibodies GmbH, Hiddenhausen, Germany), OC (1:40 dilution) (Acris Antibodies GmbH), transglutaminase II (TG) (1:40 dilution) (Labvision, Fremont CA, USA), and the corresponding unspecific antibody (mouse IgG 1 ), respectively, as negative control were applied to tissue sections in a humidified chamber and incubated overnight at 8 °C. The cross-reactivity of these antibodies with canine tissues has been extensively documented in previous studies .
The slides were washed in PBS and incubated with secondary biotinylated anti-mouse antibody (1:50 dilution) for 90 min at room temperature. After washing in PBS, the presence of antibody–antigen complexes was visualized using a streptavidin-peroxidase solution (1:250 dilution) (Acris Antibodies GmbH) and AEC (3-amino-9-ethylcarbazole) as the chromogen (Acris Antibodies GmbH).
Histomorphometrical analysis
A colour CCD camera (Color View III, Olympus, Hamburg, Germany) was mounted on a binocular light microscope (Olympus BX50, Olympus, Hamburg, Germany) and used for the acquisition of digital images. Tissue sections were evaluated at an original magnification ×100 using a software program (analySIS FIVE docu ® , Soft Imaging System, Münster, Germany).
A calibrated and masked examiner identified the augmented area (AA) (mm 2 ) from the bottom of the bone defect (BD) to the most coronal level of the bone graft particles underneath the barrier membrane ( Fig. 2 a ). The antigen reactivity of CI, OC, and TG was automatically estimated by the image analysis software as a percentage of NMT (mm 2 ) within AA ( Fig. 2 b). This also included the amount of NMT within any area of newly formed MT.
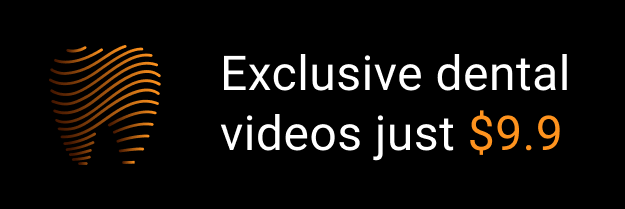