Imaging in orthodontics has evolved from cephalometric and extraoral films, manual cephalometric tracings, to digital imaging and intraoral scanners. Software-assisted cephalometric tracings and three-dimensional image analysis have become routine in orthodontic diagnosis and treatment planning. Determination of biologic boundaries of orthodontic treatment and evaluation of temporomandibular joints and airway became part of orthodontic assessment. Use of advanced imaging and software to digitally plan the orthognathic surgery and accurately predict a successful outcome are now integral to orthodontic practice. This article discusses radiographic methods used in cephalometric analysis and craniofacial growth and development for a predictable orthodontic assessment and treatment planning.
Key points
- •
Historically, 2D imaging first provided a method for evaluating the relationship between the cranium and the dentition.
- •
Standardized methods allowed for research in growth and development.
- •
3D intraoral scanning and imaging techniques are a game changer and have paved the path for:
- ○
3D analysis, diagnosis, and virtual treatment planning.
- ○
Determination of the biologic boundaries of orthodontic treatment.
- ○
3D evaluation of the TMJ.
- ○
Airway analysis methodology.
- ○
Introduction
According to the current practice guidelines published by the American Association of Orthodontists the following diagnostic imaging in orthodontics are considered as part of the diagnostic records:
- 1.
Intraoral and/or panoramic radiographs to assess the condition and developmental status of the teeth and hard tissue supporting structures, and to identify any dental anomalies or pathology.
- 2.
Radiographic imaging to permit relative evaluation of the size, shape, and positions of the relevant hard and soft tissue craniofacial structures including the dentition, and to aid in the identification of skeletal anomalies and/or pathology.
- 3.
Posterior-anterior (PA) cephalometric and lateral cephalometric radiographs may be considered as part of the diagnostic imaging. Three-dimensional (3D) cone-beam computed tomography (CBCT) may be used as an imaging source to obtain this information.
Additionally, for patients still in the growth and development stage, a hand-wrist film for evaluating the level of skeletal maturation can also be considered.
In the age of the digital workflow era, intraoral scanners have permitted clinicians to replace impressions and plaster casts with accurate virtual casts (e-models) that become part of the diagnostic record. These can also be used to print 3D models for appliance fabrication and so forth. 3D radiographic imaging merged with intraoral scanned stereolithography files have become a powerful technological tool for 3D virtual planning of orthodontic and orthognathic surgery cases.
In recent years, the introduction of CBCT imaging has provided clinicians with the option to extract two-dimensional (2D) images from a full-volume CBCT. Although 3D analysis is gaining popularity, it does have a learning curve in that accurate positioning and proper landmark identification is challenging. With further studies, standardized methodology with new norms may be required. With the introduction of more sophisticated software, the incorporation of artificial inteligence methods, and the value-added information gained from 3D analysis, using this powerful tool for 3D diagnosis will continue to gain acceptance as a routine part of orthodontic diagnosis and treatment planning.
As Ricketts stated in 1960, you cannot know how to treat a case until you know what you are treating. The purpose of a through clinical examination combined with appropriate diagnostic records is to provide the clinician with enough information to “know what you are treating.”
Digital intraoral scans
As part of the diagnostic record, intraoral scans are replacing impressions and plaster models with “digital impressions,” “e-models,” and 3D printed casts. There are many advantages to this diagnostic record modality. Scans are electronically stored with patient records, thus eliminating model storage and allowing authorized users to view patient records when not physically present in the office. Files are sent electronically to authorized dental laboratories, eliminating concerns of proper disinfection and saving delivery time. Virtual diagnostic setups can be digitally completed, and treatment plans are viewed by multiple clinicians simultaneously without being physically present when treatment planning multidisciplinary cases. In the current age of COVID, this provides the additional advantage of minimizing in person contact ( Figs. 1–3 ).



Panoramic radiograph
Although magnification and distortion factors must be taken into consideration, a properly exposed panoramic radiograph provides the clinician with valuable 2D information to view normal structures and to identify the presence of any abnormalities or pathologic conditions. It provides an overview of the cervical vertebrae and areas adjacent to the hyoid bone, areas of calcifications may be visible, condylar shape, ramus heights, the inferior alveolar canal and mental foramen, the maxillary sinuses, the nasal cavity and septum, the orbit, dental development, pattern of eruption, the dentition and a broad overview of the supporting structures associated with it. Asymmetrical growth may also be detected first on a panoramic radiograph when there are large differences in ramus heights between the right and left sides. Any area that does not appear normal or there is suspicion of an underlying pathologic condition requires further investigation. Fig. 4 shows the panoramic image of a 13-year-old patient in the permanent dentition stage with condylar resorption and asymmetric ramus heights. Roots of the premolars are shorter and the developing third molars will require further evaluation later. Rotation of the mandibular canines, lower incisor crowding, prominent marginal ridges, and prominent cingulum of the maxillary incisors is also observed.

Skeletal maturation indicators
Hand-Wrist Radiograph
It has been recognized and well established that chronologic and skeletal maturation ages do not necessarily correlate. As Fishman noted in 1979, all too often, the timing of orthodontic treatment was determined by chronologic age and the stage of dental development, neither of which are reliable to establish a child’s skeletal age.
In 1959, Pyle and coworkers established a radiographic atlas of skeletal development using hand-wrist films. Practitioners have used this as a reference to estimate the age of their patients. Bjork and Helm reported a close association between the age at maximal growth and the age when ossification of the ulnar metacarpophalangeal sesamoid of the thumb occurred. In 1981 Fishman established a clinically oriented method based on hand-wrist films to determine skeletal maturation. Fishman’s SMI method uses four stages of bone maturation using six anatomic sites on the thumb, third finger, fifth finger, and the radius. From these anatomic sites, 11 discrete stages of skeletal maturation (SMI 1–11) have been established. The 11 stages of skeletal maturation are described in Box 1 . This method continues to be used by many clinicians today and the reader is referred to Steven Wang and Brian Ford’ article, “ Imaging in Oral and Maxillofacial Surgery ,” in this issue. Fishman’s work for a more detailed understanding of this methodology.
-
Width of epiphysis as wide as the diaphysis:
-
SMI 1: Third finger-proximal phalanx
-
SMI 2: Third finger-middle phalanx
-
SMI 3: Fifth finger-middle phalanx
-
-
Ossification:
-
SMI 4: Adductor sesamoid of thumb
-
-
Capping of epiphysis:
-
SMI 5: Third finger-distal phalanx
-
SMI 6: Third finger-middle phalanx
-
SMI 7: Fifth finger-middle phalanx
-
-
Fusion of epiphysis and diaphysis:
-
SMI 8: Third finger-distal phalanx
-
SMI 9: Third finger-proximal phalanx
-
SMI 10: Third finger-middle phalanx
-
SMI 11: Radius
-
The hand-wrist radiograph in Fig. 5 is that of an adolescent patient. When this radiograph is obtained, it is preferable to have a clear image of the hand-wrist complex, including the radius and the ulna. In the image for this patient, the capping stage on the middle phalanx of the fifth digit is not as clearly visible, most probably caused by the fifth finger positioning during the exposure. The abductor sesamoid is visible, capping is seen on the middle phalanx of the third finger, and there is no fusion on the distal phalanx of the third finger. Most probably, this patient would be categorized as an SMI 7 based on Fishman’s work.

Other methods for determining the level of skeletal maturity have been reported. McNamara and Franchi reported using the cervical vertebral maturation method as a guide to determine the skeletal maturation stage. With this method, data from the second, third, and fourth cervical vertebrae are used based on the morphology. In summary, the vertebral bodies generally have a flat inferior border in the prepubertal stage and transform to a more concave inferior border with C3 and C4 remaining vertically shorter in the circumpubertal stage and elongation of C3 and C4 is evident in the postpubertal stage. The reader is referred to the work of McNamara and Franchi for a more detailed understanding of this methodology.
Two-Dimensional Lateral Cephalometric Analysis
The application of anthropometric measurements was introduced to orthodontic clinicians in the early part of the twentieth century. Most orthodontists at the time were evaluating dental discrepancies primarily by the interrelation of the teeth within the jaws. In 1931, the advent of the cephalostat by Broadbent enabled clinicians to accurately use established craniometric landmarks to evaluate skeletal and dental relationships and also introduce soft tissue findings as it relates to the position of the jaws and the dentition.
The Miriam-Webster dictionary defines cephalometric as the science of measuring the head in living individuals. This definition is further elaborated as the measurement and study of craniofacial proportions, the dentition, and the soft tissues as they relate to one another. In the skeletally immature patient, this also includes growth and development. The concept of using angles and linear measurements for evaluating facial proportions dates back to the fifteenth century when Leonardo da Vinci used it to study facial form. The first truly scientific attempt of a cranial measurement was reported by Spigel in the sixteenth century and was coined the term “lineae cephalometricae.” The measurement consisted of four lines: (1) facial, (2) occipital, (3) frontal, and (4) the sincipital. In a well-proportioned skull, it was believed that these lines should be equal to one another.
From the sixteenth to the twentieth century, numerous craniologists developed various types of analysis (eg, Camper, DeSchamps, Daubeuton, Broca, Bell, Gibson, Soemmerring, Blumenbach, Retzius, Barclay, Huxley, and Topinard). At the meeting of the thirteenth general congress of the German Anthropological Society, contributions were made that led to the development of what is referred to today as the Frankfort horizontal plane.
Roentgen’s development of X-rays in 1895 paved the path for development of the cephalostat by Hofrath in Dusseldorf and Broadbent in Cleveland. This development was a significant breakthrough because most orthodontists at the time were evaluating dental discrepancies primarily by the interrelation of the teeth using plaster models. Dental casts have limitations in that the articulation of casts may provide a reasonably good evaluation of how the teeth interrelate with each other but do not provide an accurate assessment of their relationship with the cranium. Additionally, this can provide a misrepresentation of the dental position relative to the jaw position. It is possible for teeth to be projected differently depending on how the dentition is oriented on a plaster model. Another limitation is that the growth and development cannot be assessed by dental casts. A standardized method using a cephalostat provided a great opportunity for researchers to study growth and development. Therefore, the advent of the cephalostat allowed clinicians to use radiographs for the purpose of evaluating growth and for the evaluation of interrelationships between the dentition, the jaws, the cranium, and the face and thus provide a method for determining skeletal diagnosis, treatment planning, monitoring of treatment, evaluation of the post-treatment outcome, and the detection of asymmetric growth patterns.
The dynamic pattern of growth and development also has a differential component. Based on the postnatal growth and development reported by Scammon , the brain case follows the neural growth curve gradient, whereas the face and the dentition more closely follow the general growth pattern. With this information it is possible to identify which landmarks may remain stable and can be used for comparison purposes to study growth, diagnose and treat, monitor treatment, and evaluate the post-treatment results based on superimposition of determined stable structures.
Cephalometric analysis first requires a thorough understanding of internal and external skull anatomy and the overlying soft tissue before anatomic landmarks consisting of hard and soft tissue structures are identified. The reader is referred to any major textbook of anatomy or an anatomic atlas to review this information. Although the introduction of numerous analyses has resulted in identifying additional landmarks, many of the important anthropometric points still used today were defined by the first cephalometric workshop held in 1957 at Western Reserve University. The purpose of the workshop was primarily to define cephalometric points and planes, to standardize the technique, to clarify interpretation, and to evaluate clinical applications. Contributions by Krogman and Sassouni were also studied during this workshop, the proceedings of which went on to define and validate the skeletal cephalometric landmarks ( Table 1 ).
A point | Subspinale | The deepest, most posterior midline point on the premaxilla between the anterior nasal spine and prosthion (alveolar point) [Downs]. |
ANS | Anterior nasal spine | The tip of the anterior nasal spine seen on the radiograph film from norma lateralis. Also referred to as the sharp bony process of the anterior maxilla at the lower margin of the anterior nasal opening. |
Ar | Articulare | The point of intersection of the dorsal contours of process articularis mandibulae and the occipital bone (os temporale) [Björk]. A junction point between the posterior border of the ramus and the inferior border of the posterior cranial base. |
B | Supramentale | The most posterior point in the concavity between infradentale and pogonion [Downs]. |
Ba | Basion | The lowermost point on the anterior margin of the foramen magnum in the midsagittal plane. |
Bo | Bolton point | The highest point in the upward curvature of the retrocondylar fossa [Broadbent] located at the intersection of the outline of the occipital condyle and foramen magnum. |
Gn | Gnathion | The most inferior point in the contour of the chin usually between pogonion and menton. |
Go | Gonion | The point that on the jaw angle is the most inferiorly, posteriorly, and outwardly directed. Located on the outer curvature of the angle of the mandible. Constructed gonion is located at the intersection formed by the lines tangent to the posterior ramus and the inferior border of the mandible. |
Me | Menton | The lowermost point on the symphyseal shadow in norma lateralis. |
Na | Nasion | The intersection of the internasal suture with the nasofrontal suture in the midsagittal plane. |
Or | Orbitale | The lowest point on the lower margin/inferior border of the bony orbit. |
PNS | Posterior nasal spine | The tip of the posterior spine of the palatine bone in the hard palate. |
Po | Porion | The midpoint on the upper edge of the porus acusticus externus located by means of the metal rods on the cephalometer [Björk]. It is the most superiorly positioned point of the external auditory meatus. This is a very important landmark still used routinely; however, the metal rods may not allow for accurate location of anatomic porion and it may be significantly further away. , |
Pog | Pogonion | Most anterior point in the contour of the chin. |
Ptm | Pterygomaxillary fissure | The lowest point on the teardrop shape of the projected contour of the fissure; the anterior wall represents closely the retromolar tuberosity of the maxilla, and the posterior wall represents the anterior curve of the pterygoid process of the sphenoid bone. |
“R” | Broadbent registration point | The midpoint of the perpendicular from the center of sella turcica to the Bolton plane. |
S | Sella turcica | The midpoint of sella turcica, as determined by inspection. Identified as the geometric center of the pituitary fossa. |
SO | Sphenooccipital synchondrosis | The upper most point of the suture. |
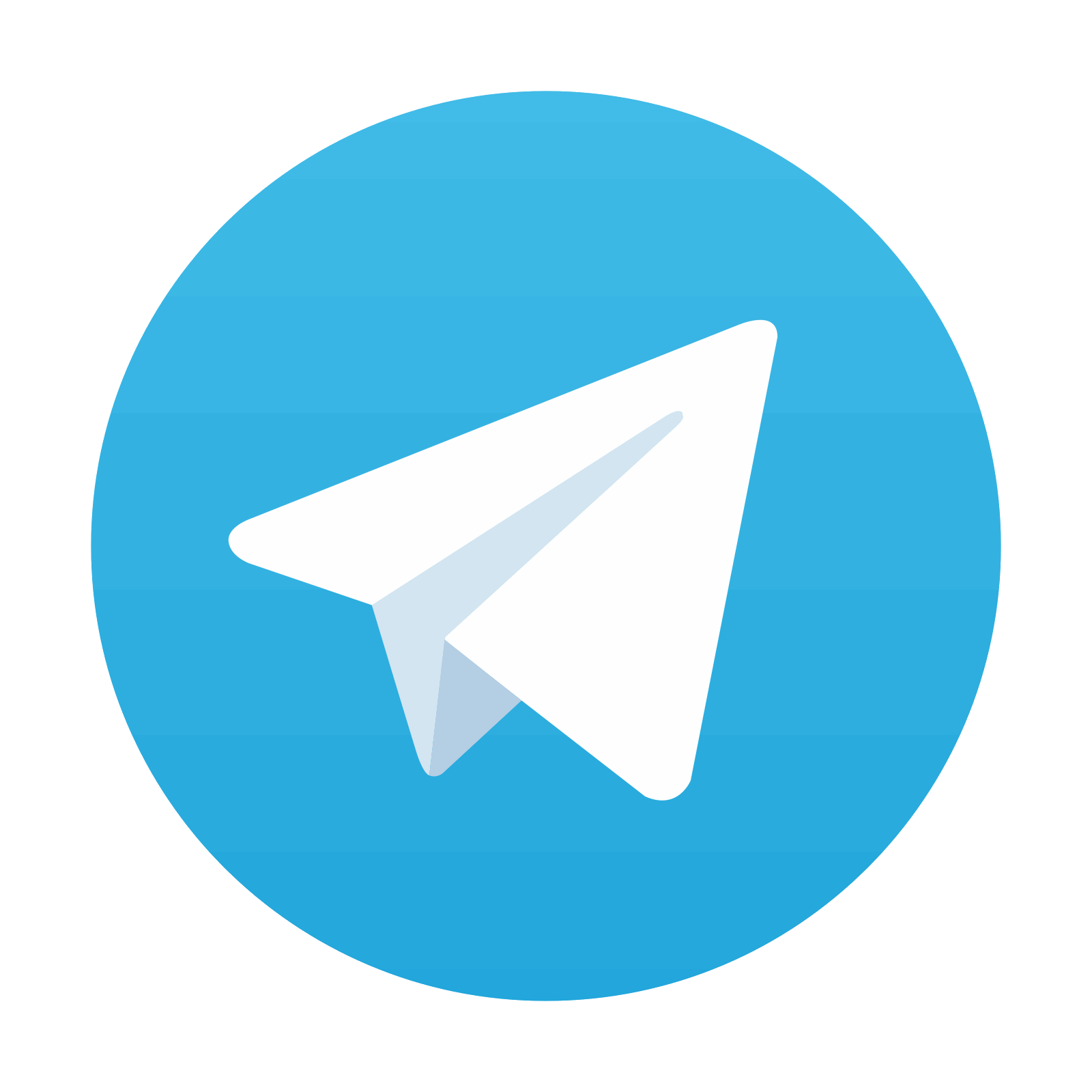
Stay updated, free dental videos. Join our Telegram channel

VIDEdental - Online dental courses
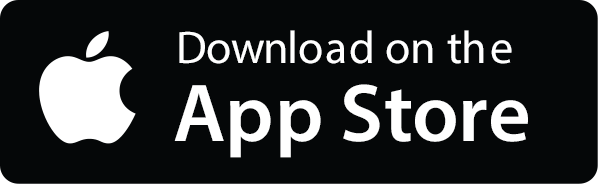
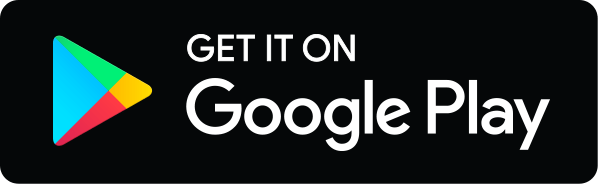
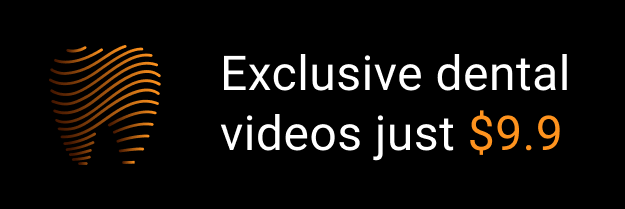