Abstract
Radioresistance is one of the main determinants of treatment outcome in oral cancer, but the prediction of radioresistance is difficult. The authors aimed to establish radioresistant oral squamous cell carcinoma (OSCC) cell lines to identify genes with altered expression in response to radioresistance. To induce radioresistant cell lines, the authors treated OSCC cell lines with an accumulated dosage of 60 Gy over 30 cycles of radiotherapy. They compared the results from cDNA arrays and proteomics between non-radiated and radioresistant cell lines in order to identify changes in gene expression. Western blot analysis was used to validate the results. The cDNA array revealed 265 commonly up-regulated genes and 268 commonly down-regulated genes in radioresistant cell lines, 30 of which were cancer-related genes. Proteomics identified 51 proteins with commonly altered expression in radioresistant cell lines, 18 of which were cancer-related proteins. Both the cDNA array and proteomics indicated that NM23-H1 and PA2G4 were over-expressed. Western blot analysis showed increased expression of NM23-H1, but not PA2G4, in radioresistant cell lines. The authors concluded that NM23-H1 may be a radioresistance-related gene and over-expression of NM23-H1 could serve as a biomarker to predict radioresistance in OSCC.
Cancers involving the oral cavity account for 2–3% of all malignancies. Typical treatments for oral squamous cell carcinoma (OSCC) are surgery, radiotherapy, chemotherapy, or a combination of these. Over the past two or three decades, newly developed surgical skills, updated radiation techniques and numerous promising chemotherapy agents have been introduced, but the survival rate of OSCC patients has not improved significantly. One of the main reasons for this could be that patients with the same gross tumour extent have received almost the same treatment without considering the individual biological behaviour of the tumour because the TNM stage including the gross tumour extent may be the only reliable predictor of tumour behaviour and treatment outcome. Accordingly, new, reliable, personalized markers of outcomes for each treatment modality are needed. The development of a new marker that can predict treatment outcome has been difficult; however, because surgery is not standardized and chemotherapy is complicated due to the use of numerous agents and their different mechanisms of action.
Radiotherapy is highly standardized, thus patients with the same gross tumour extent usually receive radiotherapy using nearly the same techniques, with variable treatment outcomes. Many patients respond well to radiotherapy, but a significant proportion of patients do not respond and they require additional risky surgery that is associated with severe morbidity and a poor survival rate. If there was a way to predict the response to radiation, then OSCC patients predicted to develop radioresistance could be treated initially by surgery followed by adjunctive therapy, which would greatly improve their survival rate. The mechanisms underlying radioresistance in OSCC patients are poorly understood and there is no accurate predictive method for radioresistance.
Over the past decade, associations between radioresistance and the expression of specific genes or alterations in tumour tissue have been reported, and several genes such as ras, raf-1, bcl-2 and survivin have been reported as candidate radioresistance-related genes. Most of these studies were intended for a small set of genes, and they have provided only a partial understanding of the molecular mechanisms underlying radioresistance. It is necessary to analyse comprehensive gene expression patterns related to radioresistance in order to fully understand the process of radioresistance development.
Microarray techniques can detect changes in thousands of genes simultaneously and are thus suitable for investigating the complex genetic mechanisms underlying radioresistance. There have been several studies on the identification of radioresistance-related genes using microarray techniques in head and neck cancer. These studies have detected genetic alternations that occur after cancer cell lines become resistant to radiation, but most previous studies have used radioresistant cancer cell lines induced under dosed radiation with little regard for clinical setting.
In this study, the authors aimed to establish radioresistant OSCC cell lines under conditions that closely resemble a real clinical setting in order to identify changes in gene expression that occur in cancer cell lines after they acquire radioresistance. Both cDNA array analysis and proteomics were used to identify candidate genes related to radioresistance in OSCC cell lines.
Materials and methods
Cell lines and culture
QLL1 cells (a squamous cell carcinoma cell line originating from metastatic lymph nodes in oral cancer) were a generous gift from Dr. J. Shah (Memorial Sloan-Kettering Cancer Center, New York, NY, USA). The SCC15 and SCC25 cell lines (squamous cell carcinoma cell lines originating from the tongue) were purchased from the American Type Culture Collection (Rockville, MA, USA). Cells were cultured in minimum essential medium (MEM) supplemented with 10% foetal bovine serum, 2 mM l -glutamine, penicillin (50 μg/ml) and streptomycin (50 μg/ml).
Establishment of radioresistant cell lines
Cells were cultured in T75 flask until they were 80% confluent and a 2 Gy dose of radiation was delivered at room temperature with a linear accelerator (21iX, Varian). Afterwards 2 Gy doses of radiation were delivered repetitively when cells were grown to 80% confluence after previous radiation until a cumulative dose of 60 Gy was reached.
Lactate dehydrogenase (LDH) assay
LDH released from surviving cells was measured in order to confirm radioresistance in the irradiated OSCC cell lines. LDH activity was compared between 60 Gy irradiated OSCC cell lines and non-irradiated OSCC cell lines after delivering 5 Gy radiation to each group in order to identify differences in radiation-induced cytotoxicity using the CytoTox96 nonradioactive assay (Promega, Madison, WI, USA). One-way ANOVA was used to test for significant differences between the two groups. Statistical analyses were performed using SPSS 11.5 statistical software (SPSS Inc., Chicago, IL, USA). Significance was defined as p < 0.05.
cDNA array
cDNA array was used to detect altered gene expression between raioresistant and non-irradiated OSCC cell lines. mRNA was amplified following the standard CMRC (Cancer Metastasis Research Center, Seoul, Korea) protocol, which was modified slightly from a previous method based on T7 linear amplification. Amplified mRNA was transcribed from double-stranded cDNA using a T7 MEGAscript kit (Ambion, Austin, TX, USA) in vitro. The quantity and quality of total and amplified RNA were determined using an ND-1000 spectrophotometer (NanoDrop Technologies, DE, USA) and agarose gel electrophoresis. Gene expression analyses were performed using a human microarray (CMRC-GT, Seoul, Korea) containing 17,104 known genes and ESTs in reference design after extracting total RNA from radioresistant and non-irradiated OSCC cell lines. The RNA from the radioresistant OSCC cell lines was labelled with Cy5 and separately co-hybridized with Cy3-labelled non-irradiated SCC15, SCC25, and QLL1 RNA.
Hybridization and washing procedures were carried out following a modified CMRC protocol. Briefly, 2 μg of amplified mRNA was labelled with Cy3- or Cy5-dUTP during reverse transcription. The final probe was concentrated to 40 μl using a Microcon YM-30 column (Millipore, Bedford, MA, USA) and denatured at 100 °C for 2 min.
After washing and drying the hybridized microarray, fluorescence signals were acquired using a GenePix 4000B scanner (Axon Instruments, Foster City, CA, USA). Scanned images were processed using GenePix Pro 4.1 software (Axon Instruments, Foster City, CA, USA).
To confirm the reproducibility of the microarray experiment, the authors performed three replicate experiments with randomly selected samples. The replicate experiments were located side-by-side using an unsupervized hierarchical clustering method with higher correlation. The mean value of the replicates was used in subsequent analyses.
Proteomics
Three radioresistant and three non-irradiated control OSCC cell line pellets were washed twice with ice-cold phosphate buffered saline (PBS) and sonicated for 10 s using a Sonoplus system (Bandelin Electronics, Germany) in sample lysis solution composed of 7 M urea, 2 M thiourea containing 4% (w/v) 3-[(3-cholamidopropy) dimethyammonio]-1-propanesulphonate (CHAPS), 1% (w/v) dithiothreitol (DTT) and 2% (v/v) pharmalyte and 1 mM benzamidine. Protein loading was normalized using the Bradford assay.
Immobilized pH gradient (IPG) dry strips were equilibrated for 12–16 h with 7 M urea, 2 M thiourea containing 2% 3-[(3-cholamidopropy) dimethyammonio]-1-propanesulphonate (CHAPS), 1% DTT, and 1% pharmalyte, and loaded with 200 μg of sample each. Sodium dodecyl sulphate polyacrylamide gel electrophoresis (SDS-PAGE) was performed using the Hoefer DALT 2D system (Amersham Biosciences, UK) following the manufacturer’s instructions. Quantitative analysis of the digitized images was carried out using PDQuest (version 7.0, BioRad) software according to the protocols provided by the manufacturer. Protein spots were enzymatically digested in gel in a manner similar to that previously described by Shevchenko et al., using modified porcine trypsin.
Protein analyses were performed using an Ettan MALDI-TOF (Amersham Biosciences, UK). The ProFound search program, developed by The Rockefeller University ( http://129.85.19.192/profound_bin/WebProFound.exe ), was used for protein identification by peptide mass fingerprinting.
Western blot analysis
Sample proteins and controls were resolved by PAGE. In each well, 10 μg/20 μl proteins were loaded. Proteins were then transferred to nitrocellulose membranes using standard methods (Invitrogen, Carlsbad, CA, USA). Blots were blocked with 10% non-fat dried milk freshly made in 1XPBS-T, rocked on a rotating shaker for 2 h at room temperature, and rinsed three times in 1XPBS-T. The blots were incubated O/N at 4 °C with primary antibodies diluted 1:500 in PBS-T. Primary antibodies were subsequently rinsed three times in 1XPBS-T. The blots were then probed with an enzyme-linked secondary antibody (horseradish peroxidase; Amersham, UK) in 1XPBS-T (1:1500) for 1 h at room temperature. Excess secondary antibody was rinsed off with three 5-min rinses in 10 ml 1XPBS-T. Finally, chemiluminescent detection reagents were used to reveal the results. Immunoblotting for GAPDH (goat, polyclonal; Santa Cruz Biotechnology) was carried out to verify equivalent protein loading. Densitometric analyses of the Western blots were carried out using TINA 2.0 software (Raytest Isotopenmessgerate, Straubenhardt, Germany).
Results
LDH assay
The LDH assay confirmed that all three irradiated OSCC cell lines became radioresistant. After delivering 5 Gy radiotherapy to each of the irradiated and non-irradiated control OSCC cell lines, the cytotoxic effect was found to be significantly more severe in the non-irradiated control OSCC cell lines ( Fig. 1 ).
cDNA array
Of 17,104 genes, the authors obtained 14,456 genes with no missing portion. Hierarchical clustering analysis was performed with Cluster software, in which the clustering algorithm was used with a complete linkage and Pearson’s correlation analysis, and the resulting dendrogram was visualized using TreeView software ( http://rana.lbl.gov/EisenSoftware.htm ) ( Fig. 2 ).
As a validation step, the authors compared the significance of selected genes between the expression profiles of radioresistant OSCC cell lines and non-irradiated control cell lines using the Significance Analysis of Microarray statistical program (SAM; ). QLL1 cells had 1473 differentially expressed genes after becoming radioresistant with 728 up-regulated genes and 745 down-regulated genes. The SCC15 line had 4040 differentially expressed genes with 2185 up-regulated genes and 1855 down-regulated genes. SCC25 cells showed 1551 differentially expressed genes with 692 up-regulated genes and 859 down-regulated genes. In total, there were 256 common up-regulated genes and 268 common down-regulated genes in all three cancer cell lines ( Fig. 3 ).
The annotation of selected genes was performed using the Database for Annotation, Visualization and Integrated Discovery (DAVID; ) and the Stanford Online Universal Resource for Clones and Expressed sequence tags (SOURCE; ). Among the 533 genes showing altered expression after radioresistance was acquired in all three OSCC cell lines, the authors identified 30 cancer-related genes through Ingenuity Systems ( www.ingenuity.com ) ( Table 1 ).
ID | Gene name | Description | Fold change |
---|---|---|---|
Proliferation | |||
AA677403 | CGA | Glycoprotein hormones, alpha polypeptide | 3.1 |
W86182 | PNN | Pinin, desmosome associated protein | 2.54 |
AW008966 | TCEB2 | Transcription elongation factor B (SIII), polypeptide 2 (18 kDa, elongin B) | 2.48 |
AA488332 | PA2G4 | Proliferation-associated 2G4, 38 kDa | 2.36 |
AA446246 | NRAS | Neuroblastoma RAS viral (v-ras) Oncogene homolog | 2.2 |
H68885 | PHLDA2 | Pleckstrin homology-like domain, family A, member 2 | 2.1 |
AA464856 | ID4 | Inhibitor of DNA binding 4, dominant negative helix-loop-helix protein | −2.283 |
Migration | |||
AA410383 | CXCL13 | Chemokine (C-X-C motif) ligand 13 (B-cell chemoattractant) | 2.67 |
AA434159 | GIPC1 | GIPC PDZ domain containing family, member 1 | 2.41 |
AI936909 | MAPK12 | Mitogen-activated protein kinase 12 | 2.02 |
R39862 | ALCAM | Activated leucocyte cell adhesion molecule | −2.336 |
DNA repair | |||
AA620446 | RECQL4 | RecQ protein-like 4 | 2.92 |
AA991871 | FOXA1 | Forkhead box A1 | 2.87 |
N93924 | RFC4 | Replication factor C (activator 1) 4, 37 kDa | 2.78 |
AA669314 | ATP5D | ATP synthase, H+ transporting, mitochondrial F1 complex, delta subunit | 2.62 |
AA933835 | ENTPD1 | Ectonucleoside triphosphate diphosphohydrolase 1 | 2.59 |
H90415 | BRCA1 | Breast cancer 1, early onset | 2.34 |
AAO85436 | NM23-H1 | Non-metastatic cells 1, protein (NM23A) expressed in | 2.09 |
Cell cycle | |||
AA478066 | PKMYT1 | Protein kinase, membrane associated tyrosine/threonine 1 | 2.58 |
AA922727 | FGF4 | Fibroblast growth factor 4 | 2.36 |
N54551 | PPP5C | Protein phosphatase 5, catalytic subunit | 2.12 |
AA486280 | TIMP2 | TIMP metallopeptidase inhibitor 2 | 2.11 |
AI361153 | SRF | Serum response factor | 2.09 |
AI355949 | CALM3 | Calmodulin 3 (phosphorylase kinase, delta) | 2.07 |
Cell death | |||
AA931820 | BCL2L1 | BCL2-like 1 | 2.87 |
AA417746 | DMTF1 | Cyclin D binding myb-like transcription factor 1 | −2.352 |
R68555 | PDCD10 | Programmed cell death 10 | −2.255 |
AA482119 | ID3 | Inhibitor of DNA binding 3, dominant negative helix-loop-helix protein | −2.185 |
AA134814 | TANK | TRAF family member-associated NFKB activator | −2.176 |
AA411814 | PSEN1 | Presenilin 1 (Alzheimer disease 3) | −2.142 |
Proteomics
Two-dimensional gel electrophoresis (2-DE) and image analysis revealed that 449 spots showed altered expression after radioresistance was acquired, from among approximately 1500 spots in all three OSCC cell lines after proteomics analysis. Among them, 57 spots showed statistically significant changes in expression ( p < 0.05; Fig. 4 ). The authors identified 45 up-regulated proteins and six down-regulated proteins associated with radioresistance using protein profile analysis and identification by mass spectrometry, and 18 of these were cancer-related proteins. Those were sorted by Ingenuity Systems, which is a reference based program ( Table 2 ). Among the 533 genes identified by cDNA array analysis and the 51 proteins identified by proteomics, the authors found that the mRNA and protein of both NM23-H1 and PA2G4 were over-expressed.
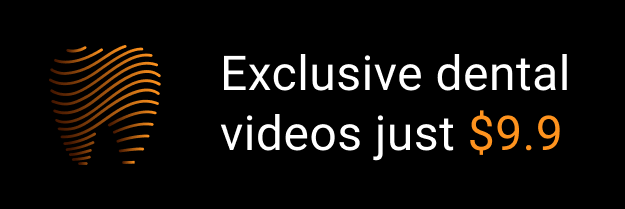