Abstract
Objectives
Methacrylic monomers are released, from dental composite resins, either into the oral cavity or in pulpal tissues, where they can cause local or systemic adverse effects. The mechanisms of these effects are not well understood, probably because such molecules can act at different levels also inducing a depletion of intracellular glutathione (GSH). GSH can detoxify methacrylates by conjugating their α,β-unsaturated carbon–carbon moiety to the thiol group, with the catalysis of glutathione S-transferases (GST). This reaction determines a GSH cellular depletion and belongs to the metabolism of α,β-unsaturated esters, protecting the body against the toxic effects of electrophiles. On the basis of the above considerations, this work aim is to set up a method for the detection of the adducts formed by methacrylic monomers with GSH in cells using HPLC coupled to mass spectrometry (HPLC–MS) and micellar electrokinetic capillary chromatography (MECK) techniques.
Methods and results
Adducts of glutathione with triethylene glycol dimethacrylate (TEGDMA) and hydroxyethyl methacrylate (HEMA) were incontrovertibly identified by HPLC–MS and MECK in human gingival fibroblasts and erythrocytes, both outside and inside cells. Molecular docking simulations of HEMA and TEGDMA in the experimental structure of glutathione S-transferase, are also reported to rationalize the effectiveness of such enzyme in the catalysis of the above described reaction.
Significance
The setup of a method for the identification of GSH-methacrylate adducts allows to determine when the metabolic pathway involving such compounds is employed by cells for the detoxification of monomers leached from composite resins.
1
Introduction
Methacrylic compounds − like triethylene glycol dimethacrylate (TEGDMA) and hydroxyethyl methacrylate (HEMA) − are used as components of polymeric materials commonly employed in various fields including dentistry, where they are present in some types of cements, in endodontic products and, mostly, in dental composite resins . Over the last thirty years these materials have reached a large diffusion throughout the Western world for the treatment of adults and children alike. After performing dental restorations with composite resins, small amounts of uncured molecules are inevitably released into either the oral cavity or – through dentinal diffusion – in pulpal tissues , hence leaching into the blood , a process which may be also facilitated by the mechanical erosion of the material .
In some instances, methacrylic monomers display a variable degree of toxicity and may cause, or contribute to, adverse biological effects like the damages of the oral soft tissues sometimes observed in vivo : it is therefore evident the need of detailed in vitro studies regarding the interactions between methacrylates and host . To understand the reasons of the adverse biological effects, in vitro biocompatibility studies of materials containing methacrylates are today performed by multidisciplinary teams including toxicology, biology and chemistry experts. The toxic effects caused by methacrylates are nevertheless still difficult to unravel probably because such molecules can act at different levels , for example interfering with the cell cycle and DNA synthesis , or increasing the production of reactive oxygen species (ROS) , or inducing a depletion of intracellular glutathione (GSH) . Such ubiquitous tripeptide is produced in all organs and cells where it accomplishes several physiological tasks including relevant protection against oxidative stress performed through the reduction of hydrogen peroxide and lipid peroxides by converting to its oxidized form (GSSG). The latter is either excreted by the cell or reconverted to GSH by the activity of NADPH-dependent GSH reductase or, alternatively, bound to protein thiol groups forming mixed disulfides . GSH can, moreover, also detoxify xenobiotics like methacrylates by conjugating, via a Michael addition , the thiol group with their α,β-unsaturated carbon–carbon moiety ( Patterns 1 and 2 ). The reaction is catalyzed by glutathione S-transferase (GST), one of several enzyme forms belonging to a multi-gene family − present in cytosol, microsomes and mitochondria − involved in detoxification processes . The described reaction, which can also occur in a cell free system , determines GSH cellular depletion as observed in several in vitro studies employing human fibroblasts and HL-60 cells .


The above said process belongs to the metabolism of α,β-unsaturated esters and protects the body against the toxic effects of endo- and xeno-biotic electrophiles. The formation of a bond between the cysteine residue of GSH and an electrophile usually results, in fact, in a less reactive and more water-soluble product , thus facilitating its clearance from the cells. GSH depletion may have also both positive and negative consequences for the cells: in fact, even if methacrylates removal represents a positive event, the decrease of GSH concentration brings many disadvantages like oxidation of protein thiol groups, protein denaturation and induction of mitochondrial permeability transition .
Although the main metabolic pathway for methacrylates detoxification may occur through esterase catalyzed hydrolysis, because only the intact esters are able to overcome the lipophilic barriers , methacrylates conjugation with GSH can nevertheless represent a very important alternative route . On the basis of the above considerations, this work aims to set up a method for the detection of the adducts formed by methacrylic monomers with GSH in gingival fibroblasts and erythrocytes using high performance liquid chromatography coupled to mass spectrometry (HPLC–MS) and micellar electrokinetic capillary chromatography (MECK).
Furthermore, molecular docking simulations of HEMA and TEGDMA in the experimental structure of Glutathione S-transferase P1, (the cytosolic enzyme isoform present in gingival fibroblasts) are also reported to rationalize the effectiveness of such enzyme in the catalysis of the reaction of glutathione with methacrylates .
2
Materials and methods
All chemicals and reagents were supplied by Sigma–Aldrich Srl (Milan, Italy) unless otherwise indicated.
HPLC grade methanol was purchased from J.T. Baker (Baker Mallinckrodt, Phillipsburg, NJ, USA). HPLC grade water (18.2 mΩ) was prepared using a Millipore Milli-Q purification system (Millipore Corp., Bedford, MA, USA).
2.1
Synthesis of glutathione-methacrylate adducts
A solution of GSH (0.8 mmol/L) in phosphate buffer solution (PBS, pH 7.4, 1.0 mL) − bubbled with nitrogen to remove oxygen and inhibit GSH oxidation − was mixed at 37 °C with an equal volume of HEMA (1.0 mmol/L) or TEGDMA (0.4 mmol/L) in PBS ; GSH solution in PBS alone was similarly prepared. The reaction mixtures were analyzed immediately and after 48 h by HPLC–MS and MEKC; likewise GSH, HEMA and TEGDMA in PBS solutions were analyzed as controls.
2.2
Detection of GSH-methacrylate adducts by HPLC–MS and HPLC–UV
GSH-methacrylate reaction mixtures were analyzed by a SURVEYOR MS micro HPLC, (Thermo Finnigan, San Josè, CA, USA) using a Discovery HS C18 column (250 mm × 4.6 mm, 5 μm) (SUPELCO, Supelco Park, Bellefonte, PA, USA) at a flow rate of 1 mL/min. Water (A) and methanol (B) were used to obtain the chromatographic gradients for the elution: (i) for GSH-TEGDMA reaction mixture, from 5% (B) to 65% (B) in 32 min and then to 95% (B) in 8 min, (ii) for GSH-HEMA reaction mixture, from 25% (B) to 65% (B) in 23 min and then 95% (B) in 7 min followed by 10 min of maintenance. In both cases the column effluent was splitted by a “T junction” positioned after the chromatographic column sharing it between an a UV detector set at 214 nm (80%) and an ESI/MS in positive ion mode (20%) using a Finnigan LCQ DECA XP Max ion trap mass spectrometer equipped with Xcalibur® system manager data acquisition software (Thermo Finnigan, San Josè, CA, USA). The capillary voltage was set at 28 V, the spray voltage was set at 4.8 kV and the tube lens offset was set at 15 V. The capillary temperature was 250 °C. Data were acquired in MS1 scanning mode and recorded in the 50–1500 m / z region.
Total ion current (TIC) profile was produced by monitoring, during the chromatographic run, the intensity of all the ions produced and acquired in every scan.
2.3
Detection of GSH-methacrylate adducts by MEKC
To obtain the electrophoretic profiles of HEMA, TEGDMA, GSH and reaction mixtures, a capillary electrophoresis system (P/ACE™ MDQ, Beckman, Richmond, CA USA) with a fused silica capillary (60 cm length, 50 μm inner , Beckman) was used; a 0.2 mm detection window was obtained by a small flame at 10 cm from the cathodic terminal. The running solution was constituted by sodium phosphate/sodium tetraborate buffer (44.4 and 11.1 mmol/L, respectively), pH 7.19, with 36.7 mmol/L SDS as micellar phase and a final content of acetonitrile (10%, v/v). Samples were diluted (1:1) with a solution of sodium phosphate (7.2 mmol/L) and sodium tetraborate (1.8 mmol/L) at pH 7.19 and injected by pressure (0.5 psi) for 10 s at the anodic end of the capillary. After every run the capillary was washed (20 psi) with H 2 O, H 2 O/acetonitrile 1:1, H 2 O, NaOH and finally H 2 O. Each analysis was performed at a constant voltage of 18 kV with a current of nearly 50 μA at a temperature of 35 °C and at a wavelength of 214 nm. The adducts previously isolated and identified by HPLC–MS were also analyzed in the same operative conditions.
2.4
Treatment of red blood cells with methacrylates and detection of the formed adducts
Red blood cell suspensions (1.0 mL, 5% final hematocrit) were incubated in a shaking water bath for 1 h at 37 °C with HEMA (4 mmol/L) or TEGDMA (2 mmol/L) in PBS. As controls, red blood cell suspensions (1.0 mL, 5% hematocrit) in absence of monomers were used. After incubation, the samples were centrifuged (500 × g , 5 min), the precipitated cells were lysed by freezing and proteins were eliminated by precipitation with metaphosphoric acid (5% m/v) and following centrifugation at 15000 × g (10′, 4 °C); the samples were then analyzed by MEKC. The diluted extracellular media (filtered by a 45 μm millipore filter) were also analyzed by MEKC.
2.5
Cell culture of human gingival fibroblasts
Human gingival fibroblasts were obtained (with informed consent) from a healthy patient subjected to gingivectomy of the molar region. Immediately after removal, the tissues were placed in Hanks’ Balanced Salt Solution (HBSS) with penicillin (250 U/mL), streptomycin (0.25 mg/mL), gentamycin (0.05 mg/mL), and amphothericin B (0.0025 mg/mL). The epithelial layer was detached mechanically and the sub-epithelial specimens were plated in tissue culture flasks with Dulbecco’s Modification of Eagles’ Medium (DMEM), supplemented with 50% fetal calf serum (FCS), l -glutamine (2 mM), sodium pyruvate (1 mM), penicillin (50 UI/mL) and streptomycin (50 μg/mL), at 37 °C in a 5% humidified CO 2 atmosphere. After the first passage, the gingival fibroblasts were routinely cultured in DMEM supplemented with 10% FCS and were not used beyond the fifth passage .
2.6
Treatment of human primary fibroblasts with methacrylates and detection of the formed adducts
Cells were plated in 6-wells plates at a density of approximately 25,000 cells/cm 2 and cultured to subconfluent monolayer; HEMA (4 mmol/L) or TEGDMA (2 mmol/L) in PBS were then added and the cells were incubated for 1 h at 37 °C. Cell monolayers without monomers were used as controls. After incubation, the cells were lysed by freezing, and proteins were eliminated by precipitation with metaphosphoric acid (5% m/vol) and following centrifugation at 14,500 g (10 min, 4 °C); the samples were then analyzed by MEKC. The extracellular media (filtered by a 45 μm millipore filter) were also analyzed by MEKC.
2.7
Molecular modeling
All calculations were performed on a PC cluster using AMD 64 bit CPUs and running the Debian Linux operating system.
2.7.1
GST structure preparation
Two three-dimensional (3-D) GST co-crystallized with etacrinic acid (EAA) in two different bound conformations were retrieved from the PDB (http://www.pdb.org/) archive (pdb entry codes: 11gs and 3gss , Fig. 11 ). The co-crystals containing the etacrinic acid compounds were chosen for the structural similarities with the molecules under studies, i.e. TEGDMA and HEMA. The structures were refined by AMBER 8.0 program using the following protocol. AM1-BCC charges were calculated on the glutathione/etacrinic acid adduct employing the Amber antechamber module. Using the xLeap Amber module, the starting complexes were added of the hydrogen atoms and solvated in a octahedral box of TIP3P water with each box side at least 10.0 Å away from the nearest atoms of the complexes. Proper ions were included to neutralize the charge of the system. The ions were placed randomly in the system 10 Å away from the nearest atoms. The hydrogen atoms, counter ions and water molecules were then minimized for 1000 iterations. Then the whole complexes were relaxed for 5000 iterations. The Amber-all-atom (parm99) and the GAFF force fields were used in all calculations.
2.7.2
Docking procedure
The docking studies were performed using Autodock 4.0 . The two GTS complexes were aligned by means of UCSF Chimera MatchMaker tool . The molecule structures of TEGDMA and HEMA were built using the cdk libraries through a java implementation available on the RCMD web-site (http://www.rcmd.it). The Autodock graphical interface AutoDock Tools package 1.5.0 was employed to generate the docking input files and to analyze the docking results; the same procedure as described in the manual was followed. A grid box size of 50 × 50 × 50 points spacing of 0.375 Å were used, centered to the bound etacrinic acids and covered most of the catalytic pocket of both enzymes. For all the inhibitors, the single bonds excluding the amide bonds were treated as active torsional bonds. One hundred structures, i.e. 100 runs, were generated using Lamarckian genetic algorithm. A default protocol was applied, with an initial population of 150 randomly placed individuals, a maximum number of 2.5 × 10 6 energy evaluations, and a maximum number of 2.7 × 10 4 generations. A mutation rate of 0.02 and a crossover rate of 0.8 were used. The 100 poses obtained in each GSH were joined and clustered with a root mean square deviation (RMSD) value of 2.0. To validate the docking method, a random EAA conformation was generated and docked into either 11gs or 3gss proteins deprived of the co-crystallized EAA. Autodock proved to reposition the modeled EAA with RMSD errors lower than 2.0 (data not shown).
2
Materials and methods
All chemicals and reagents were supplied by Sigma–Aldrich Srl (Milan, Italy) unless otherwise indicated.
HPLC grade methanol was purchased from J.T. Baker (Baker Mallinckrodt, Phillipsburg, NJ, USA). HPLC grade water (18.2 mΩ) was prepared using a Millipore Milli-Q purification system (Millipore Corp., Bedford, MA, USA).
2.1
Synthesis of glutathione-methacrylate adducts
A solution of GSH (0.8 mmol/L) in phosphate buffer solution (PBS, pH 7.4, 1.0 mL) − bubbled with nitrogen to remove oxygen and inhibit GSH oxidation − was mixed at 37 °C with an equal volume of HEMA (1.0 mmol/L) or TEGDMA (0.4 mmol/L) in PBS ; GSH solution in PBS alone was similarly prepared. The reaction mixtures were analyzed immediately and after 48 h by HPLC–MS and MEKC; likewise GSH, HEMA and TEGDMA in PBS solutions were analyzed as controls.
2.2
Detection of GSH-methacrylate adducts by HPLC–MS and HPLC–UV
GSH-methacrylate reaction mixtures were analyzed by a SURVEYOR MS micro HPLC, (Thermo Finnigan, San Josè, CA, USA) using a Discovery HS C18 column (250 mm × 4.6 mm, 5 μm) (SUPELCO, Supelco Park, Bellefonte, PA, USA) at a flow rate of 1 mL/min. Water (A) and methanol (B) were used to obtain the chromatographic gradients for the elution: (i) for GSH-TEGDMA reaction mixture, from 5% (B) to 65% (B) in 32 min and then to 95% (B) in 8 min, (ii) for GSH-HEMA reaction mixture, from 25% (B) to 65% (B) in 23 min and then 95% (B) in 7 min followed by 10 min of maintenance. In both cases the column effluent was splitted by a “T junction” positioned after the chromatographic column sharing it between an a UV detector set at 214 nm (80%) and an ESI/MS in positive ion mode (20%) using a Finnigan LCQ DECA XP Max ion trap mass spectrometer equipped with Xcalibur® system manager data acquisition software (Thermo Finnigan, San Josè, CA, USA). The capillary voltage was set at 28 V, the spray voltage was set at 4.8 kV and the tube lens offset was set at 15 V. The capillary temperature was 250 °C. Data were acquired in MS1 scanning mode and recorded in the 50–1500 m / z region.
Total ion current (TIC) profile was produced by monitoring, during the chromatographic run, the intensity of all the ions produced and acquired in every scan.
2.3
Detection of GSH-methacrylate adducts by MEKC
To obtain the electrophoretic profiles of HEMA, TEGDMA, GSH and reaction mixtures, a capillary electrophoresis system (P/ACE™ MDQ, Beckman, Richmond, CA USA) with a fused silica capillary (60 cm length, 50 μm inner , Beckman) was used; a 0.2 mm detection window was obtained by a small flame at 10 cm from the cathodic terminal. The running solution was constituted by sodium phosphate/sodium tetraborate buffer (44.4 and 11.1 mmol/L, respectively), pH 7.19, with 36.7 mmol/L SDS as micellar phase and a final content of acetonitrile (10%, v/v). Samples were diluted (1:1) with a solution of sodium phosphate (7.2 mmol/L) and sodium tetraborate (1.8 mmol/L) at pH 7.19 and injected by pressure (0.5 psi) for 10 s at the anodic end of the capillary. After every run the capillary was washed (20 psi) with H 2 O, H 2 O/acetonitrile 1:1, H 2 O, NaOH and finally H 2 O. Each analysis was performed at a constant voltage of 18 kV with a current of nearly 50 μA at a temperature of 35 °C and at a wavelength of 214 nm. The adducts previously isolated and identified by HPLC–MS were also analyzed in the same operative conditions.
2.4
Treatment of red blood cells with methacrylates and detection of the formed adducts
Red blood cell suspensions (1.0 mL, 5% final hematocrit) were incubated in a shaking water bath for 1 h at 37 °C with HEMA (4 mmol/L) or TEGDMA (2 mmol/L) in PBS. As controls, red blood cell suspensions (1.0 mL, 5% hematocrit) in absence of monomers were used. After incubation, the samples were centrifuged (500 × g , 5 min), the precipitated cells were lysed by freezing and proteins were eliminated by precipitation with metaphosphoric acid (5% m/v) and following centrifugation at 15000 × g (10′, 4 °C); the samples were then analyzed by MEKC. The diluted extracellular media (filtered by a 45 μm millipore filter) were also analyzed by MEKC.
2.5
Cell culture of human gingival fibroblasts
Human gingival fibroblasts were obtained (with informed consent) from a healthy patient subjected to gingivectomy of the molar region. Immediately after removal, the tissues were placed in Hanks’ Balanced Salt Solution (HBSS) with penicillin (250 U/mL), streptomycin (0.25 mg/mL), gentamycin (0.05 mg/mL), and amphothericin B (0.0025 mg/mL). The epithelial layer was detached mechanically and the sub-epithelial specimens were plated in tissue culture flasks with Dulbecco’s Modification of Eagles’ Medium (DMEM), supplemented with 50% fetal calf serum (FCS), l -glutamine (2 mM), sodium pyruvate (1 mM), penicillin (50 UI/mL) and streptomycin (50 μg/mL), at 37 °C in a 5% humidified CO 2 atmosphere. After the first passage, the gingival fibroblasts were routinely cultured in DMEM supplemented with 10% FCS and were not used beyond the fifth passage .
2.6
Treatment of human primary fibroblasts with methacrylates and detection of the formed adducts
Cells were plated in 6-wells plates at a density of approximately 25,000 cells/cm 2 and cultured to subconfluent monolayer; HEMA (4 mmol/L) or TEGDMA (2 mmol/L) in PBS were then added and the cells were incubated for 1 h at 37 °C. Cell monolayers without monomers were used as controls. After incubation, the cells were lysed by freezing, and proteins were eliminated by precipitation with metaphosphoric acid (5% m/vol) and following centrifugation at 14,500 g (10 min, 4 °C); the samples were then analyzed by MEKC. The extracellular media (filtered by a 45 μm millipore filter) were also analyzed by MEKC.
2.7
Molecular modeling
All calculations were performed on a PC cluster using AMD 64 bit CPUs and running the Debian Linux operating system.
2.7.1
GST structure preparation
Two three-dimensional (3-D) GST co-crystallized with etacrinic acid (EAA) in two different bound conformations were retrieved from the PDB (http://www.pdb.org/) archive (pdb entry codes: 11gs and 3gss , Fig. 11 ). The co-crystals containing the etacrinic acid compounds were chosen for the structural similarities with the molecules under studies, i.e. TEGDMA and HEMA. The structures were refined by AMBER 8.0 program using the following protocol. AM1-BCC charges were calculated on the glutathione/etacrinic acid adduct employing the Amber antechamber module. Using the xLeap Amber module, the starting complexes were added of the hydrogen atoms and solvated in a octahedral box of TIP3P water with each box side at least 10.0 Å away from the nearest atoms of the complexes. Proper ions were included to neutralize the charge of the system. The ions were placed randomly in the system 10 Å away from the nearest atoms. The hydrogen atoms, counter ions and water molecules were then minimized for 1000 iterations. Then the whole complexes were relaxed for 5000 iterations. The Amber-all-atom (parm99) and the GAFF force fields were used in all calculations.
2.7.2
Docking procedure
The docking studies were performed using Autodock 4.0 . The two GTS complexes were aligned by means of UCSF Chimera MatchMaker tool . The molecule structures of TEGDMA and HEMA were built using the cdk libraries through a java implementation available on the RCMD web-site (http://www.rcmd.it). The Autodock graphical interface AutoDock Tools package 1.5.0 was employed to generate the docking input files and to analyze the docking results; the same procedure as described in the manual was followed. A grid box size of 50 × 50 × 50 points spacing of 0.375 Å were used, centered to the bound etacrinic acids and covered most of the catalytic pocket of both enzymes. For all the inhibitors, the single bonds excluding the amide bonds were treated as active torsional bonds. One hundred structures, i.e. 100 runs, were generated using Lamarckian genetic algorithm. A default protocol was applied, with an initial population of 150 randomly placed individuals, a maximum number of 2.5 × 10 6 energy evaluations, and a maximum number of 2.7 × 10 4 generations. A mutation rate of 0.02 and a crossover rate of 0.8 were used. The 100 poses obtained in each GSH were joined and clustered with a root mean square deviation (RMSD) value of 2.0. To validate the docking method, a random EAA conformation was generated and docked into either 11gs or 3gss proteins deprived of the co-crystallized EAA. Autodock proved to reposition the modeled EAA with RMSD errors lower than 2.0 (data not shown).
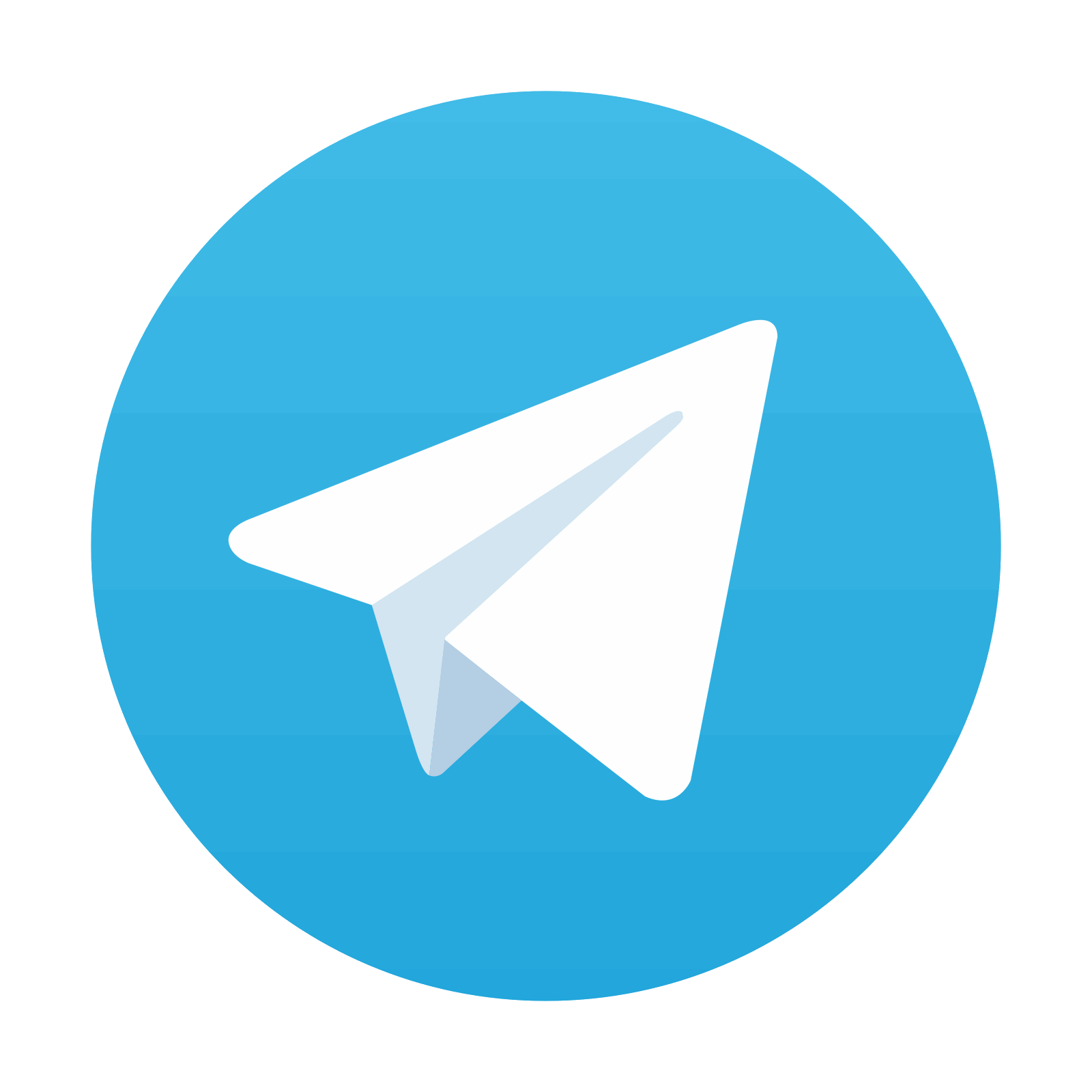
Stay updated, free dental videos. Join our Telegram channel

VIDEdental - Online dental courses
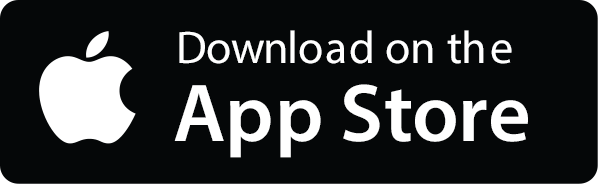
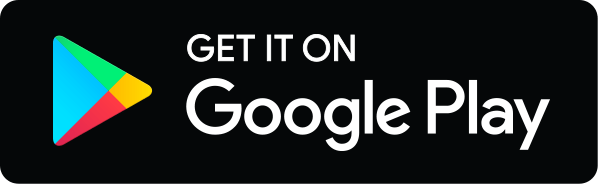