Abstract
New and more consistent biomarkers of oral squamous cell carcinoma (OSCC) are needed to improve early detection of the disease and to monitor patient management. The aim of this study was to detect new OSCC tumor markers in saliva. Unstimulated saliva, collected from patients with primary stage I OSCC as matched pre-and post-treatment samples, was used in the analysis. A surface-enhanced laser desorption/ionization time-of-flight mass spectrometry (SELDI-TOF) ProteinChip system was used to screen for differentially expressed proteins in the saliva samples. This analysis revealed 26 proteins with significantly different expression levels in the pre-and post-treatment samples (P < 0.05). A 14 kDa protein detected in pre-treatment saliva from the OSCC patients was identified as a truncated cystatin SA-I, with deletion of three amino acids from the N-terminus. The authors propose that ProteinChip analysis may provide a reliable screening test for early diagnosis of OSCC and that truncated cystatin SA-I might be a useful tumor biomarker for OSCC.
There are often few signs and symptoms of early-stage cancer and most patients present with advanced disease, associated with increased treatment-related morbidity and poorer survival rates. Early detection is the most effective means of reducing death from cancer. The importance of prevention and early diagnosis are well established for oral squamous cell carcinoma (OSCC), but there is no accepted screening test for this cancer. Head and neck SCC (HNSCC) is not mentioned in the most recent screening guidelines of the American Cancer Society , presumably because of the lack of available screening tools. A complete head and neck examination and imaging studies in patients with suspicious clinical findings or symptoms are the only current approaches, and the invasive nature of a biopsy makes it unsuitable for cancer screening in high-risk populations.
There is a need for new diagnostic tools for early detection. A molecular marker in a body fluid that predicts the development of OSCC at an early or precancerous stage would constitute such a tool. The ongoing search for biomarkers of OSCC has focused primarily on detection of genetic abnormalities leading to development of HNSCC . Molecular techniques for this purpose require significant time, labor and expertise. Efforts at detecting biomarkers associated with OSCC have revealed considerable heterogeneity in biomarker expression, and no single biomarker or combination of biomarkers has been found to be effective as a diagnostic or screening tool. The search for diagnostic biomarkers has switched to deciphering the OSCC proteome. Proteomic analysis has become increasingly important for identifying changes in protein expression associated with malignancy, traditionally through the use of two-dimensional gel electrophoresis (2D-PAGE) to detect differences in protein expression between healthy tissues or body fluid and disease samples . 2D-PAGE has limitations, particularly for detecting proteins of low abundance and molecular weight less than 10 kDa, it is labor intensive, has low throughput, and is not easily applied in the clinical setting.
There have been many recent technological advances in proteomics, including development of ProteinChip surface-enhanced laser desorption/ionization time-of-flight mass spectrometry (SELDI-TOF-MS) . This method is rapid, has high throughput capability and high sensitivity, and allows resolution of small amounts of proteins .
This technology has potential for use as a clinical assay for early diagnosis of human cancers , including detection of HNSCC-associated serum tumor biomarkers . Cancer-related nucleic acids in blood, urine and cerebrospinal fluid have been used as biomarkers for cancer diagnosis and use of saliva as a diagnostic fluid meets the demand for an inexpensive, noninvasive, and accessible diagnostic methodology . The authors tested the hypothesis that distinct protein expression patterns can be identified in saliva from cancer patients, and that the differentially expressed proteins can serve as biomarkers for detection of oral cancer. In the present study, the authors used SELDI-TOF-MS to identify a truncated cystatin SA-I as a candidate salivary tumor biomarker for OSCC.
Patients and methods
Patient selection
16 patients (10 males, 6 females; aged 56.3 ± 5.6 years) with stage I OSCC were included in the study. The primary sites were the tongue in 10 cases, the lower gingiva in 4, and the buccal mucosa in 2. All the patients had received recent diagnoses of the primary disease and had been treated with surgery alone. None had a history of malignancy, immunodeficiency, autoimmune disorders, hepatitis or HIV infection. All patients signed the institutional review board-approved consent form and agreed to serve as saliva donors.
Saliva collection
Unstimulated saliva samples were collected between 9 and 10 a.m. using previously established protocols . The patients were asked to refrain from eating, drinking, smoking or oral hygiene procedures for at least 1 h before collection. Saliva samples were centrifuged at 2600 g for 15 min at 4 °C. The supernatant was removed from the pellet, immediately aliquoted and frozen for storage at -80 °C until thawed for mass spectrometry analysis.
SELDI protein profiling
Expression difference mapping analysis profiles of the samples were obtained using anion-exchange (Q10), cation-exchange (CM10), reversed phase (H50), and immobilized affinity capture (IMAC3) ProteinChip arrays (Ciphergen Biosystems, Fremont, CA, USA). The ProteinChip arrays were assembled in a deep-well type bioprocessor assembly (Ciphergen Biosystems). The ProteinChip arrays were analyzed using the ProteinChip biology system reader (Model PBS II, Ciphergen Biosystems) and the resulting data were analyzed by ProteinChip software version 3.0 (Ciphergen Biosystems). All data were normalized by the total ion current normalization function following the instructions in the software.
The following procedure was used for purification of a biomarker candidate (m/z 13954) identified from the ProteinChip arrays. A pretreatment saliva sample was centrifuged at 14,000 rpm for 10 min and diluted with 0.4 ml of 9 M urea buffer, pH 7, and further diluted with 1.2 ml of 50 mM Tris HCl buffer, pH 9. The diluted sample was loaded onto a Q-sepharose spin column and the supernatant was separated on a CM sepharose fast flow column (GE Healthcare, Amersham Place, Little Chalfont, Buckinghamshire HP7 9NA, England) equilibrated with 50 mM sodium acetate buffer, pH 4. The biomarker candidate was eluted and collected for further purification. A 0.4-ml aliquot of the partially purified fraction from the CM sepharose column was applied to a TSK-GEL super ODS column (TOSOH CORP. Tokyo, JAPAN). The biomarker candidate was eluted from the column with a linear gradient of acetonitrile from 31.5% to 40.5% in 40 min (2.0 ml), with collection of each 0.5-min fraction (0.022 ml). The purification process was monitored using Q10 and CM10 arrays for the Q-sepharose column and a NP20 array for the TSK-GEL super ODS column.
To identify the protein, the peptide digests from each spot were analyzed by the ProteinChip biology system (Ciphergen Biosystems) for peptide mass fingerprint analysis and by a QSTAR Pulsar I (ABI) system equipped with a PCI 1000 ProteinChip array interface (Ciphergen Biosystems) for sequence tag analysis. Database searches with Mascot and MS-tag were performed. In addition to identification by mass spectrometry, an N-terminal amino acid sequence analysis was performed to confirm the structure of the N-terminal region.
Results
Proteomics analysis of pre-and post-treatment saliva samples
In the working mass range of 2,000 to 200,000 m/z, approximately 200 peaks were resolved using the ionic bead-capture approach. ProteinChip analysis indicated 26 proteins that exhibited significantly different expression levels between pre-and post-treatment saliva samples (P < 0.05): 17 with decreased expression and 9 with increased expression after treatment. Protein peak clustering analysis was also able to distinguish between the pre-and post-treatment samples. There is no significant difference of any protein expression peak between sub-regional differences and gender.
Expression difference mapping analysis
Protein profiles of 36 saliva samples were obtained on CM10, Q10, IMAC3-Cu2+, and H50 ProteinChip arrays ( Fig. 1 ). These arrays have different surface properties that lead to retention of different groups of proteins. Processing of the lysate on each surface resolved between 150 and 200 protein peaks in the mass range of 3–100 kDa. Representative spectra of saliva on CM10 arrays are shown in Fig. 2 . Expression profiles among the samples revealed several differences in protein patterns and a 14 kDa protein was found that showed significantly higher expression in pre-treatment saliva compared with post-treatment saliva from OSCC patients.
Purification of the 14 kDa marker candidate
ProteinChip arrays can be used to develop a purification process because of their chemical properties as chromatographic sorbents. A saliva sample was diluted with 9 M urea buffer (pH 7) and 50 mM Tris buffer (pH 9) and applied to a Q-sepharose spin column. The fraction containing the target protein was eluted with 500 mM NaCl in a 50 mM Tris buffer (pH 9) fraction. The eluant was then applied to a CM sepharose fast flow spin column for further separation from contaminants. Elution was performed with a NaCl gradient from 0 to 500 mM and monitored by profiling on the ProteinChip biology system. The target protein was eluted in the 200 mM NaCl fraction, with the same results as those observed in the on-chip optimization analysis. The flow-through fractions of the CM sepharose fast flow spin column were subjected to SDS-PAGE and SELDI analysis for further separation ( Fig. 3 ). These analyses gave identical results for the 14 kDa protein in pre-treatment saliva and the SDS-PAGE band corresponding to the protein was collected for identification.
Identification of the biomarker candidate
The biomarker candidate protein was identified using chromatography and mass spectrometry, as described in Methods. Database searches with Mascot and MS-tag indicated that the amino-acid sequence of the protein ( Fig. 4 ) corresponded to cystatin SA-I with deletion of the three N-terminal amino acids. Sequence analysis confirmed the structure of the N-terminal region of the truncated cystatin ( Fig. 4 ).
Expression levels of truncated cystatin SA-I
To confirm that the 14 kDa truncated cystatin SA-I is an OSCC-specific protein, the expression levels were compared between pre-and post-treatment saliva from OSCC patients. Saliva profiles on CM10 arrays at pH 4 clearly showed a higher intensity for truncated cystatin SA-I in pre-treatment saliva samples ( Fig. 5 ).
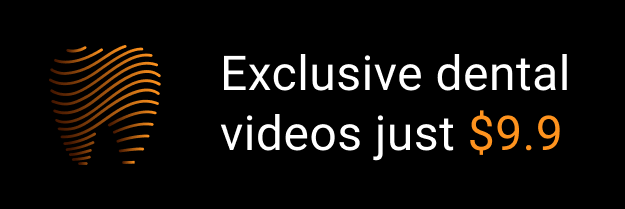