Highlights
- •
Bioceramic scaffold/hTDM constructs favored odontogenic differentiation and biomineralization.
- •
Scaffolds released Mg 2+ , Ca 2+ , Si 4+ and Zn 2+ ions and hTDMs growth factors (TGFβ-1, DMP-1 and BMP-2).
- •
Mineralized Ca-P tissue, partially transformed into bioapatite was generated in the constructs.
- •
Hr-DMP-1 and hr-BMP-2 further enhanced odontogenic differentiation phenomena.
Abstract
Objective
This study aimed to investigate the potential of Mg-based bioceramic scaffolds combined with human treated-dentin matrices (hTDMs) and dentinogenesis-related morphogens to promote odontogenic differentiation and dentin-like tissue formation by Dental Pulp Stem Cells-DPSCs.
Methods
DPSC cultures were established and characterized by flow cytometry. Experimental cavities were prepared inside crowns of extracted teeth and demineralized by EDTA (hTDMs). Zn-doped, Mg-based bioceramic scaffolds, synthesized by the sol–gel technique, were hosted inside the hTDMs. DPSCs were spotted inside the hTDMs/scaffold constructs with/without additional exposure to DMP-1 or BMP-2 (100 ng/ml, 24 h). Scanning Electron Microscopy-SEM, live/dead fluorescence staining and MTT assay were used to evaluate cell attachment and viability; Real time PCR for expression of osteo/odontogenic markers; Inductively Coupled Plasma-Atomic Emission Spectrometry-ICP/AES for scaffold elemental release analysis; ELISA for hTDM growth factor release analysis; SEM and X-ray Diffraction-XRD for structural/chemical characterization of the regenerated tissues.
Results
Scaffolds constantly released low concentrations of Mg 2+ , Ca 2+ , Zn 2+ and Si 4+ , while hTDMs growth factors, like DMP-1, BMP-2 and TGFβ-1. hTDMs/scaffold constructs supported DPSC viability, inducing their rapid odontogenic shift, indicated by upregulation of DSPP, BMP-2, osteocalcin and osterix expression. Newly-formed Ca-P tissue overspread the scaffolds partially transforming into bioapatite. Exposure to DMP-1 or BMP-2 pronouncedly enhanced odontogenic differentiation phenomena.
Significance
This is the first study to validate that combining the bioactivity and ion releasing properties of bioceramic materials with growth factor release by treated natural dentin further supported by exogenous addition of key dentinogenesis-related morphogens (DMP-1, BMP-2) can be a promising strategy for targeted dentin regeneration.
1
Introduction
Several previous studies have provided evidence that dental restorative procedures are closely linked to a number of biological and technical complications, or adverse reactions, leading to unpredictable therapeutic efficacy . This provides exemplary justification for identifying regeneration of dentin, the bulk of tooth structure, as the “ultimate goal” of current tissue engineering strategies as an alternative to simply “filling the gaps”. In recent years, regenerative dentistry has made huge steps towards the regeneration of the dentin-pulp complex or even fully functional teeth in animal models .
In this context, the choice of suitable scaffold materials, providing the three-dimensional artificial matrix mimicking the extracellular microenvironment is crucially important. Many synthetic and natural scaffold materials have already been employed in dentin regeneration protocols, including long-lasting porous bioceramics (e.g. hydroxyapatite, β-tricalcium phosphate, bioactive glasses etc.), natural molecules of intermediate duration (e.g. collagen and chitosan) and short-lasting polymers, such as polyglycolic acid (PGA), polylactic acid (PLA), or their combinations . Each material offers unique chemical properties, composition, structure, degradation profile and possibilities for modification . However, few have proved able to regenerate the complete dentin tissue. Therefore, there is still a vacant role for the ideal scaffold for targeted dentin regeneration.
Bioceramic scaffolds have shown several advantages over other materials, such as bioactivity (i.e. apatite-forming ability) and biodegradability, suitable porous structure and ability to support osteo/odontogenic differentiation and biomineralization . Tailoring ionic dissolution (= biointeractivity) from bioceramic materials is key to achieving specific biological responses, since many elements released by these materials, such as Si 4+ , P, Zn 2+ , Mg 2+ , or Ca 2+ ions, participate in signaling pathways and intracellular interactions in the human body . In particular, Mg has been shown to influence biomineralization and apatite crystal formation in bone and teeth , while Zn has been reported to affect bioactivity, biocompatibility and antimicrobial properties when incorporated into the structure of various glasses . However, use of bioceramic materials alone has been limited by poor mechanical strength .
In the present study, Zn-doped, Mg-based bioceramic scaffolds, tailored for controlled release of low concentrations of Mg 2+ , Ca 2+ , Si 4+ and Zn 2+ ions, previously characterized for their bioactivity, degradation rate, porosity and ability to support attachment and proliferation of Dental Pulp Stem Cells-DPSCs , were studied for their potential to induce odontogenic differentiation and dentin-like tissue formation when seeded with DPSCs and placed in experimental cavities of human treated dentin matrices (hTDMs) . hTDMs have been proposed as suitable biologic scaffolds, due to the combination of good mechanical properties and ability to act as a reservoir of dentinogenesis-related growth/morphogenetic factors . To our knowledge, this is the first study combining the bioactive properties of Mg-based bioceramic materials with the mechanical strength and growth factor release by hTDMs in an in vitro hybrid experimental model aiming to simulate the clinical situation.
Therefore, this study aimed to investigate the potential of Mg-based, Zn-doped bioceramic scaffolds, combined with hTDMs, to act as suitable hybrid scaffolding materials to induce odontogenic differentiation of DPSCs and regeneration of new mineralized dentin matrix. It was hypothesized that combining the properties of both types of scaffolds might provide the ideal microenvironment for targeted dentin regeneration. The second research hypothesis was that additional exogenous application of key dentinogenesis-related morphogens, such as DMP-1 and BMP-2, would further enhance odontogenic differentiation and biomineralization phenomena. The results of this study are expected to support growing evidence on the use of bioceramic materials combined with dentin pre-conditioning protocols for targeted dentin regeneration.
2
Materials and methods
2.1
Establishment of DPSC cultures
DPSC cultures were established from third molars of young healthy donors using the enzymatic dissociation method, as previously described . Samples were collected according to the guidelines of the Institutional Review Board and donors signed an informed consent form. Briefly, the teeth were disinfected and cut around the cementum-enamel junction to expose the pulp chamber. The pulp tissue was minced into small fragments and digested in a solution of 3 mg/ml collagenase type I and 4 mg/ml dispase II (Invitrogen, Karlsruhe, Germany) for 1 h at 37 °C. Single cell suspensions were obtained by passing the cells through a 70 μm cell strainer (BD Biosciences, Heidelberg, Germany). The cells were then expanded with a-MEM (Minimum Essential Media) culture medium (Invitrogen), supplemented with 15% FBS (EU-tested, Invitrogen), 100 mM l -ascorbic acid phosphate (Sigma–Aldrich, Taufkirchen, Germany), 100 units/ml penicillin, 100 mg/ml streptomycin and 0.25 mg/ml Amphotericin B (all from Invitrogen) (=Complete Culture Medium – CCM) and incubated at 37 °C in 5% CO 2 . Cultured DPSCs in passage numbers from 2 to 8 from at least two donors were used for all experiments with similar results.
2.2
Characterization of DPSC cultures with flow cytometry
DPSCs were extensively characterized by flow cytometry for several mesenchymal (CD90/Thy-1, CD73, CD29/b1-integrin, CD49f/a6-integrin, CD81-TAPA, CD166/ALCAM, CD146/MUC18, STRO-1, CD34), neural (CD271/NGFR, nestin), endothelial (CD105/endoglin, CD106/VCAM), hematopoietic (CD117/c-Kit, CD14, CD45) and embryonic (Nanog, Oct3/4, TRA-1-60, TRA-1-81, SSEA-1, 3, -4, -5) stem cell (SC) markers, as previously described . Briefly, cells were trypsinized, washed with PBS and then stained with the following fluorochrome-conjugated mouse anti-human antibodies: CD90-FITC (fluorescein isothiocyanate) CD73-PE (phycoerythrin), CD29-APC (allophycocyanin), CD49f-APC, CD81-FITC, CD166-PE, CD146-PE, STRO-1-FITC, CD34-APC, CD271-PE, CD105-FITC, CD106-APC, c-kit/CD117-PerCP-Cy5.5 (Peridinin-Chlorophyll-Protein Complex), CD14-FITC, CD45-PE, TRA-1-60-PE, TRA-1-81-APC, SSEA-1-PE, SSEA-3-PE, SSEA-4-FITC, SSEA-5-APC (all from BioLegend, Fell, Germany). For intracellular staining for Nanog, Oct3/4 and nestin, the cells were first fixed with a 4% paraformaldehyde buffer, permeabilized with a 0.1% saponin buffer (both from BD Biosciences) and then stained with the mouse anti-human antibodies Oct3/4-Alexa Fluor 647, Nanog-PE (both from BD Biosciences) and nestin- APC (RnD Systems, Minneapolis, USA). The stained cells were washed twice with staining buffer (PBS + 1% BSA + 0.1% NaN3) and analyzed using a BD LSR II Flow Cytometer. A total of 100,000 events were acquired for each sample. Data were analyzed using Summit software, version 5.1 for Windows (Beckman Coulter, Inc. Krefeld, Germany).
2.3
Preparation of 3D bioceramic scaffolds
Ceramic scaffolds were synthesized with the foam replica technique using a sol–gel derived glass composition in the system (SiO 2 = 60, MgO = 7.5, CaO = 30, ZnO = 2.5 in wt%) . The sol–gel solution was prepared as described by Theodorou et al. by the hydrolysis of tetraorthosilicate (TEOS) and the addition of calcium nitrate tetrahydrate (Ca(NO 3 ) 2 ·4H 2 O), magnesium nitrate hexahydrate (Mg(NO 3 ) 2 ·6H 2 O) and zinc nitrate hexahydrate (Zn(NO 3 ) 2 ·6H 2 O) (all from Sigma–Aldrich) into the mixture. Polyurethane (PU) foam was cut into pieces 10 mm × 10 mm × 5 mm and immersed in the sol–gel solution described above. After immersing the foam in the sol–gel and mechanical stirring for 5 min, the samples (green bodies) were retrieved and squeezed to remove the excess of sol from the pores and then left to dry out for at least 12 h. The thickness of the bioactive glass on the green bodies was adjusted using droplets of sol–gel and the excess removed after centrifuging the green bodies. Next, the synthesized scaffolds were sintered at 890 °C (10 °C/min) with 2 h annealing, as previously described . The fabricated scaffolds presented 84% porosity and pore interconnectivity, a mean compressive strength of 0.10 (±0.06) MPa and controlled degradation rate of 3.5% after 120 h immersion in Tris Buffer solution used for the simulation test and 5% in citric acid solution used for the extreme test .
2.4
Preparation and characterization of human treated dentin matrices (hTDMs)
2.4.1
Preparation of hTDMs
hTDMs were prepared using crowns of impacted third molars of young healthy donors obtained after routine extraction. The extracted teeth were preserved in PBS containing 2x antibiotics/antimycotics, in order to reduce their microbial load and maintain their moisture level. Periodontal tissues were scraped away and the coronal part of the tooth separated from the root by a transverse section at the cemento-enamel junction. Dental pulp tissues and part of the pre-dentin were also removed by mechanical means. Experimental cavities (approx. 4 mm × 4 mm × 2.5 mm) were then prepared inside the tooth crowns to host the scaffolds ( Fig. 1 ). Cavity preparation was followed by a multi-step soaking procedure to achieve dentin demineralization, as already described by Li et al. . In detail, the prepared crowns were immersed in deionized water (dH 2 O) for 20 min in an ultrasonic cleaner followed by 40 min immersion in dH 2 O without sonication. Then dH 2 O was changed and the same procedure repeated a further 4 times for a total of 5 h. The crowns were then treated (hTDM) with descending concentrations of the chelating agent Ethylene Diamine Tetraacetic Acid – EDTA (Sigma–Aldrich), as follows: 17% EDTA followed by 10% and 5% treatment, each for 5 min. After each EDTA treatment step, a washing step with dH 2 O for 2 min in an ultrasonic cleaner was performed. The hTDMs were then stored in sterile PBS, supplemented with antibiotics/antimycotics for 24 h and then disinfected by UV irradiation for 30 min before being used for further experiments.
2.4.2
Evaluation of growth factor release from hTDMs
To verify the beneficial effect of EDTA treatment in growth factor release from the hTDMs, enzyme linked immunosorbent assays (ELISA) were used. The prepared crown specimens were placed in 24 well-plates and fully covered with 1 ml of a-MEM medium, supplemented with 1% FBS to avoid protein adsorption on plastic. The culture medium was collected at regular time-points after 3, 6, 9, 12 and 15 days (d), centrifuged at 200 × g for 5 min to remove any debris and the samples stored at −80 °C until used for further experiments. All samples were screened for the presence of growth factors, including BMP-2, DMP-1 and TGFβ-1. Sandwich colorimetric ELISA kits were obtained from R&D Systems and assays performed following the manufacturer’s instructions. Baseline values for BMP-2, DMP-1 and TGFβ-1 contained in culture medium were subtracted from the values measured in the collected samples.
2.4.3
Morphological observation of hTDMs by Scanning Electron Microscopy-SEM
To investigate whether EDTA treatment was effective in thoroughly exposing the dentinal tubules and collagen bundles for growth factor release, hTDMs were observed by SEM. Briefly, hTDMs were washed in PBS, fixed with 3% glutaraldehyde in 0.1 M sodium cacodylate containing 0.1 M sucrose, at pH 7.4, dehydrated using a graded series of ethanol concentrations and remained under air-drying in the hood for 20–30 min before finally being carbon coated and observed under SEM (JEOL J.S.M. 840A, Tokyo, Japan).
2.5
Analysis of ions released from the bioceramic scaffolds using inductively coupled plasma-atomic emission spectrometry – ICP/AES
Scaffolds were placed in 24 well-plates and fully covered with 1 ml of a-MEM culture medium, supplemented with antibiotics/antimycotics without any serum. The medium was collected at regular time-points after 3, 6, 9, 12 and 15 d. After each collection point the medium was renewed and re-collected at the next time-point, to assess the time-course elemental release into the culture medium from the bioceramic scaffolds. The collected samples were centrifuged at 200 × g for 5 min to remove any scaffold debris and stored at −80 °C until used for the ICP-AES. The samples were analyzed for silica (Si 4+ ) magnesium (Mg 2+ ), calcium (Ca 2+ ), phosphorous (P) and zinc (Zn 2+ ). As control, the culture medium used for the elution was also analyzed to detect the baseline concentration values of these ions, which were finally subtracted from those detected in the scaffold eluates.
For the analysis a Perkin Elmer (USA) model 3100 XL Axial-Viewing Spectrometer was used. The plasma atomizer was configured to include a cross-flow nebulizer attached to a Scott-type spray chamber for nebulization, while the nebulizer gas flow rate was adjusted to 0.8 L min −1 . As plasma gas high purity argon (99.995%) was used and the radiofrequency power was adjusted to 1350 W. The spectrometer was equipped with a solid-state segmented array charge-coupled device (SCD) as a detector. The whole measurement cycle was 75 s, taking 7 points per analyte peak. The following wavelengths were used respectively: Si 251.611 nm; Ca 396.847 nm; P 213.617 nm; Zn 213.857 nm; Mg 280.271 nm. All solutions were prepared using analytical grade reagents supplied by Merck (Darmstadt, Germany) and ultrapure water of Milli-Q quality (18.2 MΩ, Millipore, Bedford USA). Quantitative determinations were based on linear calibration curves obtained from multi-element working standards containing the analytes in the range 2 × 10 −2 –2 × 10 1 mg/L. These working standard solutions of the analytes (Si, Ca, Mg, Zn, P) were prepared by appropriate stepwise dilutions of stock standard solutions containing 1000 mg l −1 of each analyte. A suitable portion of each sample solution was transferred into 50 mL or 100 mL volumetric flask and diluted to the mark with double ionized water. The final results were expressed as mg/L (ppm).
2.6
DPSCs seeding into the hTDMs/scaffold constructs and analysis of cell viability and osteo/odontogenic differentiation potential
The scaffolds were adjusted into the prepared cavities in the hTDMs by minor trimming of their edges to achieve direct contact with the dentinal cavity walls ( Fig. 1 ). The hTDMs/scaffold constructs were first placed into each well of 24-well plates and incubated with CCM for 2 h at 37 °C and 5% CO 2 , to mimic the effect of tissue fluids, allowing initial wetting of the scaffolds with the serum containing medium, optimizing the conditions for protein absorption and therefore initial cell attachment, as previously supported . Each scaffold was then spotted with 100 μl CCM containing 10 6 DPSCs. The spotted scaffolds were incubated for 2 h at 37 °C and 5% CO 2 to allow initial cell attachment and then fully covered with 1.2 ml CCM/well. Medium change was performed every 2 d. In parallel experiments, DPSCs were either seeded in 2D culture 6-well plates at 3 × 10 5 cells/well, or spotted in scaffolds (SC) placed directly inside 24-well plates without the presence of hTDMs. These parallel (control) experiments were designed to to allow separate evaluation of each factor of the constructs (i.e. CELLS, SC and hTDMs) on the odontogenic differentiation of DPSCs. In a second set of experiments, CELLS, SC/CELLS or hTDMs/SC/CELL constructs were first exposed to 100 ng/ml human recombinant hr-BMP-2 (Invitrogen) or hr-DMP-1 (R&D Systems) for 24 h before being exposed to CCM for the rest of the observation period. The purpose of this procedure was to assess whether external application of these growth/morphogenetic factors would enhance or accelerate the odontogenic shift of DPSCs inside the constructs.
After 3, 7 and 14 days (d), live/dead fluorescent staining (Calcein M/PI staining) was applied to evaluate cell viability inside the constructs using confocal microscopy, while cell morphology/attachment inside the scaffolds was evaluated by SEM. MTT assay was additionally used to evaluate cell viability/proliferation after 3, 6, 9, 12 and 15 by means of a metabolic-based test. Total RNA was isolated after 7 and 14 d and Real time PCR was performed to evaluate expression of several osteo/odontogenic differentiation markers, including Dentin Sialophosphoprotein (DSPP), Bone Morphogenetic Protein (BMP-2), Alkaline Phosphatase (ALP), Ostecalcin (Bone gamma-carboxyglutamic acid-containing protein- BGLAP), Runt- related transcription factor 2 (Runx2) and Osterix. Finally, protein lysates were collected from the constructs after 3, 7 and 14 d to evaluate ALP enzymatic activity.
2.6.1
Evaluation of cell viability by live/dead fluorescence staining
The constructs were double stained with Calcein AM and Ethidium Homodimer-EthD1 (live/dead respectively) staining. Stained complexes were observed under a confocal microscope (Leica Microsystems, Wetzlar, Germany). Approximately 20 depth dependent serial sections were taken and the projection images were produced. Quantification of the percentage of live and dead cells was performed by pixel analysis using the Image J color pixel counter plugin. hTDMs/SC/CELL complexes were also examined at the same time-points (3, 7 and 14 d) in higher magnifications regarding cell morphology and attachment by SEM, as described in Section 2.4.3 .
2.6.2
Evaluation of cell viability/proliferation by MTT assay
Cell viability/proliferation was determined using the MTT [3-(4, 5-dimethylthiazol-2-yl)-2,5-diphenyltetrazolium bromide] assay. The hTDMs/SC constructs were spotted with 2.5 × 10 5 cells/construct, as described in paragraph 2.6. After 3, 6, 9, 12 and 15 d, 50 μl of MTT (5 mg/ml in PBS) were added in each well hosting the hTDMs/SC/CELL complexes, which were incubated for 4 h at 37 °C and 5% CO2. After this period, the medium containing the MTT solution was discarded, the complexes were washed with PBS and the insoluble formazan was dissolved with DMSO overnight at room temperature (RT). The absorbance was measured against blank (DMSO) at a wavelength of 545 nm and a reference filter of 630 nm by a microplate reader (Epock, Biotek, Biotek instruments, Inc, Vermont, USA). As controls, scaffolds without cells were incubated under the same conditions and the optical density values were subtracted from values obtained by the corresponding hTDMs/SC/CELL complexes.
2.6.3
Quantitative real-time reverse-transcription polymerase chain reaction analysis
Total mRNA was isolated from each of the CELLS, SC/CELL and hTDMs/SC/CELL constructs using a Nucleospin RNA isolation kit (Macherey Nagel, Düren, Germany) and reverse transcribed (1 μg/sample) using superscript first-strand synthesis kit (Invitrogen), according to manufacturer’s instructions. Reactions were performed using SYBR-Select PCR Master Mix (Applied Biosystems, Foster City, CA) in a Step One Plus thermal cycler (Applied Biosystems). All reactions started with two initial incubation steps at 50 °C for 2 min and at 95 °C for 2 min and were followed by 40 cycles of PCR, comprising denaturation for 15 s at 95 °C and annealing/extension for 1 min at 60 °C. Primers were designed using the Primer-Blast software from the NCBI nucleotide sequence database ( ) for the following genes: DSPP, BMP-2, RUNX2, OSTERIX, ALP, BGLAP ( Table 1 ). The results were adjusted by amplification efficiency (LinRegPCR) and were normalized to the two most stable housekeeping genes evaluated by geNorm (succinate dehydrogenase complex, subunit A, flavoprotein-SDH-A and beta-2-microglobulin-B2M).
Gene symbol | Forward (5′–3′) | Reverse (5′–3′) | Amplicon size (bp) |
---|---|---|---|
DSPP | GCTGGCCTGGATAATTCCGA | CTCCTGGCCCTTGCTGTTAT | 135 |
BMP-2 | GGAACGGACATTCGGTCCTT | AGTCCGTCTAAGAAGCACGC | 100 |
ALP | CCGTGGCAACTCTATCTTTGG | CAGGCCCATTGCCATACAG | 89 |
RUNX2 | CCACCGAGACCAACAGAGTC | TCACTGTGCTGAAGAGGCTG | 118 |
OSTERIX (SP7) | ATCCAGCCCCCTTTACAAGC | TAGCATAGCCTGAGGTGGGT | 78 |
BGLAP | GACTGTGACGAGTTGGCTGA | AAGAGGAAAGAAGGGTGCCT | 137 |
B2 M | TGTCTTTCAGCAAGGACTGGT | ACATGTCTCGATCCCACTTAAC | 138 |
SDHA | GCATGCCAGGGAAGACTACA | GCCAACGTCCACATAGGACA | 127 |
2.6.4
Evaluation of ALP enzymatic activity
ALP activity was detected using a commercial kit (Abnova, Taipei, Taiwan) according to the manufacturer’s protocol. Briefly, the cell/scaffold constructs were homogenized in 100 ul of the lysis buffer supplied by the kit. The lysates were centrifuged at 13,000 × g for 3 min at 4 ∘C and the supernatant was quantified regarding protein content with a Bicinchoninic acid (BCA) assay kit (Sigma–Aldrich) according to the manufacturer’s instructions. Then, 5 μg of each protein sample were used for the ALP assay using p-nitrophenyl phosphate (pNPP) as a phosphatase substrate and the ALP supplied by the kit as a standard. The absorbance was measured at 405 nm by a microplate reader (Epock, Biotek, Biotek instruments, Inc, Vermont, USA) and the amount of ALP in the cells was normalized against total protein content.
2.7
Structural and chemical characterization of the mineralized tissue formed inside the hTDMs/SC/CELL constructs by means of scanning electron microcopy – energy dispersive X-ray spectroscopy (SEM-EDS) and X-ray diffraction analysis (XRD)
For this set of experiments DPSCs were seeded into the hTDMs/SC constructs, as described in 2.6 and exposed to CCM, additionally supplemented with 1.8 mM monopotassium phosphate (KH 2 PO 4 ) and 5 mM β-glycerophosphate (β-GP) as external sources of phosphates. In parallel experiments, the constructs were first exposed to 100 ng/ml hr-BMP-2 or hr-DMP-1 for 24 h followed by changing the phosphates medium every 2 d. After 28 d the surfaces of randomly selected constructs from each experimental group were evaluated by SEM (as described in Section 2.4.3 ), and XRD to assess any compositional alterations of the scaffolds during the cell culture process and to identify any possible formation of mineralized tissue. Topographical evaluation of the constructs and surface elemental composition analysis were performed by SEM with additional EDS (JEOL J.S.M. 840A, Tokyo, Japan). The XRD measurements were carried out by using a diffractometer with Ni-filtered CuKα radiation (PW1710; Philips, Eindhoven and Almelo, The Netherlands).
2.8
Statistics
All experiments were run with 2–4 replicate samples and repeated at least three times. Statistical analysis of the data was performed using two-way analysis of variance (ANOVA). Follow-up comparisons between groups and treatment times were performed with Tukey’s post hoc test. Analysis was performed with Prism 6.0 Software (GraphPad, CA, USA) and the level of statistical difference was 0.05 ( p < 0.05).
2
Materials and methods
2.1
Establishment of DPSC cultures
DPSC cultures were established from third molars of young healthy donors using the enzymatic dissociation method, as previously described . Samples were collected according to the guidelines of the Institutional Review Board and donors signed an informed consent form. Briefly, the teeth were disinfected and cut around the cementum-enamel junction to expose the pulp chamber. The pulp tissue was minced into small fragments and digested in a solution of 3 mg/ml collagenase type I and 4 mg/ml dispase II (Invitrogen, Karlsruhe, Germany) for 1 h at 37 °C. Single cell suspensions were obtained by passing the cells through a 70 μm cell strainer (BD Biosciences, Heidelberg, Germany). The cells were then expanded with a-MEM (Minimum Essential Media) culture medium (Invitrogen), supplemented with 15% FBS (EU-tested, Invitrogen), 100 mM l -ascorbic acid phosphate (Sigma–Aldrich, Taufkirchen, Germany), 100 units/ml penicillin, 100 mg/ml streptomycin and 0.25 mg/ml Amphotericin B (all from Invitrogen) (=Complete Culture Medium – CCM) and incubated at 37 °C in 5% CO 2 . Cultured DPSCs in passage numbers from 2 to 8 from at least two donors were used for all experiments with similar results.
2.2
Characterization of DPSC cultures with flow cytometry
DPSCs were extensively characterized by flow cytometry for several mesenchymal (CD90/Thy-1, CD73, CD29/b1-integrin, CD49f/a6-integrin, CD81-TAPA, CD166/ALCAM, CD146/MUC18, STRO-1, CD34), neural (CD271/NGFR, nestin), endothelial (CD105/endoglin, CD106/VCAM), hematopoietic (CD117/c-Kit, CD14, CD45) and embryonic (Nanog, Oct3/4, TRA-1-60, TRA-1-81, SSEA-1, 3, -4, -5) stem cell (SC) markers, as previously described . Briefly, cells were trypsinized, washed with PBS and then stained with the following fluorochrome-conjugated mouse anti-human antibodies: CD90-FITC (fluorescein isothiocyanate) CD73-PE (phycoerythrin), CD29-APC (allophycocyanin), CD49f-APC, CD81-FITC, CD166-PE, CD146-PE, STRO-1-FITC, CD34-APC, CD271-PE, CD105-FITC, CD106-APC, c-kit/CD117-PerCP-Cy5.5 (Peridinin-Chlorophyll-Protein Complex), CD14-FITC, CD45-PE, TRA-1-60-PE, TRA-1-81-APC, SSEA-1-PE, SSEA-3-PE, SSEA-4-FITC, SSEA-5-APC (all from BioLegend, Fell, Germany). For intracellular staining for Nanog, Oct3/4 and nestin, the cells were first fixed with a 4% paraformaldehyde buffer, permeabilized with a 0.1% saponin buffer (both from BD Biosciences) and then stained with the mouse anti-human antibodies Oct3/4-Alexa Fluor 647, Nanog-PE (both from BD Biosciences) and nestin- APC (RnD Systems, Minneapolis, USA). The stained cells were washed twice with staining buffer (PBS + 1% BSA + 0.1% NaN3) and analyzed using a BD LSR II Flow Cytometer. A total of 100,000 events were acquired for each sample. Data were analyzed using Summit software, version 5.1 for Windows (Beckman Coulter, Inc. Krefeld, Germany).
2.3
Preparation of 3D bioceramic scaffolds
Ceramic scaffolds were synthesized with the foam replica technique using a sol–gel derived glass composition in the system (SiO 2 = 60, MgO = 7.5, CaO = 30, ZnO = 2.5 in wt%) . The sol–gel solution was prepared as described by Theodorou et al. by the hydrolysis of tetraorthosilicate (TEOS) and the addition of calcium nitrate tetrahydrate (Ca(NO 3 ) 2 ·4H 2 O), magnesium nitrate hexahydrate (Mg(NO 3 ) 2 ·6H 2 O) and zinc nitrate hexahydrate (Zn(NO 3 ) 2 ·6H 2 O) (all from Sigma–Aldrich) into the mixture. Polyurethane (PU) foam was cut into pieces 10 mm × 10 mm × 5 mm and immersed in the sol–gel solution described above. After immersing the foam in the sol–gel and mechanical stirring for 5 min, the samples (green bodies) were retrieved and squeezed to remove the excess of sol from the pores and then left to dry out for at least 12 h. The thickness of the bioactive glass on the green bodies was adjusted using droplets of sol–gel and the excess removed after centrifuging the green bodies. Next, the synthesized scaffolds were sintered at 890 °C (10 °C/min) with 2 h annealing, as previously described . The fabricated scaffolds presented 84% porosity and pore interconnectivity, a mean compressive strength of 0.10 (±0.06) MPa and controlled degradation rate of 3.5% after 120 h immersion in Tris Buffer solution used for the simulation test and 5% in citric acid solution used for the extreme test .
2.4
Preparation and characterization of human treated dentin matrices (hTDMs)
2.4.1
Preparation of hTDMs
hTDMs were prepared using crowns of impacted third molars of young healthy donors obtained after routine extraction. The extracted teeth were preserved in PBS containing 2x antibiotics/antimycotics, in order to reduce their microbial load and maintain their moisture level. Periodontal tissues were scraped away and the coronal part of the tooth separated from the root by a transverse section at the cemento-enamel junction. Dental pulp tissues and part of the pre-dentin were also removed by mechanical means. Experimental cavities (approx. 4 mm × 4 mm × 2.5 mm) were then prepared inside the tooth crowns to host the scaffolds ( Fig. 1 ). Cavity preparation was followed by a multi-step soaking procedure to achieve dentin demineralization, as already described by Li et al. . In detail, the prepared crowns were immersed in deionized water (dH 2 O) for 20 min in an ultrasonic cleaner followed by 40 min immersion in dH 2 O without sonication. Then dH 2 O was changed and the same procedure repeated a further 4 times for a total of 5 h. The crowns were then treated (hTDM) with descending concentrations of the chelating agent Ethylene Diamine Tetraacetic Acid – EDTA (Sigma–Aldrich), as follows: 17% EDTA followed by 10% and 5% treatment, each for 5 min. After each EDTA treatment step, a washing step with dH 2 O for 2 min in an ultrasonic cleaner was performed. The hTDMs were then stored in sterile PBS, supplemented with antibiotics/antimycotics for 24 h and then disinfected by UV irradiation for 30 min before being used for further experiments.
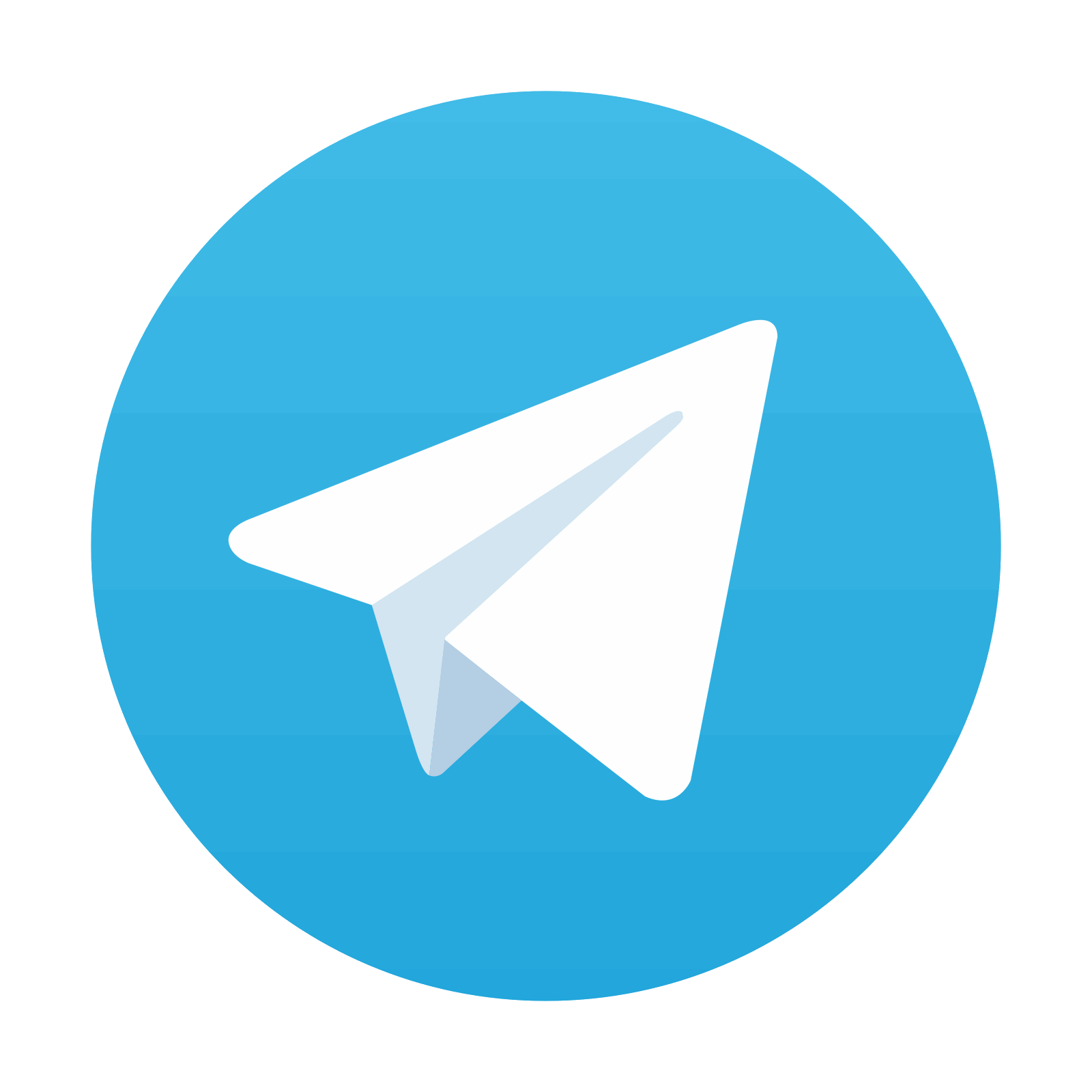
Stay updated, free dental videos. Join our Telegram channel

VIDEdental - Online dental courses
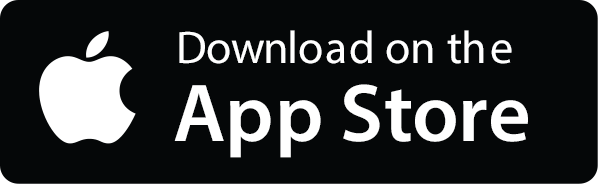
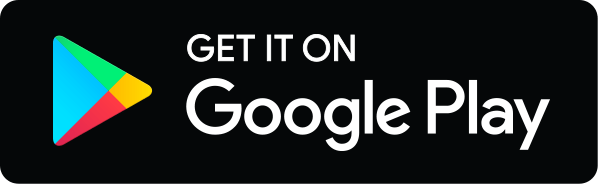
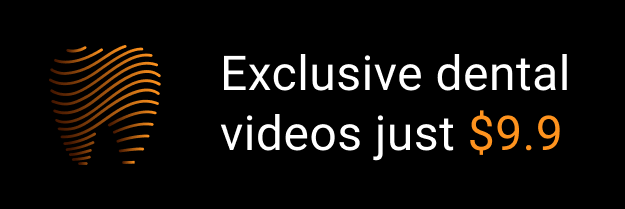