Abstract
This study evaluated the effects of homogenous demineralized dentin matrix (HDDM) slices and platelet-rich plasma (PRP) in surgical defects created in the parietal bones of alloxan-induced diabetic rabbits, treated with a guided bone regeneration technique. Biochemical, radiographic, and histological analyses were performed. Sixty adult New Zealand rabbits were divided into five groups of 12: normoglycaemic (control, C), diabetic (D), diabetic with a PTFE membrane (DM), diabetic with a PTFE membrane and HDDM slices (DM–HDDM), and diabetic with PTFE membrane and PRP (DM–PRP). The quantity and quality of bone mass was greatest in the DM–HDDM group (respective radiographic and histological analyses: at 15 days, 71.70 ± 16.50 and 50.80 ± 1.52; 30 days, 62.73 ± 16.51 and 54.20 ± 1.23; 60 days, 63.03 ± 11.04 and 59.91 ± 3.32; 90 days, 103.60 ± 24.86 and 78.99 ± 1.34), followed by the DM–PRP group (respective radiographic and histological analyses: at 15 days 23.00 ± 2.74 and 20.66 ± 7.45; 30 days 31.92 ± 6.06 and 25.31 ± 5.59; 60 days 25.29 ± 16.30 and 46.73 ± 2.07; 90 days 38.10 ± 14.04 and 53.38 ± 9.20). PRP greatly enhanced vascularization during the bone repair process. Abnormal calcium metabolism was statistically significant in the DM–PRP group ( P < 0.001) for all four time intervals studied, especially when compared to the DM–HDDM group. Alkaline phosphatase activity was significantly higher in the DM–HDDM group ( P < 0.001) in comparison to the C, D, and DM–PRP groups, confirming the findings of intense osteoblastic activity and increased bone mineralization. Thus, HDDM promoted superior bone architectural microstructure in bone defects in diabetic rabbits due to its effective osteoinductive and osteoconductive activity, whereas PRP stimulated angiogenesis and red bone marrow formation.
The goal of bone tissue engineering (BTE) is to create bone grafts that enhance bone repair following trauma, infection, or neoplasm, and for developmental abnormalities; these procedures represent a challenge in maxillomandibular complex surgery.
To obtain effective bone regeneration or osseointegration the following are necessary: osteoprogenitor and osteoconductive cells, which offer potential to differentiate and facilitate the various stages of bone regeneration, growth factors, structural integrity with the absence of any kind of local infection, and, in long bones, mechanical immobilization.
Many strategies have been used to accelerate bone repair or to act as an alternative bioactive material scaffold, including demineralized dentin matrix (DDM) and platelet-rich plasma (PRP). PRP contains several proteins that are of great benefit in wound healing. These proteins regulate cell migration and proliferation (mitogenesis), remodel the extracellular matrix, and promote angiogenesis, creating a favourable environment that quickly stimulates tissue repair and wound healing.
Various studies on the complications of diabetes mellitus have demonstrated extensive alterations to bone and mineral metabolism, causing diabetic osteopenia. Some of the factors that have been associated with the pathogenesis of this bone disorder include hyperglycaemia, oxidative stress, and body weight loss. Thus, hyperglycaemia may exert its detrimental effects on bone cells by increasing oxidative stress, characterized by a high level of reactive oxygen species (ROS), causing an imbalance in intracellular reduction–oxidation and, as a consequence, inducing cellular dysfunction. Accumulating evidence also suggests that mesenchymal stem cells (MSCs) are adversely affected by hyperglycaemia, which compromises their differentiation and function and may result in low bone turnover and formation. Additionally, researchers have reported that diabetes decreases the percentage of osteoclasts and osteoblasts, enhances apoptosis of osteoblasts, and reduces osteocalcin synthesis, leading to significant bone loss and a delay in dynamics of the bone regeneration process. In a study by Vieira et al., diabetes mellitus was induced experimentally in rabbits with monohydrate alloxan and this triggered noticeable oral and general clinical complications, including weight loss, polyuria, polyphagia, and severe chronic gingivitis. Furthermore, bone repair in surgical defects was significantly impaired.
Based on these investigations, the effects of homogenous demineralized dentin matrix (HDDM) and PRP on the bone repair of calvarial defects in diabetic rabbits, using a polytetrafluoroethylene (PTFE) membrane to accomplish the guided bone regeneration technique, were evaluated. Biochemical measurements of calcium, phosphorus, and alkaline phosphatase (ALP) in the blood plasma, measurements of optical density, and histological analyses were performed.
Materials and methods
Animals
Sixty healthy adult New Zealand rabbits, 30 male and 30 female, with an average age of 4–6 months and an average body weight of 3.5 kg, were selected. These rabbits were divided into five groups of 12 rabbits (each with six males and six females): normoglycaemic rabbits (control, C), diabetic rabbits (D), diabetic rabbits with PTFE membrane (DM), diabetic rabbits with PTFE membrane and slices of HDDM (DM–HDDM), and diabetic rabbits with PTFE membrane and PRP (DM–PRP). The experiment was approved by the Animal Experimentation Ethics Committee of the Institute of Science and Technology – Campus São José dos Campos/UNESP.
Preparation of the biomaterials
The biomaterials used were HDDM and PRP. The HDDM was obtained from the central incisors of the normoglycaemic rabbits. This was prepared and then cut into slices of 8 μm in thickness by frozen microtomy, according to the method described by Gomes et al. The PRP was obtained from a blood sample taken from the left ventricle of the heart. This sample was placed into two 5-ml sterile test tubes containing 0.5 ml of buffered sodium citrate (anticoagulant). The blood was then centrifuged at 1200 rpm for 10 min at 20 °C. The plasma and red blood cells were separated into a sterile test tube. Next, the plasma was removed and a second centrifugation performed, identical to the first; the red blood cells located in the bottom of the test tube were discarded. After centrifugation, the plasma presented as two portions: upper (supernatant) and lower. The upper portion contained platelet-poor plasma (PPP), which was discarded; the lower portion contained PRP, which was pipetted and placed in a sterile test tube. Thereafter, the PRP was mixed with 10% calcium chloride solution (proportion 8:1) and kept in a water bath at 37 °C until it reached a gel-like consistency. This bioactive product was used in the surgical bone defect immediately after preparation. This method has been described previously by Monteiro et al.
Induction of diabetes and blood glucose measurement
Diabetes mellitus was induced by a single intravenous injection of 90 mg/kg monohydrated alloxan (Sigma-Aldrich, St Louis, MO, USA) dissolved in 5 ml of NaCl (0.15 mol/l). This was administered promptly to the anesthetized rabbit through the marginal ear vein. The animals received 10% glucose in drinking water for the first 24 h for the transient hypoglycaemia that develops after alloxan treatment and were then placed on regular rabbit chow and water. Hyperglycaemia was indicated when the blood glucose level was above 200 mg/dl. NPH human insulin (Humulin N-100; Eli Lilly do Brasil, São Paulo, Brazil) and regular human insulin (Biohulin R-100; BioBRÁS, Minas Gerais, Brazil) were administered subcutaneously to maintain a stable serum glucose level between 200 mg/dl and 350 mg/dl. The blood glucose level was monitored at 24, 48, and 72 h after alloxan treatment and three times a day thereafter until sacrifice. The animals had free access to tap water; however, the daily food ration was controlled (50 g per rabbit per day) to ensure equal intake in all diabetic groups.
Creation of bone defects
The animals underwent general anaesthesia via intramuscular xylazine (0.1 mg/dl, Rompun; Bayer, São Paulo, Brazil) and ketamine (0.25 mg/dl, Ketalar; Holliday-Scott, São Paulo, Brazil). The rabbits were shaved and an incision made, after which a circular bone defect was created surgically in the parietal bone with the aid of an 8.0-mm trephine under irrigation with 0.9% sterile saline solution. The HDDM slices were placed around the edges of the surgical bone defect promoting a haemostatic action, while the PRP was used to entirely fill the cavity ( Fig. 1 ). The PTFE membrane was placed on the external surface and floor of the bone defect in the DM, DM–HDDM, and DM–PRP groups. This membrane was placed 3 mm beyond the limits of the defect to ensure the guided bone regeneration technique. For the control, D, and DM groups, the bone defects were totally filled by a blood clot. The periosteum, muscle, and skin were then closed with 6–0 Vicryl absorbable suture (Ethicon/Johnson and Johnson, Brazil).

Histology
Three anaesthetized animals in each group were euthanized at time intervals of 15, 30, 60, and 90 days. The bone that contained the surgical defect area was fixed in 10% formalin for 72 h, decalcified in Plank–Rychlo solution (AlCl 2 ·6H 2 O (126.10 g), HCl (85 ml), HCOOH 88% (52 ml), and distilled water (500 ml)), and embedded in paraffin. Histological sections were cut to a thickness of 5 μm, stained with haematoxylin–eosin, Masson’s trichrome, and Schmorl’s stains, and described histologically.
Biochemical parameters
For the biochemical analysis, a colorimetric method was used and absorbance readings obtained using a spectrophotometer (model DU- 600B; Beckman Coulter Inc., Brea, CA, USA). Analytical reagents were also used (Labtest Diagnóstica S.A., Minas Gerais, Brazil). After the animals had been anaesthetized, a 10-ml blood sample was collected through cardiac puncture of the left ventricle, with the aid of a heparinized hypodermic syringe, and placed in an ice bath to maintain the enzymatic properties of the ALP. Subsequently, these were centrifuged at 4100 rpm for 10 min at 4 °C to obtain the plasma. Thereafter, the plasma levels of calcium (Ca), phosphorus (P), and ALP were analyzed. These samples were processed in triplicate and the average value calculated for each biochemical parameter studied.
The colorimetric methods used to measure the plasma levels of calcium, phosphorus, and ALP have been described by Moorehead and Biggs, Fiske and Subbarow, and Coleman, respectively. To determine the calcium concentration, cresolphthalein complexone calcium was measured in a spectrophotometer at a wavelength of 550 nm. For determination of the phosphorus concentration, the sample was diluted 1:5 with 10% trichloroacetic acid (TCA). The phosphomolybdate complex is reduced by ascorbic acid, producing a blue colour; this was measured in a spectrophotometer at a wavelength of 880 nm. For ALP, thymolphthalein monophosphate substrate was used; after its hydrolysis the thymolphthalein is released, indicating the activity of this enzyme. The absorbance of this process was measured in a spectrophotometer at a wavelength of 590 nm.
Optical density
To obtain digital radiographic images of the specimens, a sensor-type image plate (charge-coupled device; Trophy Radiologie, Vincennes, France) was used, and the radiographs were exposed at a fixed focal distance (ffd) of 40 cm and settings of 7 mAs and 65 kVp. The sensor plate images were read by the software VixWin 1.9 (Gendex Dental Systems, Hatfield, PA, USA) and these images were transferred to a computer according to bone mineral density features. Measurements of these digital images were made by software that allows the measurement of 256 grey-scale levels (Aldus Photo Styler 2.0; Kodak). One observer measured the optical density of each radiographic image three times at intervals of 5 days; the average value was then used in the statistical analysis.
Histomorphometry
For histomorphometric analysis, a Zeiss II reticule was placed over a compensation microscope (10×, W-PI; Carl Zeiss, Oberkochen, Germany) to measure the quantity of new bone tissue. The reticule image was superimposed on the desired histological field. The reticule points ( Ni ) and the total number of points over the bone defect ( N ) were counted. The amount of newly formed bone tissue was evaluated through the following formula: bone mass = Ni / N . Approximately 100 sections were obtained from each bone defect selected for examination. Of these sections, four were chosen at random for the histomorphometric analysis. Subsequently, eight histological fields from the surgical bone defect region were analyzed. For this step, a 20× objective lens (A-Plan; Carl Zeiss) and a 10× ocular lens (W-PI; Carl Zeiss) were attached to an optical microscope (Axioskop 40; Carl Zeiss). The objective showed a 100-point reticule encompassing a bone tissue area of 7840 μm 2 . This method has been described by Gomes et al.
Statistical analysis
Standard deviations of 3, 5, and 7 units were obtained by means of a pilot study for the biochemistry, optical density, and histomorphometry analyses, respectively, using Minitab software version 17.1.0 (Minitab Inc., State College, PA, USA). From these values, five animals per group were deemed necessary for each observation period ( n = 4) in order to attain 80% test power.
Quantitative results, expressed as the mean and standard deviation (SD), were submitted to analysis of variance (one-way ANOVA) and the Tukey test using GraphPad InStat software version 5.0 for Windows 7 (GraphPad Software, San Diego, CA, USA). The level of significance was set at P < 0.05.
Results
Histomorphological analysis
15 days
For the C group, the bone defect was found to be filled with connective tissue with osteogenic activity and a few immature bone trabeculae. The bone trabeculae were localized mainly at the periphery and were growing towards the centre of the defect; however some islets of new bone tissue were also randomly evident. Undesirable tissues could be found inside the defect. The band of newly formed bone at the defect site was thin when compared with the original cortical bone ( Fig. 2 a).
For the D group, the histological features were similar to those of the C group; however there was a reduced amount of newly formed bone trabeculae ( Fig. 2 b).
For the DM group, the defect was filled with immature bone trabeculae and osteogenic connective tissue; nevertheless remnants of the blood clot and granulation tissue were still found at the centre of the defect. The PTFE membrane acted as a biological barrier to the undesirable tissues and presented osteoconductive properties. The band of new bone tissue was of about the same thickness as the original cortical bone ( Fig. 2 c).
For the DM–HDDM group, the defect was filled with numerous immature bone trabeculae and osteogenic connective tissue. These trabeculae were largely found at the periphery and were growing rapidly towards the centre of the bone defect ( Fig. 2 d). The HDDM slices were surrounded by numerous osteoblasts, osteoprogenitor cells, and osteoid tissue, as well as being incorporated within the newly formed bone tissue. Additionally, osteoclasts were found on the surface of the HDDM slices, promoting their resorption ( Fig. 3 ). No sign of rejection of the HDDM was observed.
For the DM–PRP group, the defect was filled with dense and loose connective tissue and a few thin newly formed bone trabeculae in a linear arrangement ( Fig. 2 e). Dense connective tissue showed osteogenic activity, whereas the loose tissue presented myxoid features and angiogenic activity. The myxoid tissue consisted of delicate collagen fibres, numerous spindle cells, and a large amount of extracellular amorphous matrix. The bone trabeculae were mostly at the periphery of the bone defect, growing towards its centre. The band of new bone tissue at the defect site was inferior in relation to the original cortical bone.
30 days
For the C group, the defects were filled with bone trabeculae and osteogenic connective tissue. These trabeculae were mature at the periphery and immature at the centre of the bone defect, thus centripetal bone growth was seen. The band of neoformed bone was thinner than the original cortical bone.
For the D group, the defect was filled with immature bone trabeculae and osteogenic connective tissue. The amount and arrangement of the new bone trabeculae were very similar to those of the C group sections.
For the DM group, the histological features were also similar to the C group. The osteogenic connective tissue was well-organized, showing collagen fibres parallel to each other. Immature bone marrow tissue was located mainly at the periphery of the defect. The band of newly formed bone tissue had about the same thickness as the original cortical bone.
For the DM–HDDM group, the defect was almost entirely filled with new bone trabeculae. Thick bone trabeculae and bone marrow tissue with reduced spaces were seen. HDDM slices had been incorporated within the newly formed bone tissue. Remnants of the odontoblastic process were in evidence inside the HDDM slices on Schmorl staining. Numerous osteoblasts populated the surface of the HDDM, promoting deposition of newly formed bone matrix.
For the DM–PRP group, the defect was filled with immature bone trabeculae, connective tissue with myxoid and osteogenic features ( Fig. 4 a ), and bone marrow tissue. The bone trabeculae showed a uniform, delicate, linear arrangement in parallel with the PTFE membrane, showing its osteoconductive properties. Very intense vascularization was evident, due to the angiogenic activity of the connective tissue which was stimulated by the PRP. As a consequence, there was a rapid formation of bone marrow tissue that was mainly located in the central portion of the bone defect ( Fig. 4 b). The band of newly formed bone tissue was uniform and of similar thickness to the original cortical bone.
60 days
For the C group, the defect was mostly filled by mature bone tissue, which was distributed regularly throughout; however some osteogenic connective tissue areas were still evident. Several osteoclasts were present, in order to start the bone remodelling process. The band of new bone tissue was thinner than the original cortical bone.
For the D group, the defect was largely filled with dense connective tissue and discrete bone trabeculae that were distributed randomly. The connective tissue was fibrous and disorganized due to the irregular arrangement of the collagen fibres. The bone trabeculae were mature at the periphery but immature at the centre of the bone defect. The amount of new bone tissue remained lower in comparison to the other groups. In some sections, undesirable tissues were still found inside the defect region.
For the DM group, the defect was filled with new bone tissue and dense connective tissue with numerous collagen fibres. The features of bone maturation and its appearance were similar to those of the D group. The bone trabeculae were distributed regularly, showing centripetal growth. The band of new bone tissue was of the same thickness as the original cortical bone.
For the DM–HDDM group, the bone defect was totally filled with mature bone trabeculae and bone marrow tissue. These trabeculae were well-delimited and distributed irregularly. The cortical bone of the bone defect area was not well-structured. Some HDDM slices were still incorporated inside the new bone tissue. The bone marrow spaces were large and mostly filled with yellow bone marrow. A panoramic view showed that the thickness of the defect region was higher than the limits of the original cortical bone.
For the DM–PRP group, the defect was filled with new bone trabeculae, bone marrow tissue, and connective tissue with osteogenic and angiogenic activities. The bone trabeculae were mature at the periphery but immature at the centre of the bone defect area. They were also distributed irregularly and were interconnected. The medullary spaces were large and filled mainly with red bone marrow. In some sections, connective tissue with myxoid features was still found. A panoramic view showed that the thickness of the bone defect region was similar to the original cortical bone.
90 days
For the C group, the defect was filled with mature bone trabeculae, some fibrous connective tissue, and medullary spaces of variable size. Rare islets of newly formed bone trabeculae were also found. In all specimens, undesirable tissue was seen; therefore the thickness of the cortical bone was not preserved ( Fig. 5 a ).
For the D group, the defect area was filled with connective and new bone tissue. There was a high amount of fibrous connective tissue, which was distributed across the entire bone defect region. Very few haversian systems were in evidence. Undesirable tissues were present in all histological sections and consequently the cortical bone was thin ( Fig. 5 b).
For the DM group, the defect was filled with mature bone tissue, red and yellow bone marrow, and fibrous connective tissue. The new bone tissue was well-organized, showing thick and regular bone trabeculae distributed across the whole defect area. The cortical bone of the surgical defect region was preserved in comparison with the original cortical bone ( Fig. 5 c).
For the DM–HDDM group, the defect region was completely filled with mature bone trabeculae and bone marrow ( Fig. 5 d). Even though its architectural microstructure was reconstructed, new bone tissue presented exophytic growth. The physiological conversion from red to yellow bone marrow was clearly evident, and the medullary spaces were large and regular. The resorption process of the HDDM slices presented the following phases: (1) the dentin matrix was first incorporated into the newly formed bone tissue; (2) next, the dentin was degraded during bone remodelling; and (3) the area in which the dentin matrix was located was replaced by new bone tissue ( Fig. 6 ).
For the DM–PRP group, the defect was filled with mature bone trabeculae, bone marrow, and fibrous connective tissue that was located mainly in the central portion. The medullary spaces were large and filled predominantly by red bone marrow. The bone trabeculae showed a regular, homogeneous, linear arrangement. The thickness of the defect area slightly exceeded the limits of the original cortical bone in most of the specimens ( Fig. 5 e).
Statistical results
To interpret the statistical results of the effects of HDDM and PRP on the bone repair process in diabetic rabbit calvarial defects, as shown in the tables, statistical significance was considered at the levels P < 0.05, P < 0.01, and P < 0.001; significance was mainly shown for the DM–HDDM and DM–PRP groups. In the tables, the five groups are represented by the following lowercase letters: C (a), D (b), DM (c), DM–HDDM (d), and DM–PRP (e). The letters ‘abcde’ indicate the statistical significance in relation to the experimental groups; where a group of these letters is found, the result for the group represented by the first letter is statistically significant in relation to the others.
Biochemical analysis
The blood plasma levels of calcium, phosphorus, and ALP were quantified for the diabetic rabbits after creation of the calvarial bone defect that was treated with HDDM or PRP in the guided bone regeneration technique.
With regard to the calcium concentration, the DM–HDDM group presented a low plasma level and steady state when compared to the other groups. The DM–PRP group showed statistical significance in relation to the DM–HDDM and DM groups ( P < 0.001) for all periods, D group at 15 days ( P < 0.001) and 30 days ( P < 0.01), and the C group at 90 days ( P < 0.001). The D group presented a statistical difference in relation to the C and DM–HDDM groups at 60 days ( P < 0.001) and the C, DM, and DM–HDDM groups at 90 days ( P < 0.001). For the phosphorus concentration, there was steady state in the plasma levels of the experimental groups for all studied periods; therefore no significant difference was found among the groups. For ALP enzymatic activity, the DM–HDDM group showed a statistical difference when compared with the C, D, and DM–PRP groups for all periods ( P < 0.001), whereas for the DM–PRP group this was statistically significant only in relation to the D group at 15 days ( P < 0.05), 30 days and 60 days ( P < 0.01), and 90 days ( P < 0.001). Moreover, the DM group ( P < 0.001) presented expressive statistical difference in relation to the C, D, and DM-PRP groups at all periods, with exception of the DM-HDDM group in the periods of 30 days, 60 days, and 90 days ( Table 1 ).
Days after surgery | Plasma levels | C (a) | D (b) | DM (c) | DM–HDDM (d) | DM–PRP (e) | ||||||||||
---|---|---|---|---|---|---|---|---|---|---|---|---|---|---|---|---|
Mean ± SD | Median | Min–Max | Mean ± SD | Median | Min–Max | Mean ± SD | Median | Min–Max | Mean ± SD | Median | Min–Max | Mean ± SD | Median | Min–Max | ||
15 | Ca | 19.0 ± 1.10 | 18.4 | 17.7–19.8 | 15.0 ± 0.70 | 14.7 | 14.5–15.9 | 12.0 ± 2.80 | 11.2 | 11.2–16.2 | 11.0 ± 0.60 | 11.8 | 10.6–11.8 | 21.9 ± 2.90 ebcd*** | 23.5 | 2.40–23.7 |
P | 3.0 ± 0.10 | 3.4 | 3.4–3.5 | 3.0 ± 0.40 | 2.3 | 2.2–2.9 | 3.0 ± 0.05 | 2.9 | 2.9–3.0 | 3.0 ± 0.30 | 3.1 | 2.7–3.4 | 3.0 ± 0.10 | 3.4 | 3.2–3.4 | |
ALP | 15.0 ± 1.20 | 15.5 | 14.3–16.6 | 8.0 ± 0.80 | 8.1 | 7.3–8.9 | 63.0 ± 1.70 cabde*** | 64.4 | 61.7–65.0 | 47.0 ± 3.80 dabe*** | 46.3 | 42.60–51.9 | 19.0 ± 1.00 eb* | 18.5 | 17.6–19.6 | |
30 | Ca | 19.0 ± 2.90 | 20.1 | 16.2–21.8 | 16.0 ± 0.20 | 16.4 | 16.3–16.6 | 10.0 ± 1.00 | 10.2 | 10.0–11.9 | 11.0 ± 1.30 | 10.3 | 10.3–12.1 | 21.7 ± 2.70 ecd***,eb** | 23.2 | 23.2–23.3 |
P | 3.0 ± 0.10 | 3.3 | 3.0–4.2 | 3.0 ± 0.30 | 2.6 | 2.2–2.9 | 3.0 ± 0.50 | 2.8 | 2.6–3.6 | 3.0 ± 0.10 | 3.3 | 3.3–3.9 | 3.0 ± 0.30 | 2.7 | 2.6–3.2 | |
ALP | 15.0 ± 0.70 | 15.5 | 15.5–16.7 | 8.0 ± 0.90 | 8.3 | 7.2–8.9 | 62.0 ± 2.80 cabe*** | 63.0 | 59.0–64.0 | 49.0 ± 2.80 dabe*** | 48.1 | 48.1–48.1 | 25.0 ± 2.90 eb** | 19.1 | 18.7–20.5 | |
60 | Ca | 19.0 ± 2.60 | 18.2 | 20.3–22.2 | 21.0 ± 1.10 bad*** | 20.8 | 17.1–22.5 | 11.0 ± 1.50 | 11.9 | 10.5–12.0 | 10.0 ± 0.60 | 10.9 | 10.1–11.0 | 19.7 ± 0.60 ecd*** | 19.5 | 19.3–20.4 |
P | 3.0 ± 0.10 | 3.7 | 3.1–4.3 | 3.0 ± 0.20 | 2.7 | 2.5–2.8 | 3.0 ± 0.20 | 2.7 | 2.4–2.9 | 3.0 ± 0.20 | 3.4 | 3.0–3.5 | 3.0 ± 0.40 | 2.9 | 2.6–3.3 | |
ALP | 15.0 ± 0.70 | 15.0 | 14.3–15.1 | 6.0 ± 0.50 | 6.5 | 5.0–7.0 | 56.0 ± 2.20 cabe*** | 56.9 | 54.0–57.0 | 41.0 ± 2.60 dabe*** | 42.0 | 38.0–50.6 | 18.0 ± 0.90 eb** | 18.0 | 17.3–19.0 | |
90 | Ca | 18.0 ± 0.50 | 17.7 | 17.7–18.0 | 24.0 ± 0.70 bacd*** | 24.9 | 23.9–25.2 | 12.0 ± 0.50 | 12.4 | 12.0–13.2 | 12.0 ± 0.90 | 12.6 | 11.6–12.9 | 24.7 ± 0.60 eacd*** | 24.1 | 24.0–25.4 |
P | 3.0 ± 0.30 | 3.1 | 2.8–3.5 | 2.0 ± 0.30 | 3.2 | 3.1–3.4 | 3.0 ± 0.30 | 2.5 | 2.0 – 2.6 | 3.0 ± 0.30 | 3.9 | 3.2–3.2 | 3.0 ± 0.20 | 2.9 | 2.5–3.5 | |
ALP | 15.0 ± 0.70 | 15.5 | 15.5–16.7 | 5.0 ± 0.50 | 5.5 | 5.4–6.4 | 42.0 ± 2.60 cabe*** | 43.7 | 40.7–44.4 | 47.0 ± 3.90 dabe*** | 47.1 | 44.4–50.0 | 20.0 ± 1.40 eb*** | 19.5 | 19.0–21.6 |
Optical density analysis
When the parietal bone fragments were removed during the creation of the bone defects in the healthy rabbits (C group), six fragments were selected at random to obtain the standard optical density of the original parietal bone. The digital radiographic images were measured and the mean value was found to be 81.21 ± 16.56. The DM–HDDM group showed variable statistical significance in relation to the other groups. The differences between the DM–HDDM group and the C group ( P < 0.05), D and DM groups ( P < 0.01), and DM–PRP group ( P < 0.001) at 15 days were statistically significant. At 30 days the difference was significant for the D group ( P < 0.01). At 60 days the difference was significant for the D group ( P < 0.01) and DM–PRP group ( P < 0.05), and at 90 days it was significant for the C, D, and DM groups ( P < 0.001) and the DM–PRP group ( P < 0.01) ( Table 2 ). The DM–HDDM group showed the highest optical density value for the bone defect area when compared with the standard optical density of the original parietal bone at 90 days. Additionally, well-defined and round, radiolucent areas of a large size and with irregular limits were found in some diabetic rabbits. This bone rarefaction was distributed particularly in the frontal and occipital bones, suggesting diabetic osteoporosis. These radiographic features were present mainly at 60 and 90 days.
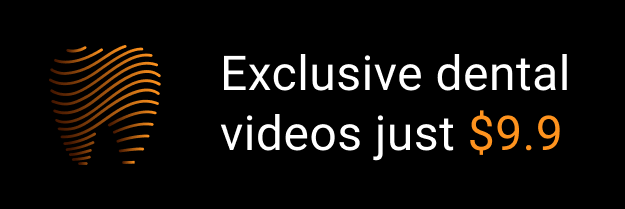