Michael Miloro (ed.)Trigeminal Nerve Injuries201310.1007/978-3-642-35539-4_1© Springer-Verlag Berlin Heidelberg 2013
1. Historical Perspectives on Trigeminal Nerve Injuries
(1)
Department of Oral and Maxillofacial Surgery, Virginia Commonwealth University, Medical College of Virginia, Richmond, VA, USA
(2)
Virginia Tech Carilion School of Medicine, Roanoke, VA, USA
Abstract
Understanding and treating the effects of human neurotrauma was hampered for centuries by ancient misunderstandings of the primary loci of sensory perception. However, elegant descriptions were made of the clinical effects of nerve injuries suffered by Civil War survivors, and research following the World Wars explained many of the underlying neural mechanisms. In the nineteenth century, nerve injuries had been treated unsuccessfully by secondary neural ablations, and fortunately, in the twentieth century, this led to therapies designed to repair nerves and enhance regeneration, enabled by studies of animal injury models, the introduction of the operating microscope, and proof that microsurgical techniques used for repair of hand injuries could be successfully adapted to epineurial trigeminal nerve repairs.
Understanding and treating the effects of human neurotrauma was hampered for centuries by ancient misunderstandings of the primary loci of sensory perception. However, elegant descriptions were made of the clinical effects of nerve injuries suffered by Civil War survivors, and research following the World Wars explained many of the underlying neural mechanisms. In the nineteenth century, nerve injuries had been treated unsuccessfully by secondary neural ablations, and fortunately, in the twentieth century, this led to therapies designed to repair nerves and enhance regeneration, enabled by studies of animal injury models, the introduction of the operating microscope, and proof that microsurgical techniques used for repair of hand nerve injuries could be successfully adapted to epineurial trigeminal nerve repairs.
The modern era has demonstrated that direct end-to-end trigeminal nerve repairs can result in high levels of useful functional sensory recovery even following long intervals after partial trigeminal injury. Historically, entubulation repairs of larger nerve gaps have been largely unsuccessful in the trigeminal system, although interpositional nerve grafting has proven useful and has been enhanced recently by the development of processed cadaveric allografts and promising research with stem cell tissue engineering. Reversal of neuropathic pain through peripheral nerve surgery, however, has continued to be disappointing, as well as the failure to incorporate knowledge of nerve injury and neuropathic pain mechanisms into the medical and dental curriculum.
Injuries to the nerves of the jaws and face have surely been a part of life since the dawn of mankind. Yet, it has only been in the last three centuries that the devastating effects of peripheral nerve injuries have begun to be appreciated, and a scientific basis for effective treatments has emerged.
This opening chapter will plot the beginnings of published knowledge regarding neurotrauma. It will review what has been learned in the last two centuries, especially about early descriptions of the clinical effects of nerve injuries resulting from the American Civil and World Wars. It will summarize the main findings that underlie understanding of the effects of neurotrauma and recognize the roles that outstanding neuroscientists and clinicians have contributed along the way. It will focus especially on the foundations of microsurgery that led to modern techniques for treating trigeminal nerve injuries, noting the progress that has been made in the last few decades toward finding more useful diagnostic methods and implementing more effective surgical and nonsurgical treatment modalities.
Finally, because a prime purpose of historical knowledge is the impact that it may have on directing the future, this chapter will end with a section on “Unfinished Business” that will highlight the current challenges and offer a “wish list” that could lead to a deeper understanding of nerve injury effects, more accurate clinical diagnoses, more appropriate treatment regimens, and enhanced professional education that will facilitate healthcare access and delivery for patients who sustain nerve injures.
1.1 The Beginnings of Knowledge
Ancient understandings of peripheral nerve injury effects were hampered for many centuries by devotion to the philosophies of Aristotle and Hippocrates (460–370 B.C.) that placed functions of the “five senses” and “soul” in the heart, rather than the nervous system [1]. Galen (A.D. 131–200) however doubted the Aristotelian views. Galen’s experiments included the sectioning of the recurrent laryngeal nerves of newborn pigs and supported his conclusions that the peripheral nerves and the brain are the mediators of sensation [82]. In support of Galen’s theories, Rene Descartes speculated in the year 1664 that sensations were mediated through “fast moving particles of fire…(that) pass(es) through the nerve filament until it reaches the brain” [25]. He also observed that “suffering may follow amputation.”
Considering the early confusion regarding the functional role of peripheral nerves, there are remarkable early reports of injured nerve treatments. In the thirteenth century, William of Salicito (Bologna) reportedly rejoined the ulnar nerve of an Italian soldier, and a detailed description of suture repair was made by Gabriele Ferrara in 1608 [6, 38]. In 1847, Sir James Paget sutured the median nerve of an 11-year-old boy, reportedly achieving “complete recovery” [144]. In an experimental milestone, Augustus Waller [137] created glossopharyngeal (IX) and vagus (X) nerve injuries in frogs and described gross degeneration distal to the site of injury, regeneration of axons in proximal segments, and greater preservation of nerves in younger compared to older frogs [137].
Better understanding of the anatomic effects of nerve injuries, however, depended upon the development and use of the light microscope by Anton van Leeuwenhoek (1632–1677). Additionally, Ramon y Cajal from Spain, the father of neuroanatomy and winner of the 1906 Nobel Prize shared with Camillo Golgi, used silver staining to demonstrate nerve microstructure, degeneration of fibers distal to nerve injury sites, and Schwann cell guidance of regeneration cones [118]. Cajal’s work also predicted the presence of “synapses,” a term coined by Sherrington (1857–1952) to designate the connections between nerves [130].
The physiologic effects of nerve injury were also being revealed by the mid-eighteenth century. Galvani (1737–1798) had demonstrated that nerve fibers respond to electrical stimuli [84]. Electrical “action potentials” were described in peripheral nerve fibers by von Helholz (1821–1894) and Duchenne, who measured conduction velocities in human nerves [33]. This information laid the groundwork for the 1948 Nobel Prize work by Erlanger and Gasser, who demonstrated nerve action potentials in teased single nerve fibers. This revealed that nerves contain a spectrum of fibers from fine tactile A-fibers to C-fiber nociceptors that would later become the primary targets for neurophysiologic testing of patients with nerve injuries [34].
1.2 The Clinical Features of Nerve Injuries: Lessons from the Historic Wars
The major wars of the past two centuries were followed by spurts of knowledge concerning the clinical effects of nerve injuries. The greatest impetus came from the American Civil War with the pioneering observations of Silas Weir Mitchell (1864–1906) [101, 102]. As Senior Acting Assistant Surgeon in the Union Army at the 400-bed Turners Street Philadelphia Hospital, he treated hundreds of nerve-injured patients, mostly survivors from Gettysburg, and, assisted by his son, followed their courses for 40 years. Through his remarkable observations and publications, he revealed many of the prime clinical features of traumatic neuropathies that have been verified by subsequent clinicians and neuroscientists. Some key nerve injury features credited to SW Mitchell include:
1.
Phantom phenomena, referred pain, and daily pain cycles
2.
Burning pain (causalgia) (an intractable post-traumatic collision effect of autonomic and somatosensory functions linking temperature, environmental, and psychiatric factors)
A 24-year-old member of the 26th Pennsylvania Volunteers was shot at Chancellorsville through the leg. He lay in the field for 28 h before wet compresses were put on his leg. At that time, he began to complain of severe burning in his heel and showed (minimal) sensory loss.
3.
Touch-evoked pain (as a common effect of nerve trauma)
Agents usually felt as touch only becoming painful is sufficiently common after many forms of injury.
4.
Psychogenic impact of nerve injury (describing “traumatic neurosis” and anticipating WWI descriptions of “battlefield fatigue” and the modern diagnosis of post-traumatic stress disorder (PTSD))
Perhaps few persons who are not physicians can realize the influence which long continued and unendurable pain can have on the body. When terrible pain has lasted a few days or weeks, the whole surface becomes hyperesthetic, with every vibration, and changes of light, brings on new agony. Under such torments, the temper changes, the most amiable grow irritable, the soldier becomes a coward, and the strongest man is scarcely less nervous than the most hysterical girl.
5.
Gender differences in chronic pain
Moreover, the greatest number of such cases was women, and the hysterical element comes largely into view as the disorders progress.
6.
Intractability of nerve injury impaired functions
Entire restoration of function-sensation after nerve injury was extremely rare.
7.
Dysesthesias greater with partial than with complete nerve injuries
The greatest symptoms were due to wounds of small filaments; few instances of like symptoms have followed those of the great trunks.
8.
Centralization of traumatic neuropathies and neuralgias
It is quite rare for a patient with neuralgia to arouse from sleep with pain. Those who suffer with causalgia find pain immediately on awakening.
9.
Neuroviral lesion exacerbations with nerve injuries
At some time in the history of a nerve injury, it is common to see active forms of eruptions, ulcers, or herpetic vesicles or in the shape of bullae.
10.
Trigeminal injury features
John Schultz, a 23-year-old German who joined the Wisconsin Volunteers was injured on July 3, 1863, while marching in an assault line at Gettysburg. The injury, which was deduced to be from a picket sniper above the victim, resulted from a Minie ball which avulsed his ear lobe, entered his right cheek beneath the malar bone, passed through the ascending ramus of the mandible and exited through the submandibular space, reentered and fractured the clavicle, and came to rest in the trapezius muscle. The Minie ball, much deformed, was removed two weeks after the injury by Dr. Keen. Patient displayed initial anesthesia over the mental nerve distribution, accompanied by eventual “tingling pain” from moving the lip.
Considered a primary founder of the specialty of neurology, SW Mitchell is also viewed among the most versatile Americans since Benjamin Franklin. The first half of his life was devoted to scientific achievements, and the second half to a literary career. He wrote 19 novels including one published when he was 84, seven books on poetry, a historical book on Washington, and a story of “Kris Kringle” which sometimes gets credit for initiating the idea of Santa Claus.
Early investigators from this era including SW Mitchell, Henry Head, and James Sherren heroically experimented with self-imposed nerve injuries. Mitchell froze his own ulnar nerve to study the effects on hand sensation, describing the overlap of cutaneous nerves adjacent to injured nerves. Henry Head damaged his own radial nerve and described the phenomena of first (A-delta fiber) pain and second (C-fiber) pain that he labeled “protopathic and epicritic” [58]. Mitchell also championed the use of animal experimentation in medical research in an era when the use of dogs for scientific purposes was vigorously opposed (as it is presently) (“Vivisection: A Statement in Behalf of Science, 1888”). He argued that only scientists should decide whether, when, and in what way animals should be used, “(o)therwise in a matter involving the interest of the community, those who know would be directed by those who do not know.”
1.3 Treating Nerve Injuries in the Early Twentieth Century: Neural Ablation
In the aftermath of the Civil War, early twentieth-century treatments for patients with painful trigeminal nerve injuries centered on destructive peripheral nerve lesioning techniques. Harvey Cushing used extirpation of the entire Gasserian ganglion frequently [20]. Peripheral neurectomy of the trigeminal nerve branches, particularly infraorbital and mental branches, were widely used in the early decades by neurosurgeons and some oral and maxillofacial surgeons, often applied after local anesthetic blockade had demonstrated acute pain relief. Although useful for patients with short life expectancies and classic trigeminal neuralgia, neurectomy proved unsuitable for long-term symptom resolution, instead producing cases of “anesthesia dolorosa” in the deafferentated nerve distributions [49, 68].
It had also been observed that surgical resections of traumatic neuromas, even cauterizing or capping the resected nerve ends with epineurial sutures, failed to prevent reformation of painful neuromata [141]. This experience led White to speculate that “(t)he surest way to prevent recurrence of traumatic neuroma-in-continuity is to resect the scarred area and do end-to-end anastomosis of healthy ends of the nerve.”
Nevertheless by the mid-twentieth century, other forms of neuro-destructive lesions were in widespread use and also included varying concentrations of alcohol injections, phenols (glycerol), formalin, and even hot boiling water [21, 142]. Unfortunately, these procedures often led to secondary inflammatory neuritis, exacerbated neurosensory functional impairments and dysesthesias, and largely fell out of routine clinical use.
There are surviving legacies of these early neuroablative procedures extending into the modern era, however, which employ more selective treatments of damaged and painful injured trigeminal nerves. Partial and reversible neurolysis using radiofrequency thermal neurolysis at 65–75 °C have been shown to depress pain from C-fiber and A-delta fiber pain hyperfunctioning while retaining the potential for eventual recovery of tactile functions [52]. More recently, radiosurgical (“gamma knife”) lesioning of trigeminal sensory roots has shown promise for mitigating traumatic trigeminal neuralgias while preserving some touch and proprioceptive functions [72].
1.4 The Emergence of Balanced and Multidisciplinary Nerve Treatments
Dr. John Bonica, Professor of Anesthesiology at the University of Washington, had studied patient responses to injuries on the WW II beaches of Anzio, Italy, and returned to establish a first-of-its-kind multidisciplinary clinic in Seattle for treating patients with chronic post-traumatic pain [12]. Bonica argued that future scientific and patient care progress would benefit from multidisciplinary collaborations of clinicians and researchers. Bonica’s vision led to the establishment of the International Association for the Study of Pain (IASP) and the current journal known as Pain.
Bonica’s multidisciplinary approach also recognized the need for balanced surgical and nonsurgical therapies and less-destructive approaches for the treatment of painful nerve injuries. Two promising nonsurgical approaches that came to the forefront during the last century are neural blockade and neural stimulation.
1.4.1 Neural Blockade
The early twentieth century witnessed a “Golden Age of Regional Neural Blockade” for the treatment of injured and painful nerve distributions [13]. Local anesthetics were shown to provide remissions that far outlasted the expected drug effects and often lead to overall improvements in both clinical symptoms and neural functions. Even in the modern era, neural blockade remains an important modality for managing chronic dysesthesias using serial nerve trunk blockade with long-acting anesthetic agents, often combined with adrenocorticosteroids [119].
Recent extensions of the neural blockade concept have led to the development of low-risk topical multimodal compounds containing agents such as ketamine, tricyclic antidepressants, alpha-antagonists, pre-gabalins, and lidocaine that can be applied peripherally to injured trigeminal nerve distributions [39, 59, 73, 107]. And, in a return to the early twentieth-century experience with neural blockade, there have been recent promising reports of the use of botulinum toxin A for the management of intractable trigeminal neuralgias, although its mechanism of action is not presently fully understood [81, 148].
1.4.2 Neural Stimulation
The approach to treating neuropathic conditions through physiologic counter-stimulations is as old as recorded history [71]. Egyptian tombs from the year 2750 B.C. contained pictures of electric Nile catfish being used to treat human afflictions. Aristotle and Galen both advocated attaching electric torpedo ray fish to the face and head for treating headaches. The Chinese, of course, are known to have treated painful injuries using electrical and thermal acupuncture stimulation applied at key peripheral neuroanatomic “meridians” for over 4,000 years. And, although classical acupuncture remains very popular internationally for a wide range of painful conditions, acupuncture in any form has not been proven superior to placebo for neurotraumatic dysesthesias in reviews of controlled studies [35]. Even in the nineteenth century, “electrogalvanism” was briefly considered as a surgical analgesic for the extraction of teeth [41]. More recently, electrotherapy has proven useful for the management of nerve injury pain [79].
Interestingly, the electrophysical characteristics of the torpedo fish, with voltage ranges of 40–50 V, are similar to energy ranges used in a variety of artificial electrotherapy analgesia techniques developed in recent decades to treat nerve injury pain: notably transcutaneous electrical nerve stimulation (TENS), implanted stimulation electrodes, and intracerebral brain stimulation therapies [69].
TENS, as a form of low-level electrical stimulation analgesia, came into widespread use in the 1970s, triggered by Melzack and Wall’s 1965 “Gate Control Theory of Pain” [86]. This theory predicted that stimulation of intact large fibers in peripheral nerves could inhibit the pain-producing small C-fibers known to be found in excess following peripheral injury [11]. Unfortunately, TENS therapy has not had a wide application for trigeminal nerve injuries despite its early clinical promise [77].
More aggressive electrical impulse therapies delivered through implanted paraneural electrodes have been effective for spinal nerve injury pain management and show promise for treatment of trigeminal traumatic neuralgias [64, 129].
Intracerebral stimulation therapies (ICS), developed in the 1970s for control of Parkinsonian tremors, known as deep-brain stimulation (DBS) have also proven useful for relief of neuropathic pain [53]. More recently, motor cortex stimulation (MCS) systems have proven of benefit to patients with intractable nerve injury pain, including those with extreme deafferentation dysesthesias [105, 117].
A recently introduced benign form of stimulation therapy that appears to support recovery after nerve injury is laser therapy. “Low-level laser” (LLL) or “cold laser” or “soft laser” photoirradiation stimulations applied to nerve distributions using the 650–850 nm portion of the visual spectrum have shown promise in published case series, although its efficacy beyond placebo has been questioned [4, 92, 98].
1.5 Legacies of WW II and Beyond: Understanding Mitchell’s Observations
Much like the Civil War, World War II was followed by expanding knowledge regarding nerve injuries. Sir Henry Seddon is credited with providing the scientific foundations for peripheral nerve surgeries, including suggestions that nerve grafts might be used for this purpose. He also posed a nerve injury classification system based upon progressive anatomic derangements, coining the terms “neurapraxia, axonotmesis, and neurotmesis” [127]. Sunderlund later further subdivided the scope of nerve injuries into five types (grades I–V) and then further modified by Mackinon and Dellon to include a sixth type of mixed nerve injury (grade VI) [80, 132].
A sensory functional classification of nerve injury recovery also emerged from British WWII experiences. The Highet classification has a starting point of no sensory detection (anesthesia) and progresses through the attainment of noxious and crude touch detections, and finally fine touch abilities, with or without hyperesthesia. This system has subsequently led to a consideration that intermediate crude touch levels can be considered “useful sensory recovery” by the British Medical Research Council Scale (MRCS) [10].
In the decades following WW II, many of the neural mechanisms underlying Mitchell’s seminal clinical observations regarding nerve injuries have been clarified, including the following:
1.
Burning pain
He lay in the field for 28 h before wet (cold) compresses were put on his leg. At that time he began to complain of severe burning in his heel and showed sensory loss.
It was theorized in 1944 that “cross innervations” may form between damaged somatosensory nerve fibers and adjacent sympathetic nerve fibers [31, 46]. Subsequent researchers verified that upregulated sympathetic postganglionic nerve “baskets” may form around damaged sensory ganglia and also sprout to form hyperactive couplings with sensory fibers, termed “ephapses” within traumatic neuromas [26, 42, 85, 126]. Trauma may also induce chemically mediated non-synaptic excitation in sensory ganglia after peripheral nerve injury [3].
The experience of many trauma patients complaining of burning pain aggravated by cold temperatures or stimuli (cold hyperalgesia) has been further attributed to ectopic impulses generated by C-fiber nociceptors [120] as well as coupling with autonomic fibers [43]. For patients with this form of “sympathetic-mediated pain” mechanism, stellate ganglion anesthetic blocks are often effective for chronic pain management [13, 115].
In 1959, Noordenbos, using nerve fiber spectrum analysis, showed that early regenerated nerves have a paucity of myelinated large A-beta fibers and an excess of regenerated smaller nerve fibers [104]; clinical neurosensory studies have verified that A-fiber fine tactile mechanoreceptive functions are the last to recover after nerve injury (“first to be lost and last to recover”). Patient complaints of chronic constant paresthesias (“numbness”) and painful dysesthesias (“aching, burning”) after nerve injury have been shown to be due to involvement of hyperactive small C-fibers [19].
2.
Tingling paresthesias and pain
Patient displayed initial anesthesia over the mental nerve distribution, accompanied by eventual “tingling pain” from moving the lip.
Scientific explanations of clinical “triggering” of painful and non-painful paresthesias began to emerge with the work of Hoffman and Tinel in 1915 [16]. They described the clinical phenomenon of tingling and shock-like sensations that are elicited by digital tapping over distal portions of regenerating nerves. These clinical signs were felt to represent “the presence of young axons in the process of growing.” Subsequent experiences, however, have shown that the “Tinel’s sign” may be easily misinterpreted, and, rather than a positive sign of spontaneous neural regeneration, it may also represent a poor sign of chronic peripheral neuropathy with neuroma formation.
Foerster had demonstrated painful responses to percussion over previously damaged nerve trunks with or without Tinel’s sign, and he coined the term “hyperpathia” [40]. Modern studies have verified that hyperpathic clinical signs can represent sites of sensitized neuroma-in-continuity, as well as chronically sensitized amputation-type neuromas. Considerable variability in traumatic trigeminal neuromas has been seen under operating microscopes, and mechanisms of peripheral sensitization within neuromas have been clarified [50, 51]. Painful neuromas have been shown to contain chronic inflammatory cells, as well as cannabinoid and heat-sensitive vanilloid 1 (proinflammatory) receptors [5, 9].
3.
Partial vs. complete nerve injuries
The greatest symptoms were due to wounds of the small filaments; few instances of the symptoms have followed those of the great trunks.
Research with both nerve-lesioned animals and patients with known types of nerve injuries has led to the conclusion that neuropathic pain is often inversely related to the degree of nerve damage [62, 149]. Quantitative neurosensory testing of patients with trigeminal nerve injuries has verified that higher retention of A-beta fibers is correlated with early triggered forms of pain, whereas delayed and disturbing paresthesia, often accompanied by dull aching and burning pain, is correlated with excess regeneration of C-fibers in more completely damaged nerve distributions [19, 63].
4.
Pain elicited by touch
Agents usually felt as touch only become painful is sufficiently common after many forms of injury.
Mitchell had observed that some nerve-injured patients displayed a paradoxical syndrome that combines loss of sensation (“numbness”) with an explosive hyperphenomena, manifesting as triggered paresthesias and pain. Over the years, accumulating literature has attributed touch-evoked pain (allodynia) and heightened responses to painful stimuli (hyperalgesia) to both peripheral and central neural “sensitization” [136, 145]. Research has shown that uninjured large myelinated A-beta fibers contained within the trunks of traumatized nerves become upregulated with inflammatory vanilloid receptors and display ectopic and low-threshold activities that partially explain the resultant allodynia [15]. These mechanisms also explain how immediate-onset pain and allodynia may occur within 1–3 days after partial nerve injury. In contrast, delayed-onset pain and hyperalgesia developing 3–4 weeks after nerve injury are attributed to the regeneration of excessive C-fibers as well as central sensitization [27, 45].
5.
Centralization after peripheral nerve injury
It is quite rare for a patient with neuralgia to arouse from sleep with pain.
Mid-1970s research with vital staining of sensory root and spinal subnuclei after experimental trigeminal (V) nerve transections confirmed that peripheral injuries can induce “plastic” central degeneration [47, 48]. These studies established an important link explaining clinical nerve injuries and central nerve derangements [66]. Histochemical studies have now shown that the deafferentated neurons of the central trigeminal complex become chronically “sensitized” by glial cells that stimulate NMDA (N-methyl-D-aspartate) receptor excitotoxicity [124, 125, 147]. Similar neuroexcitation may also occur at higher ascending levels and combine with loss of descending inhibition to further magnify “central sensitization,” contributing to clinical hyperalgesia and allodynia [32, 108, 145].
The realization that the CNS undergoes “plastic” responses to peripheral nerve injury has also led to modern rehabilitation therapies that seek to induce central nervous “recovery.” Dellon has advocated “sensory reeducation” exercise techniques that can induce “reverse plasticity” effects in thalamic and cortical perceptual regions following injured and repaired peripheral nerves [23]. Recent studies have verified that sensory reeducation techniques can be effectively applied to trigeminal nerve injury conditions generated by mandibular sagittal split osteotomies [90, 109].
6.
Phantom paresthesia sensations
I am more sure of sensing the leg which ain’t than the one which are.
Patients with chronic trigeminal injuries often complain of impaired oral-facial functions and disturbing paresthesias that include perceived movement “phantoms” and tingling “afterimages” [54]. Livingstone theorized that phantom phenomena could be due to hyperactivity in traumatic neuromas, a concept that has been supported by modern demonstration of ectopic spiking activity in experimental and human neuromas [76, 120]. Phantom paresthesias have also been attributed to anatomic and physiologic responses at many other levels of the CNS resulting from sensory deafferentation [78].
A central nervous basis of phantom phenomena was revealed in a landmark 1984 experiment in which selected digits in monkeys were amputated, resulting in major anatomic derangements in their somatosensory cortical maps [88]. More recent f-MRI (functional MRI) imaging has exposed central demyelination lesions in human thalamic and cortical regions in response to repetitive injury and chronic neuropathy [83, 134].
7.
Intractability of impaired functions
Entire restoration of function-sensation after nerve injury was extremely rare.
Mitchell’s frustration in 1860 with the intractability of nerve injury functional impairments unfortunately would still have persisted 100 years later, awaiting new biologic and technical means of restoring neural function.
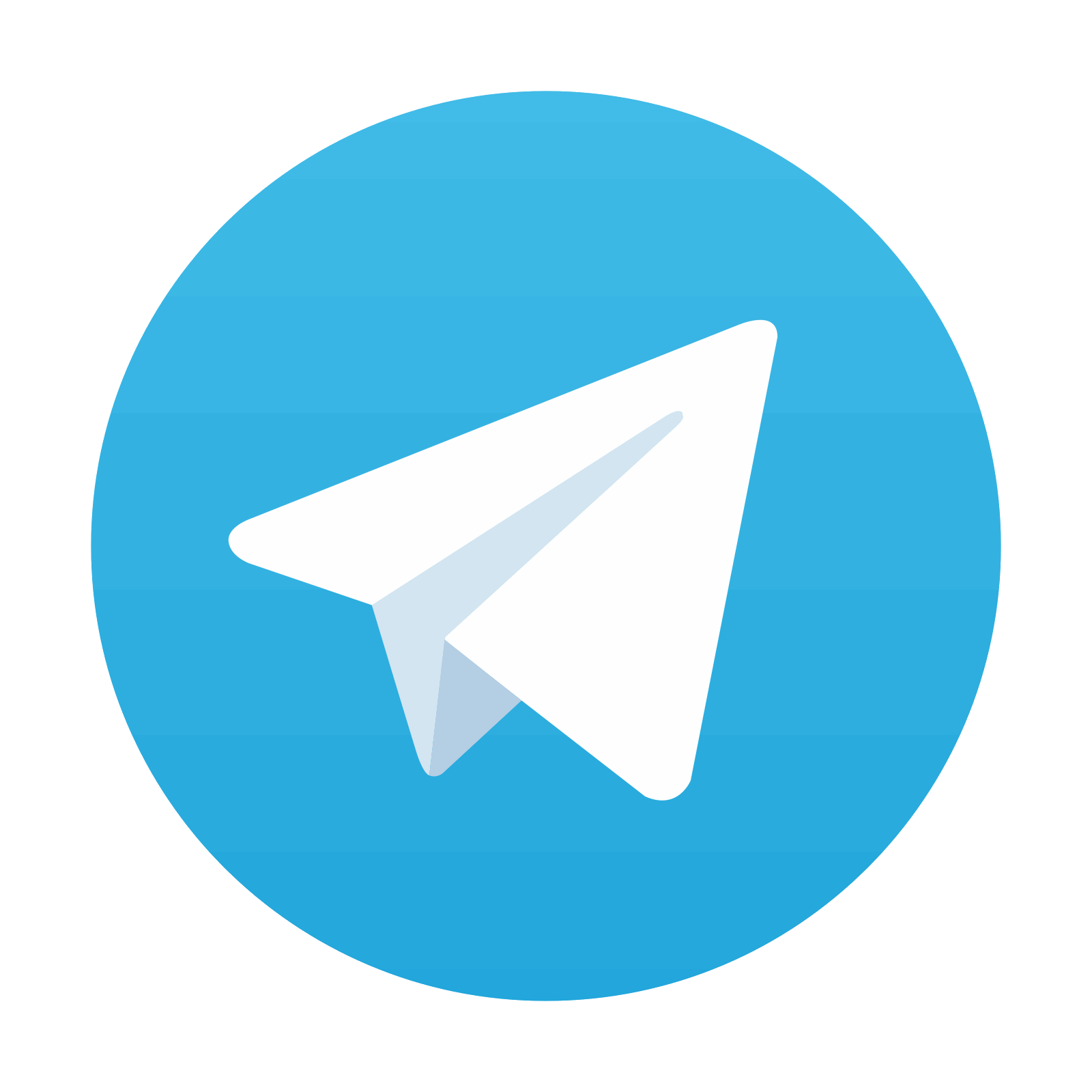
Stay updated, free dental videos. Join our Telegram channel

VIDEdental - Online dental courses
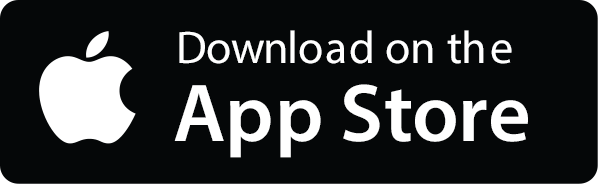
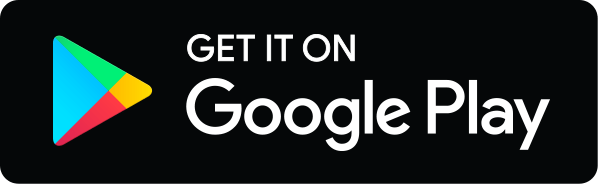