Fig. 23.1.
This EDA implant reveals bone accumulation along its basal and lateral surfaces up to below the first rib of the threaded pin. The total bone height above the implant disk was around 2–3 mm. The disk was located just above the maxillary sinus
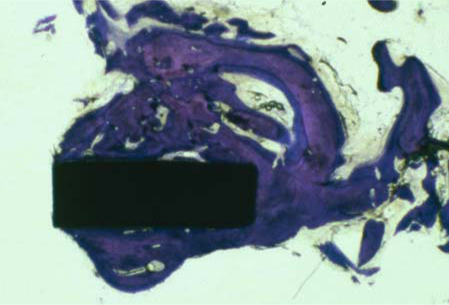
Fig. 23.2.
Central portion of the web bar of the same implant. Again, virtually the entire surface area is perfectly osseointegrated
The next specimen (Fig. 23.3) is a vestibular ring segment of an EDAS implant that was removed after around 1 year of clinical service in the position of tooth 37 (mesiodistal view). Only the surface-enlarged portion of the ring was explanted.
Two human explant specimens exhibited direct contact of the surrounding bone structure with the implants. In one case, the surface-enlarged portion of the threaded pin revealed direct bone contact as well. Both findings – i.e. direct osseointegration along the ring structures, including the web bars, and along the surface-enlarged shaft portions – were consistent with the histological findings obtained in animal specimens.
23.1.2.2 Histological Analysis of Animal Specimens
The histological specimens illustrated in the following paragraphs had been collected 14–18 weeks after implantation. The experiments had originally been performed to evaluate bone formation patterns on BOI implants of different surface types.
A total of five dogs had been treated with implants for this purpose. All animals were 20–25 months old at the time of implantation, that is, all of them were fully grown. The premolars of all dogs had been removed 3 months before the procedure. In one case, the horizontal portions of the lower canines were left in place in order to simulate implant-induced intrabony tooth trauma and to verify how the tissue reacts to such trauma.
Dogs 1 and 2 were treated with three BOI implants each on the left side of the jaw. The two anterior implants were immediately loaded with metallic crown blocks, while the third implant was left without restoration (Fig. 23.4).
Furthermore, three solid screw implants (Allfit, STI) were inserted on the right side of the jaw for comparison, again followed by immediate loading of the two anterior implants with metallic crown blocks. Due to an error by the surgical team, the implant beds in dog 2 were prepared with the same instruments that had been used immediately before in dog 1. The implant sites in dog 2 were therefore more contaminated and less sterile following preparation. The implant specimens of both dogs were collected 4 months after implantation (Figs. 23.4–23.6).
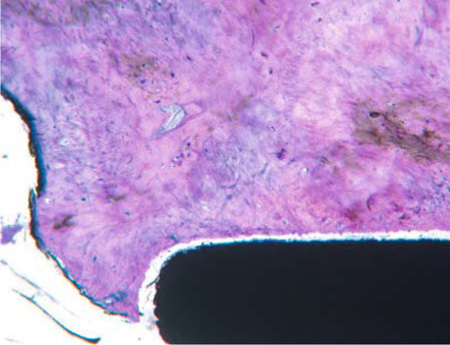
Fig. 23.3.
Fully osseointegrated ring disk area. The distance between the titanium and the bone structure was produced artificially during the process of fixation and drying. The secondary osteons were diagonally cut in accordance with the principle direction of load transmission in the mandible
Another implant-tissue specimen (dog 3) was obtained after an unloaded solid screw implant (Allfit, STI) had been inserted to study bone regeneration. In addition, a bone defect was induced on the vestibular aspect below the implant collar, so that one-third of the implant surface was denuded. The subsequent membrane-based attempt at augmentation did not influence the regenerative process in a significant way. The specimens from this animal were collected 3 months after implantation (Fig. 23.7).
No antibiotics were used during the procedure. Tetracycline i.v. was administered as a label 1 week before specimen collection.
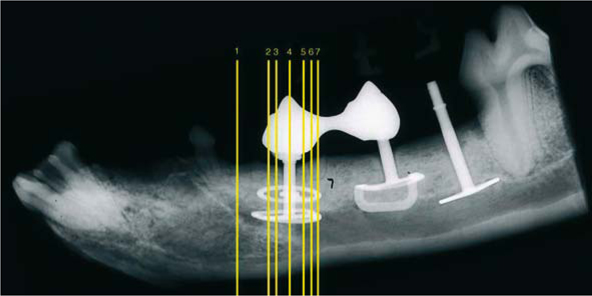
Fig. 23.4.
Lateral radiograph of a dog’s mandible with BOI implants in place. All implant disks feature a diameter of 10 mm. The disk-to-disk distance of the left implant is 3 mm
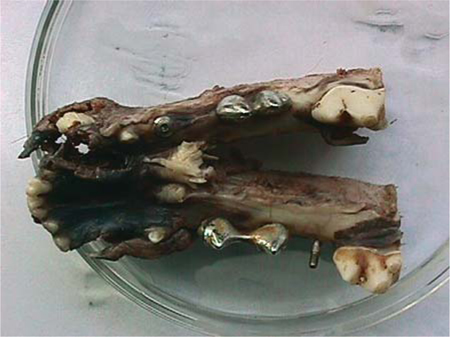
Fig. 23.5.
Dog’s mandible with three implants on each side of the jaw. The presence of attrition on the metallic crown restorations indicates that the implants were subjected to adequate functional loading
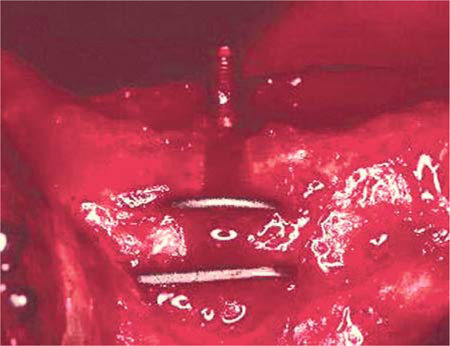
Fig. 23.6.
The disks of the anterior implant projected from the vestibular bone surface following insertion. This situation is quite commonly observed in human patients as well
Bone blocks containing the implants were collected for histological analysis, dried using established techniques, and embedded in polymethacrylate. Up to six ground sections for each BOI implant, and one section for each screw implant, were obtained (Plenck 1986).
All screw implants featured a sandblasted enossal surface. The BOI implants from dog 1 were shot-peened until the surfaces were perfectly smooth. In dog 2, the load-transmitting surface was enlarged up to the border of the threaded pin with aluminium oxide.
In dogs 4 and 5, implants were placed both in the maxilla and in the mandible. Some implant segments were located along the maxillary sinus. All implants in dogs 3 and 4 were inserted uncleaned under non-sterile conditions. In dog 4, transosseous ligatures were placed that induced massive inflammation of the alveolar mucosa and the mandibular bone.
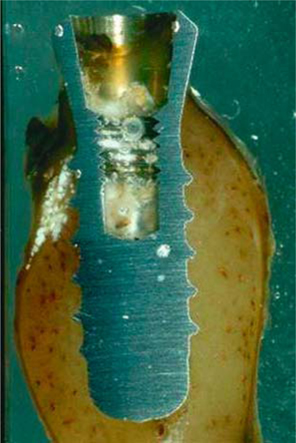
Fig. 23.7.
Screw implant in the mandible after non-loaded healing
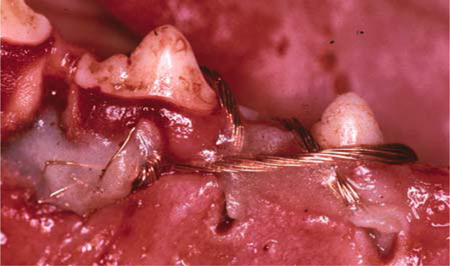
Fig. 23.8.
This picture illustrates how copper ligatures and plastic covers were applied to obstruct drainage conditions in the mandible of dog 4, thus inducing severe osteomyelitis associated with bone necrosis
This experiment was designed to investigate whether the good healing results obtained after non-sterile insertion of BOI implants the first time around was a random or systematic finding. Another purpose was to find out whether osseointegrative healing of BOI implants in dogs can be prevented at all.
23.1.3 Histological Results for BOI Implants
All implants that had been inserted under sterile conditions and immediately loaded were associated with comprehensive modelling. Among the BOI implants, this modelling was almost exclusively observed on the lingual side.
The BOI implants that had been inserted in dog 2 under non-sterile conditions were found to be equally well osseointegrated as the implants in dog 1. The remodelling processes crestally to the implant disk were, however, substantially more comprehensive after non-sterile insertion. Lingual remodelling, by contrast, was minimal in infected sites (Fig. 23.17). While the process of bone healing was apparently slower after non-sterile insertion, close osseoadaptation of the load-transmitting implant segments was nevertheless achieved in principle.
The bulky implant body inserted under sterile conditions also failed to induce subperiosteal modelling in the premolar area of the mandible in the absence of immediate loading.
23.1.4 Histological Results for Crestal Implants
The immediately loaded screw implants were associated with both lingual and vestibular modelling. Appositional lingual modelling was more pronounced, however. The crestal implants were associated with remodelling on the vestibular longitudinal side of the implant, while lingual remodelling compared to the preoperative situation was largely absent.
As expected, no osseointegration was observed with the crestal implants that had been inserted under non-sterile conditions followed by immediate loading. The jaw segments in question did not reveal any subperiostal modelling. In fact, there was hardly any of the pre-existing osteonal bone structure left. Almost the entire jaw was pervaded by woven bone. While osseointegration was achieved with the third ipsilateral implant, which had been left non-loaded, this implant was not associated with any modelling from the periosteum either. Apparently the jaw segment had been subjected to pronounced osteomyelitis that was subsequently repaired by woven bone formation. All crestal implants which had been inserted under non-sterile conditions lacked integration by histological criteria, whereas all crestal implants which had been inserted under sterile conditions have been integrated well into the bone.
23.1.5 Discussion and Clinical Application
Bone tissue resembles the structural properties of composite material by permitting crack formation while resisting crack propagation to prevent downright failure (Martin and Burr 1989).
Judging from its architecture, the mandible is essentially a long tubular bone (Ashman and van Buskirk 1987). Due to the strong flexion and the associated overlapping of trajectories, the interforaminal area is a particularly stable place to insert implants. In the posterior segment of the mandible, the typical tubular bone structure is largely re-established in the phase of bone resorption following tooth loss.
The bone turnover rate in the mandible is known to be very high (Bays 1986). In addition, Atkinson et al. (1977) demonstrated that bone density varied in different regions of the mandible and that any damage inflicted to bone structures during remodelling did not have the same effect throughout the mandible. Following tooth extraction, bone density in areas exceeding the alveola changed. In the initial phase, the density would decrease because tunnelling BMUs would increase the porosity of the jaw. The effects of implantation would spread much like waves in a pond on throwing a stone. The tunnelling secondary osteons (BMUs) would induce transient weakening of bone structures even in areas that were located far away from the alveolus. The area of the extraction socket was afflicted by disuse atrophy. This process was delayed in cases where the extraction socket was filled with crestal implants, which would promote bone mineralization to the point where the degree of bone density would sometimes even exceed the original level. Nine months after implantation, however, resorption processes that adversely affected the preservation of alveolar bone were seen in the area of the (non-loaded) implant.
On balance, the experiments performed by Atkinson et al. yielded no evidence of any changes on the lingual side of the mandible following insertion of crestal implants, which is basically in keeping with our own findings obtained with unloaded implant fixtures. The extent to which the entire bone structure will react to the implant procedure also depends on the size of the implant relative to the size of the jaw.
The results of this follow-up investigation suggest that the functionally induced propagation of cracks and microcracks caused by the implantation procedure will give rise to a process of subperiosteal modelling that will ensure the continuity of the jaw. In the absence of any chewing loads acting on the implants, the cracks will not propagate but are allowed to heal locally, so that additional bone volume to preserve the continuity of the jaw is not required.
If the formation of new bone does not take place after implants have been inserted in the mandible, the chances are that the principal load-transmitting trajectories will remain unchanged (Atkinson et al. 1977). Further studies are needed to learn more about the fate of any bone structures that are newly formed after implantation procedures. Generally speaking, the further remodelling process can be expected to develop in accordance with Wolff’s law (Wolff 1892), which includes the following concepts:
-
The bone structure is optimized for strength and weight.
-
The trabeculae are arranged in accordance with the main direction of forces.
-
The bone structure regulates itself through cells reacting to mechanical stress.
If the newly formed bone structures remain localized and undergo mineralization, then the chances are that other areas whose preservation is not a principal concern will be subjected to remodelling. This could mean that the probability of long-term implant survival may vary depending on whether immediate or delayed loading is used, since both concepts involve different preconditions for longterm remodelling of the jaw areas harbouring the implant sites. On the other hand, situations are to be expected in which the newly installed implants will cause the masticatory function to improve and the chewing forces to increase. Apparently, the newly formed bone structures are preserved in this situation because they are needed. The reader is referred to Chapter 9 for a detailed discussion of this topic including relevant clinical observations.
If long tubular bones are subjected to increasing loads, particularly in the context of rapid growth in young individuals, woven bone is built up from the periosteal area, and endosteal apposition is observed as well (Jaworski and Uthoff 1986). Proliferation of woven bone from the periosteum may, however, also ensue in adults as a result of fatigued bone structures (Johnson et al. 1963; Uthoff and Jaworski 1985).
The principal remodelling mechanism observed in older patients in connection with increased loading is, however, endosteal apposition (Jaworski et al. 1980). Older patients show a smaller reapposition rate than younger individuals, and the diameter of the tubular bone is not observed to increase. The above investigations concerned situations in which the load increases continuously without significant numbers of cracks and serious injuries (e.g. by implantation procedures) to the cortical bone structure.
The fact that bone fractures healing in a curved position will straighten out over the years under physiologic loading conditions has been known for a long time (Jansen 1920; Basset 1965). Frost (1964) postulated that the bone reaction does not primarily result from compressive and tensile forces but from a tendency of the incoming forces to modify the excessive curvature of the bone. It must therefore be expected that the process of bone apposition will be reversed in the course of further remodelling when the jaw function will return to its initial state in the long term. The prosthetic restoration is a major determinant of long-term jaw function. In this way, the masticatory concept selected by the dentist has a decisive impact on the probability of long-term survival of enossally anchored restorations. This is particularly true of implants subjected to immediate loading.
An interesting question in connection with the present study is whether the additional subperiosteal modelling may have been induced by greater masticatory loads. At least such a connection would appear plausible in theory, since the absence of osseointegration and loading of implants would be likely to cause painful symptoms, thus giving rise to avoidance patterns and reduced chewing forces. What argues against this assumption is the fact that no modelling was observed laterally to the BOI implants that had been inserted under non-sterile conditions although these implants were immediately loaded as well. It seems more likely that the establishment of frictional bone adhesion was delayed with the BOI implants and prevented with the crestal fixtures, such that the initial crack could be repaired in the interval. In this way, there would have been no need for subperiosteal modelling. Furthermore, a delayed healing mechanism with greater initial resorption of the old bone areas would also result in a more elastic implant bed in the early postoperative phase, thereby preventing crack propagation. A relationship between implant type and the amount of newly formed bone could not be observed, which is consistent with the results obtained by Rubin and Lanyon (1984). The formation of woven bone as a rapid response to excessive flexural stresses on the side of increasing convexity is an «all-or-nothing» response because neither the number of loading cycles nor the stress levels have any effect on the degree to which the bone volume increases.
Following the formation of new bone from a periosteal direction, there is no incentive for the old bone areas affected by implantation to include the macroscopic cracks, which extend right into the cortical bone layer, in the repair work. After all, the cracks have developed longitudinally along the mandible, so that the fracture areas are slightly mobile against each other. Rather, the woven bone structures are already being pervaded by osteonal activity, while macrocracks are not systematically repaired.
Inflammatory processes of enossal origin are capable of inflicting serious damage to bone structures within a short period of time. In response to this, considerable amounts of woven bone may be formed from the endosteal and periosteal surfaces. In the presence of crestal implant fixtures, renewed pervasion of these areas by osteons was not demonstrable during the observation period. The level of damage inflicted to bone structures is, by contrast, much lower if infections are introduced during the insertion procedure for BOI implants – which has been known to experienced BOI users all along.
BOI implants can also be inserted in periodontally involved areas – for example in single-step procedures right after extracting any teeth that were causally related to the periodontal problem (see Chapter 8). The good results obtained in these areas are presumably due to the increased blood supply and sufficient suppuration to the associated soft-tissue structures related to the inflammatory process.
The observations made by Joos et al. (2003) that no differences between the crestal and apical implant regions were present on both the cellular and the inorganic side of the interface after immediate loading have been confirmed. Differences in bone reaction were, however, present between the vestibular and lingual sides. This was actually an expected finding, considering the small likelihood of achieving initial integration of rigid crestal implant designs into the viscoelastic environment of live bone on all sides of the implant, at the same time, and at the same high degree of mineralization. Remodelling on one side of the vertical implant surface only may also result in the biomechanically necessary adaptation of elasticity. Any mutual shifts between bone layers, including those shifts that do start from cracks or microcracks, will also affect the degree of mineralization in the interface area. More studies are needed to address the question how and why the bone conditions around the implant become homogeneous. However, while the studies by Joos et al. covered an observation period of up to 14 days, the histological implant specimens analysed in our own study had been in clinical service for more than three months. Surprisingly, the degree of primary stability achieved in the initial phase, which apparently came from the lingual side of the jaw, was good enough to ensure a smooth process of healing under masticatory function and maintaining osseointegration.
Assenza et al. (2003), by contrast, observed no differences between loaded and unloaded implants in terms of bone apposition. These authors did not, however, analyse the mandible at large but only looked at the bone structures that were directly in contact with the implants. This group also found remodelling in the crestal aspects of the bone.
At any rate, the reaction of peri-implant bone structures in the mandible following immediate loading appears to be influenced not so much by the surface design of the implant as by the masticatory forces combined with jaw flexion. After all, the same sandblasted surfaces were present both lingually and vestibularly, and completely different reactions were found on either side of the implants.
23.1.5.1 Clinical Application – BOI Implants and Jaw Fractures
In patients with hormonal bone strength deficiencies, there is a greater risk that crack propagation by immediate loading my cause jaw fractures.
We observed three cases of jaw fracture in the mandible after BOI implants had been inserted and immediately loaded in one male and two female patients known to suffer from marked osteoporosis. All three cases healed spontaneously without a problem under reduced masticatory loading (liquid diet). All fractures occurred after 6–8 weeks after the placement of the implants, and all bones fractured along and below the threaded pin. All load-transmitting disks (in areas distant from the threaded pin) were well integrated by the time the fracture occurred, such that adequate cohesion of the jaw was ensured. One may therefore cautiously conclude that a fixateur interne in the form of a fixed bridge supported by BOI implants can be considered as an alternative or supplementary treatment option to plate osteosynthesis in fractures of the edentulous resorbed mandible. This approach would offer several advantages. First of all, it would allow the physician to maintain or re-establish a physiological jaw function in a timely manner. Furthermore, the mandible would be symmetrically weakened in addition to the fracture-related elastic alterations, thus enabling a symmetrical remodelling and mineralization process throughout the entire jaw. The smooth process of fracture healing following the insertion of BOI implants for complete mandibular bridges might be supported by the fact that modelling and remodelling occurs simultaneously and symmetrically at all implant sites, such that peak stresses are avoided. It appears that patients with osteoporosis who are prone to incurring spontaneous fractures are well practised in avoiding specific bone structures. Unlike untrained dentists, they tend to regard jaw fractures not as a serious complication but as a relatively normal eventuality.
Figures 23.11–23.13 demonstrate that conditions in terms of bone mineralization may vary substantially even at different horizontal levels of the same BOI implant. Therefore, if mandibular fractures occur in one area, the other (external) areas of the horizontal load-transmitting surfaces may both take care of the load transmission and ensure the cohesion of the jaw. Currey (1981) reported extremely great differences between the mechanical properties of cortical bone structures (of different origins) even with the differences in mineralization being relatively small. What applies to different species also applies to different areas in the bones of one individual.
BOI implants appear better suited for the treatment of fracture-prone osteoporosis patients than crestal implants due to the smooth surface of their vertical component. Crestal implants have a structural requirement for surface enlargement in the vertical implant part. They carry a high risk of infection in the interface area along the surface-enlarged vertical implant axis following early jaw fractures (Binger 1989). The fact that load transmission may no longer be possible in this area after fracture is irrelevant for BOI because the load-transmitting surfaces are located in the protected environment of the basal cortical bone structure anyway. Furthermore, our implantation experiment under non-sterile conditions suggests that infections cannot be caught in the bone around freshly inserted BOI implants. Further studies are needed to learn more about this phenomenon. Crestal implants, by contrast, lack any lateral load-transmitting segments, so that their vertical components, which feature an enlarged surface for load transmission, will quickly be populated by microorganisms in the wake of mobility or mobilizing fractures (Tolman and Keller 1991). For this reason, the probability of survival of crestal implants in fractured jaws is greatly reduced.
Robert et al. (1984) reported a higher incidence of bone fractures after immediate loading of enossal titanium implants than after unloaded healing in femurs of rabbits. In these experiments, two implants each were introduced longitudinally along the femur of each rabbit and pushed apart with a compression spring. All fractures occurred within the first 7 days; no fractures were observed after the healing phase had been completed. Accordingly, the spread of microcracks after surgical stress might be an important reason for the loss of primary implant stability. The microcracks in the experimental set-up used by Robert et al. were propagated by the springs to such a large extent that spontaneous fractures occurred quickly even when the animals were only exposed to normal functional stresses.
The fractures observed in our own study, which occurred within 6–8weeks of implantation, would rather suggest that increasing porosity induced by remodelling will even spread to more remote jaw areas, while a reactive increase of mineralization renders areas that have never been injured increasingly brittle, such that they eventually fracture (Scully and Bestermann 1982). The large differences between the histological sections of one implant demonstrate that the degree of mineralization may vary greatly even within the same jaw area and around the same implant.
23.1.5.2 Clinical Application – Implant Procedures in Infected Areas and Periodontally Involved Regions (see Chapter 8 for Details)
In our view, the successful integration of BOI implants that were contaminated at the time of insertion can be attributed to the fact that infections can quickly suppurate through the wide horizontal and vertical insertion slot. Crestal implant fixtures, by contrast, will close the drainage pathway like a cork in a bottle.
Placement of sterile screw implants may work however in periodontally involved jaws , as long as the osteotomy area is keep clean enough. The healing in periodontally infected sites was slower initially, reaching levels of the non diseased sites after 12 weeks (Marcaccini et al. 2003).
23.1.5.3 Clinical Application – Morphological Changes of the Jawbone After Immediate Loading
Goodship et al. (1979) demonstrated that the first reaction to occur after surgical separation of the ulna in pigs was substantial formation of woven bone on the opposite side of the missing ulna. Only 3 months later, these structures had resorbed, while new woven bone had formed on the side of the missing ulna instead. What had happened was an attempt of the remaining bone structure to adapt to the situation by replacing the missing ulna in terms of volume and spatial position.
The following conclusions can be drawn from this experiment:
-
If considerable tensile stresses are present on the side of the bone with increasing convexity, woven bone is deposited in that area very quickly. This initial reaction originates from the periosteum. We observed similar reactions with BOI implants in dogs’ mandibles.
-
However, in Goodship’s experiment, after 3 months the dissected bone was replaced on the side that had grown more convex as a result of load and function. This finding was consistent with Frost’s flexural neutralization theory but could not be confirmed in the dogs’ mandibles, in which modelling had occurred exclusively on the lingual side, and the bone gained there seemed to have stabilized.
-
The immediately loaded crestal implants were associated with both lingual and vestibular remodelling at the end of the observation period.
-
While the duration of these experiments was too short to establish whether the newly modelled bone was going to be preserved in the long run, there are some indications that this was going to be the case both with the crestal implants and with the BOI implants. After all, the modelling zones that were still observed by Goodship et al. within a few weeks of the surgical procedure on the concave side were once again resorbed after 3 months. The fact that modelling zones in the mandible can be extremely stable is apparent from the high prevalence of bony outgrowths (tori mandibularis) in clinical practice.
-
Clinically, the following can be seen with failing BOI implants: If a BOI is no longer integrated due to an infection reaching down to the basal plate, lingual bone apposition similar to the formation of a mandibular torus can be observed. This formation of bone is proof of changes in the 0–1 properties inside the mandible, and it indicates that the implant prevents the regular formation of osteons and regular load transmission. The implicated BOI implants usually have to be replaced.
23.1.6 Conclusions
Immediate loading of implants will result in propagation of microcracks induced by the implantation procedure. Crestal implant fixtures will induce reactive modelling on the lingual and vestibular side of the mandible by their vertical notch effect. BOI implants that have been placed through a vestibular insertion path, by contrast, only involve a lingually oriented notch effect.
Crestal implant fixtures that are not osseointegrated will not involve any frictional adhesion at the implant-bone interface, and crack propagation cannot be demonstrated. Without immediate loading, compensational modelling does not even take place when very bulky (relative to the size of the jaw) crestal fixtures are inserted and the vestibular bone volume is additionally reduced. BOI implants are relatively resistant to any infections present within the bone or introduced during the procedure. Both the prosthetic concept and the concept of immediate loading will influence the long-term morphology of the bone structures around enossal implants. The development and propagation of microcracks provide information on whether and to what extent enossal implants were immediately loaded in animal experiments.
Immediate loading of crestal and basal implants in dogs’ mandibles will give rise to lateral bone apposition. Crestal implants that are allowed to heal in a non-loaded state are not associated with bone apposition. Appositional bone modelling is also absent if osseointegration of crestal implants is prevented by infection.
In contrast to the developments seen with the crestal implants in our study, non-sterile insertion of BOI implants did not prevent their osseointegration even when immediate loading was used. Appositional modelling as observed after sterile implantation procedures was, however, not observed.
We attempted to create conditions under which osseointegration of BOI implants would fail by selecting osteomyelitic areas or dental roots as insertion sites, or by inserting extremely dirty and infected implants, or by combining these adverse factors. None of these attempts, however, prevented the successful integration of BOI implants in this study. Note, however, that some of these experiments were performed with smooth implant surfaces while all screw implants that were inserted under non-sterile conditions featured enossal load-transmitting surfaces that had been created through sandblasting.
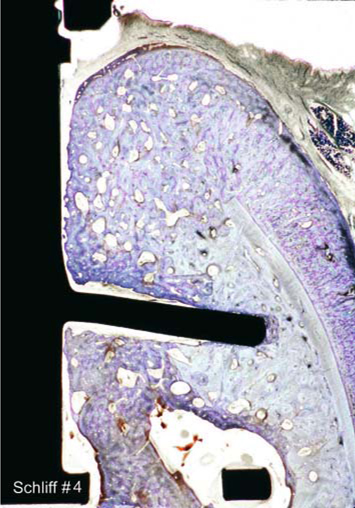
Fig. 23.9.
Double-disk BOI implant (both the threaded pin and the crestal disk are visible) with a crown block restoration. The old cortical bone line (small black arrows) is clearly visible. To the right, there is newly formed bone (dark blue). Lingually, there is new formation of woven bone. The connective tissue in the shaft area extends below the crown, and there is no epithelial growth in a caudal direction. The soft-tissue structures around BOI implants do not seem to have a layered structure (biological width) like the one described with crestal implants. The implant has been treated by shot-peening for a smooth uniform surface. The implant had not been inserted in an optimally centric position. The specimen does reveal, however, that the crestal plate had penetrated the lingual cortical bone structure at one specific location. The load-transmitting area of the crestal plate has been optimally osseointegrated (red arrow). Extensive remodelling is observed above the crestal disk and vestibularly to the threaded pin. The basal ring segment visible in this picture came to be located in a medullary space (green arrow)
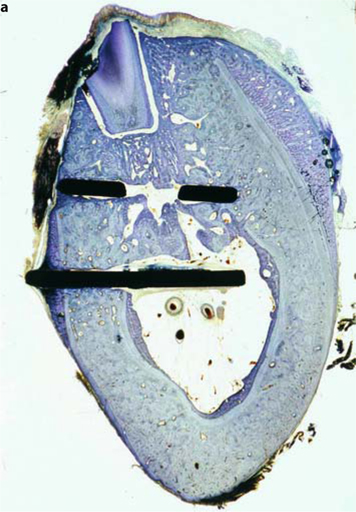
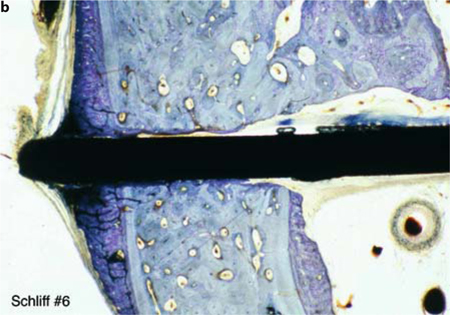
Fig. 23.10a,b.
Bone apposition of around 1.5 mm is also seen on the lingual aspect (right) at a distance of around 3 mm from the threaded pin. The old cortical bone line had almost completely dissolved by that time. Close osseoadaptation is present from above the basal disk down to the medullary compartment of the mandible. There are no indications that osseointegration is impaired in any way by the residual root structure. Perfectly inflammation-free medullary space with endosteal new bone formation. The “tent effect” gives rise to new bone formation below the periosteum of the slightly projecting basal plate (b). The basal plate, although located in the bone-free medullary space, is overgrown with a thin layer of new bone from a crestal direction. The residual root structure only affects the integration of the load-transmitting surface to the extent that it is able to influence the direction of remodelling. Sterile insertion at a disk height of 0.7 mm
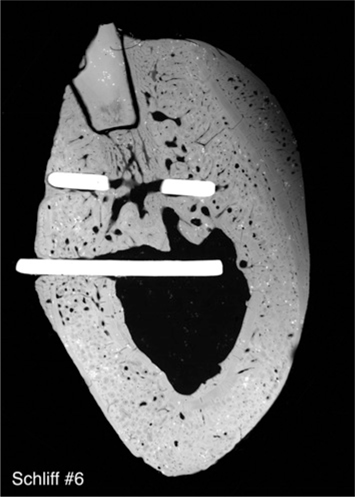
Fig. 23.11.
Radio contrast image illustrating a caudovestibular increase of bone mineralization. The subperiosteally formed bone structure (top right) is still undermineralized. The partially remodelled bone between the vestibular disk segments (on the insertion side) reveals bright cores of higher mineralization
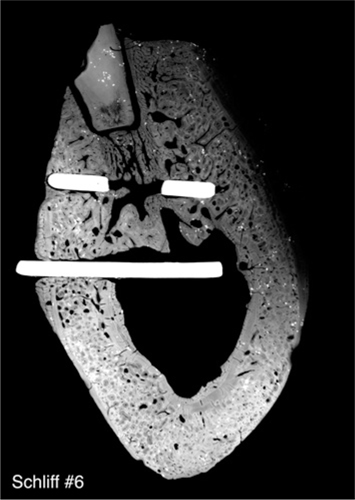
Fig. 23.12.
On lowering the radiation intensity, differences density become apparent in the basal jaw segment as well
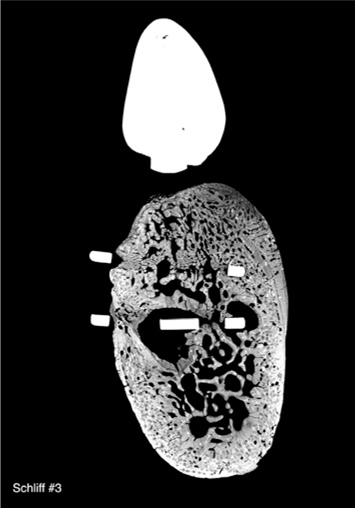
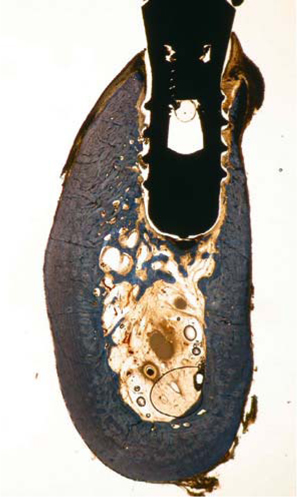
Fig. 23.14.
A non-osseointegrated implant that was immediately loaded after insertion (dog 2, right side). No forces are transmitted directly to the bone (implant core diameter: 3.6 mm). No additive formation of new bone of periosteal origin is visible. Instead, almost the entire jawbone, which had previously been characterized by inflammatory resorption, was newly established as woven bone. The histological appearance is consistent with a post-osteomyelitic state. Connective-tissue residues of inflammation are still visible in the medullary space
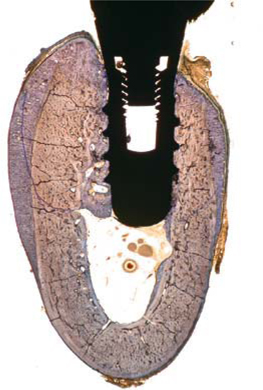
Fig. 23.15.
Osseointegrated screw implant that was immediately loaded after insertion. The formation of new bone on the lingual and vestibular sides is clearly visible. The bone structure around the thread was extensively remodelled as well (blue areas). The core diameter was 3.6 mm. The newly modelled and remodelled bone structures can be identified by their blue colour. Extensive remodelling only took place along the vertical implant axis on the vestibular side. On the lingual side, the pre-existing bone structure is still present, almost unchanged. A moderate amount of endosteal bone apposition is present as well. The vestibulo-crestal bone structure was remodelled in its entirety
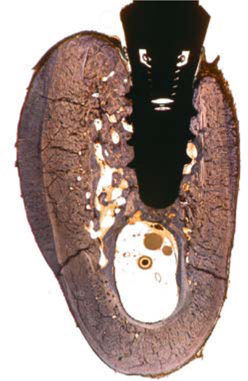
Fig. 23.16.
Bone modelling will spread to non-restored jaw areas on the immediately loaded side in the immediate vicinity of the loaded implants. Unloaded implants, by contrast, reveal smaller amounts of remodelled bone along the load-transmitting interface. The darker lines visible in this picture are true fracture lines because they are not directly in touch with the implant. The fractures in this area had already been eliminated by remodelling of the bone structure immediately surrounding the implant (clearly visible by its distinct colour), among other factors. This implant, too, reveals much more extensive remodelling on the vestibular than on the lingual side of the vertical implant axis
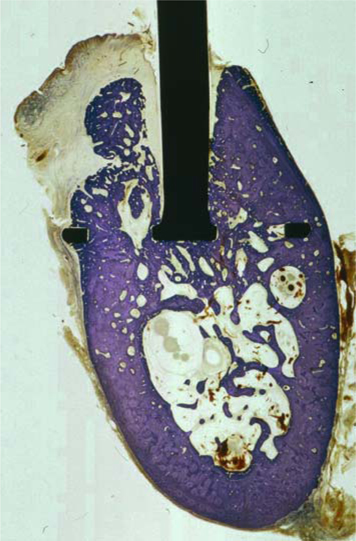
Fig. 23.17.
This BOI implant is well osseointegrated along its basal ring and lower portion of its threaded pin at 4 months although it had been inserted in a contaminated state under non-sterile conditions followed by immediate loading. Tiny residues of infection are still visible in the medullary space. All visible cortical areas were rebuilt with plexiform bone. The formation of new bone in the area of the threaded pin appeared to be delayed in comparison with implants that had been inserted under sterile conditions. In contrast to the specimen depicted in Fig. 23.27, which was collected after the same duration of service, remodelling of the woven bone structures and any missing areas has already been initiated at many sites. New formation of trajectorially oriented trabeculae is also under way.
The more pronounced invagination of soft-tissue segments in the vertical osteotomy slot indicates that connective-tissue structures that had advanced into the area first are gradually being displaced by the newly formed bone structures. This implant features a surface-enlarged apical portion of the threaded pin, which is already characterized by close osseoadaptation and osseointegration in this picture. The lateral insertion slots are largely filled with bone as well. Once again, the large amounts of woven bone (dark bone on the margin of the specimen) are indicative of a post-osteomyelitic state
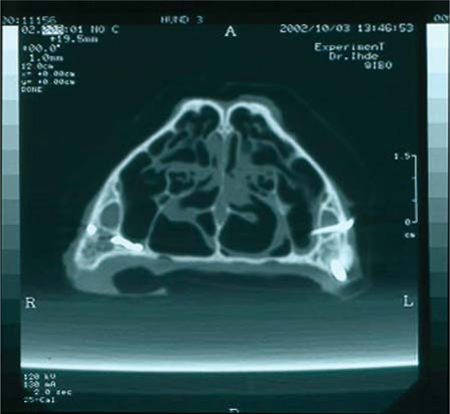
Fig. 23.18.
CT overview obtained 4 months after insertion. The attempt to induce mucosal alterations or chronic inflammation by inserting a contaminated implant into the maxillary sinus was not successful
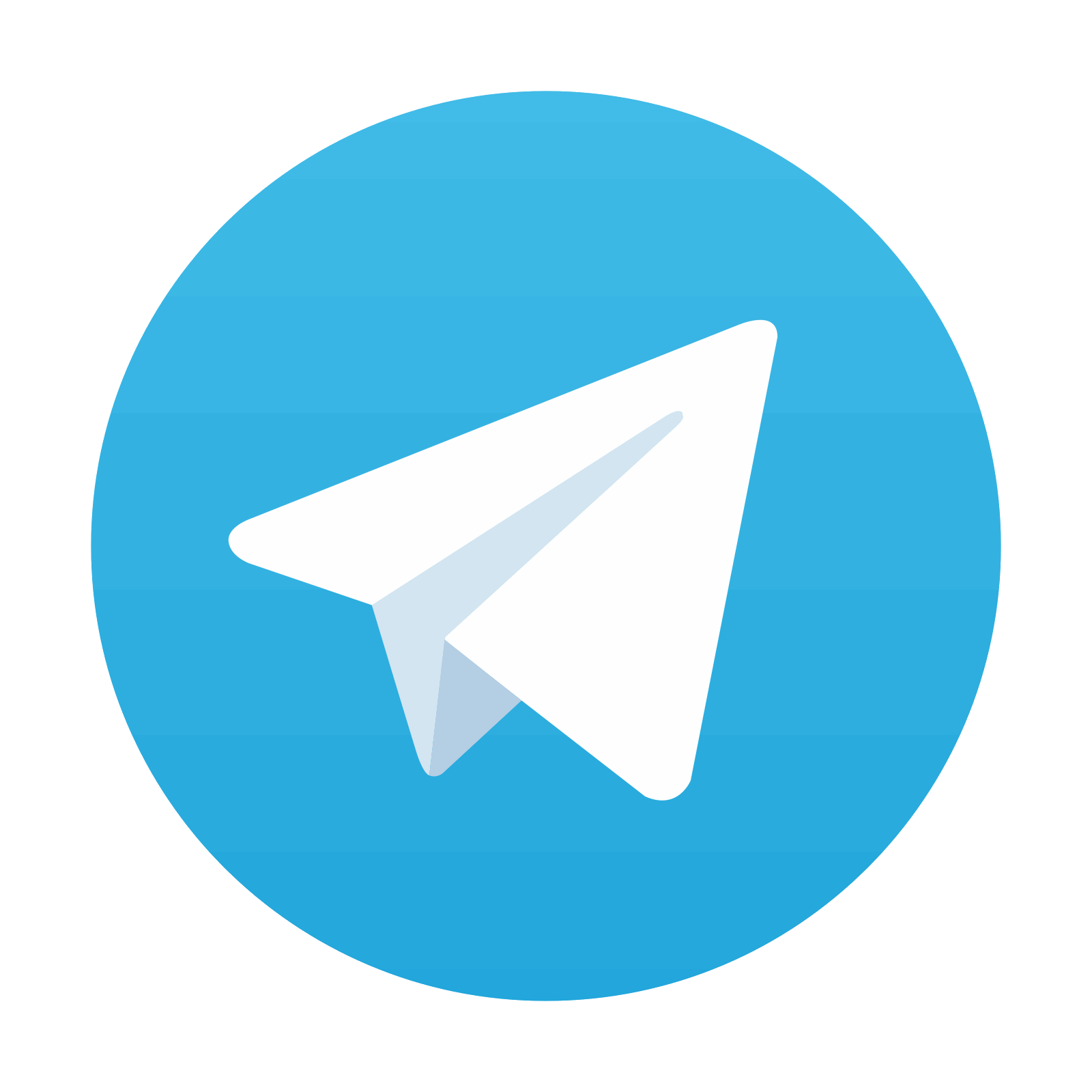
Stay updated, free dental videos. Join our Telegram channel

VIDEdental - Online dental courses
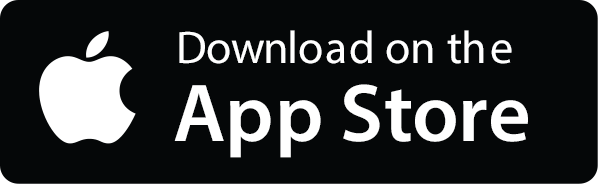
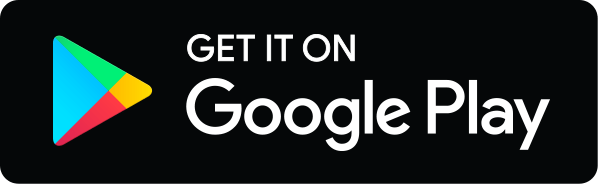