Abstract
Objective
Development of high strength dental composites with adhesive, antibacterial and re-mineralizing potential.
Materials
Urethane and triethylene glycol dimethacrylates were combined with HEMA (10 or 20 wt%) and 2MP (2 or 10 wt%), antibacterial chlorhexidine (2.5 wt%) and chemical cure initiators. Reactive mono/tri calcium phosphate (CP) mixed with silica/silicon carbide nanoparticles (S) (CP:S weight ratio 1:2 or 2:1) was added (50 wt%).
Results
Decreasing CP/S ratio and HEMA content reduced monomer conversion at 15 min from 93 to 63%. Conversely, decreasing CP/S increased initial “dry” compressive (137–203 MPa) and flexural (79–116 MPa) strength. With high HEMA content, these decreased by ∼15–20 MPa upon 24 h water storage. With low HEMA content, average decline was <8 MPa due to reduced water sorption. Early water sorption induced mass increase, volume expansion, mono calcium phosphate dissolution and chlorhexidine release, were proportional to the initial calcium phosphate content. Furthermore, they increased ∼1.5 fold upon raising HEMA wt%. These diffusion controlled processes and strength decline slowed after 24 h as phosphates reaction bound water within the materials. Increasing 2MP concentration reduced calcium release but did not affect strength. Formulations with high CP/S indicated greater antibacterial activity in agar diffusion and in vitro biofilm tests.
Significance
New material use beneath a conventional composite could potentially reduce high failure rates associated with residual caries and bacterial microleakage.
1
Introduction
Dental caries involves acid-producing bacteria that enhance dissolution of hydroxyapatite from both enamel and dentin. In dentin, remaining collagen is subsequently degraded by matrix metalloproteinase enzymes (MMPs) . In order to arrest disease progression and restore shape and function, damaged dental structures were previously replaced by amalgam but this is increasingly being replaced by more esthetic composite materials . The use of adhesives with dental composites has reduced the need for over cutting and sound tooth removal procedures previously adopted to ensure amalgam retention . Rapid bond strength deterioration upon thermal or mechanical cycling, however, is a well known problem . Moreover, composites shrink upon polymerization. The resultant stress can enhance tooth adhesion loss, microgap formation and ultimately bacterial microleakage . This, in combination with lack of antibacterial action, can result in current composite resin restorations having a greater risk of secondary caries and being replaced at a higher rate than amalgam .
To overcome bacterial microleakage, chlorhexidine (CHX) has previously been added into dental composites . In addition to being antibacterial, this drug can inhibit MMP action . Composites with early release of chlorhexidine might reduce the need for extensive disease affected tissue removal as advocated in modern tooth restoration procedures . Unfortunately, CHX is not readily released from the bulk of a conventional composite. This problem has been overcome through partial replacement of hydrophobic composite monomers with the hydrophilic monomer, hydroxyethylmethacrylate (HEMA). Hyrophilicity enhances water sorption and expansion which counteracts polymerization shrinkage. It also increases early drug release and antibacterial action . Unfortunately, strength is reduced.
An alternative method employed to reduce bacterial microleakage with composite use has been addition of amorphous calcium phosphate. It has been proposed that release of calcium phosphate may help re-mineralization of surrounding dentin . Poor initial strength, however, was common due to lack of any bonding mechanism between the filler and matrix phase .
Additionally, reactive acidic and basic mono and tri calcium phosphate fillers (MCPM/β-TCP) have been included in dental composites . Use of the hydrophilic and soluble MCPM alone caused rapid water sorption, calcium phosphate release and decline in strength. When β-TCP was added, MCPM in the surface of the material still dissolved. MCPM in the bulk, however, reacted with the β-TCP binding water in brushite (dicalcium phosphate dihydrate) crystals.
MCPM/β-TCP fillers and chlorhexidine were previously incorporated in urethane (UDMA)/triethylene glycol (TEGDMA)/hydroxyethyl (HEMA) di and mono methacrylate resins . The combined presence of high levels of hydrophilic MCPM (25–38 wt% of composite) and HEMA (50 wt% of resin) enabled much higher CHX release than generally possible with dental composites . It also, however, caused excessive water sorption, swelling and early strength decline.
The following new study aim is to address if reduction in HEMA level (10–20 wt%) can control these reactive filler composite problems without overly inhibiting release of chlorhexidine. Furthermore, the reactive calcium phosphate fillers are partially replaced with silica/silicon carbide particles in an attempt to improve early strengths whilst maintaining some calcium phosphate release. Additionally, the calcium binding monomer (Bis[2-(methacryloyloxy)ethyl] phosphate (2MP)) is added to assess if this can provide a bonding mechanism between the calcium phosphate fillers and monomer, thereby raising strength.
2
Materials and methods
2.1
Sample preparation
2.1.1
Filler
β-TCP (β-tricalcium phosphate) (Fluka, Germany) of particle diameter < 15 μm was used without modification. MCPM (monocalcium phosphate monohydrate) (Fluka, Germany) particles were ground by means of a ball mill. To obtain MCPM particles of 20–38 μm diameter (median 29 μm), the ground powder was sieved through Endecotts sieves (Laboratory Sieve, UK).
Silica nanoparticles (Sigma–Aldrich, UK) (diameter 10–20 nm) were mixed with an equal mass of silicon carbide nanoparticles (Sigma–Aldrich, UK) (diameter < 100 nm). Mixing was undertaken in ethanol (BDH, UK) using a magnetic stirrer bar and hot plate (Heidolph Stirrer, UK). Samples were stirred in a fume hood until dry. The dried mixture was subsequently heated in an oven (Lenton Thermal Design Ltd., UK) at 800 °C for 30 min. After cooling, the silica–silicon carbide was reacted with 4% 3-methacryloxypropyltrimethoxysilane (MPS) (Aldrich–Sigma, UK) by stirring for 1 h at room temperature in ethanol. The silanized mixture was subsequently allowed to dry for 1 h at room temperature and then at 37 °C for 24 h. Using a ball mill, the silanized nano-silica–silicon carbide powder was finally ground to obtain particles in the range 20–38 μm.
2.1.2
Accelerator and initiator containing pastes
The composite resin consisted of UDMA (Rhom, Germany) base monomer: TEGDMA diluent (Sigma–Aldrich, UK) (1:1 weight ratio). This provided fluid monomers that could readily mix with other components and cure well with chemical initiators. HEMA (Sigma–Aldrich, UK) was added at 10 or 20 wt% as previous work suggests this should provide measurably different but not excessive levels of water sorption . Bis[2-(methacryloyloxy)ethyl] phosphate (2MP) (Polyscience, USA) was added at lower levels (2 or 10 wt% of resin). These levels would be sufficient to provide a thin surface layer around any calcium phosphate particles without monomer phase separation. Chlorhexidine diacetate (Sigma–Aldrich, UK) was fixed at 2.5 wt% of the resin. This ensured most of the chlorhexidine was dissolved in the monomer phase but release was sufficient for ready quantification by UV. Either N,N-dimethyl-p-toluidine accelerator (DMPT) (Sigma–Aldrich, UK) (1 wt%) or benzoyl peroxide (BP) initiator (Sigma–Aldrich, UK) (2 wt%) with 2,6-di-tert-butyl-4-methyl phenol inhibitor (BMP) (Fluka, Spain) (0.05 wt%) was subsequently included. Preliminary investigations indicated this gave stable liquids that could cure in a short period after mixing.
Filler was added at a level of 50 wt% of the total mass to produce separate initiator and activator pastes. 50 wt% was the maximum filler loading that gave pastes of reasonable consistency. The filler consisted of MCPM and β-TCP (CP) in equal masses combined with silica–silicon carbide particles (S). CP:S weight ratio was 2:1 or 1:2. Preliminary calculations and earlier work suggested these levels should help ensure sufficient water sorption to balance polymerization shrinkage.
2.1.3
Sample discs
Equal mass fractions of initiator and activator paste were blended and placed into suitable molds to obtain discs/cylinders of different dimensions. Circular discs, 10 mm diameter and 2 mm thick were prepared for biaxial flexure strength (BFS) tests. 4 mm diameter and 6 mm long cylinders were prepared for compressive strength (CS) tests. For water sorption, CHX release, ion release and agar diffusion studies 10 mm diameter discs of 1 mm thickness were used. For the bacterial biofilm studies 5 mm diameter, 1 mm thick samples were required. The discs/cylinders were covered by acetate sheet and a microscope slide, which was slightly pressed to remove excess material. After hardening (6 ± 0.6 min) (mean ± 95% CI), the samples were removed from the molds and stored at room temperature (23 °C) until usage.
2.1.4
Formulations used
In Fourier Transform Infrared (FTIR), strength, gravimetric, calcium phosphate and chlorhexidine release studies a two level factorial experimental design was used and 8 samples with all possible combinations of HEMA level, 2MP content and CP/S prepared (see Table 1 ). For X-ray diffraction (XRD) investigations a formulation containing 2MP (2 wt%), HEMA (10 wt%) and CP/S (2:1) was used. For antibacterial work, formulations with CP/S (2:1), HEMA (20 wt%) and 2MP (10 wt%) with 0 or 2.5% CHX were compared. Furthermore, 3 formulations with CHX (2.5%) and CP/S (1:2) were investigated. These had HEMA (20 wt%) and 2MP (10 or 2 wt%) or HEMA (10 wt%) and 2MP (2 wt%). The commercial resin modified glass ionomer cement Fuji II LC (GC Corporation, Japan) was used as a control in strength and bacterial biofilm experiments.
(a) | |||||||||
---|---|---|---|---|---|---|---|---|---|
CP/S (w/w) | HEMA (wt%) | 2MP (wt%) | Monomer conversion (%) (RCI = 2%) |
Compressive strength (MPa) (RCI = 2%) |
Flexural strength (MPa) (RCI = 4%) |
||||
Dry | 24 h | 1 week | Dry | 24 h | 1 week | ||||
2 | 20 | 2 | 93 | 137 | 123 | 119 | 81 | 64 | 51 |
2 | 10 | 2 | 83 | 138 | 130 | 130 | 79 | 76 | 73 |
2 | 20 | 10 | 93 | 138 | 125 | 122 | 80 | 64 | 52 |
2 | 10 | 10 | 84 | 137 | 132 | 131 | 82 | 78 | 76 |
0.5 | 20 | 2 | 78 | 201 | 183 | 180 | 114 | 94 | 86 |
0.5 | 10 | 2 | 64 | 203 | 195 | 191 | 112 | 107 | 105 |
0.5 | 20 | 10 | 74 | 199 | 182 | 178 | 116 | 94 | 84 |
0.5 | 10 | 10 | 63 | 200 | 196 | 190 | 114 | 109 | 108 |
Geometric mean | 78 | 166 | 155 | 152 | 96 | 84 | 77 |
(b) | ||||||||
---|---|---|---|---|---|---|---|---|
CP/S (w/w) | HEMA (wt%) | 2MP (wt%) | Initial mass gradient (wt% min −0.5 ) (RCI = 9%) |
Maximum increase (RCI = 5%) |
CHX release (RCI = 10%) |
|||
Mass (%) | Volume (%) | Gradient (wt% min −0.5 ) | Maximum (%) | D. coeff. (μm 2 s −1 ) | ||||
2 | 20 | 2 | 0.033 | 2.3 | 4.7 | 0.23 | 32 | 0.017 |
2 | 10 | 2 | 0.023 | 1.5 | 3.0 | 0.14 | 21 | 0.006 |
2 | 20 | 10 | 0.032 | 2.1 | 4.4 | 0.24 | 31 | 0.019 |
2 | 10 | 10 | 0.020 | 1.6 | 2.8 | 0.14 | 23 | 0.006 |
0.5 | 20 | 2 | 0.015 | 1.1 | 1.9 | 0.10 | 18 | 0.003 |
0.5 | 10 | 2 | 0.009 | 0.8 | 1.6 | 0.08 | 11 | 0.002 |
0.5 | 20 | 10 | 0.016 | 1.0 | 1.8 | 0.10 | 18 | 0.003 |
0.5 | 10 | 10 | 0.009 | 0.8 | 1.4 | 0.06 | 10 | 0.001 |
Geometric mean | 0.018 | 1.3 | 2.5 | 0.12 | 19 | 0.005 |
2.2
Chemical analysis
2.2.1
Degree of monomer conversion
Equal masses of accelerator and initiator pastes of each formulation was mixed and loaded immediately into a metal ring mold (10 mm internal diameter and 2 mm thickness) on the center diamond of a golden gate™ heated ATR top-plate (Specac Ltd., UK) in an FTIR spectrometer (Perkin Elmer Series 2000, UK). The temperature was kept at 37 °C using a 3000 Series RS232 high stability temperature controller (Specac Ltd., UK). The top surface of the sample was covered with acetate sheet to prevent oxygen inhibition of the polymerization process. FTIR spectra of the sample in contact with the diamond at 20 s and 15 min after the start of mixing were obtained with resolution set at 4 cm −1 , wavenumber range between 600 and 2000 cm −1 and scan number of 8. Degree of monomer conversion was calculated using
% degree of conversion = 100 [ A 20 s − A 15 min ] A 20 s
A t is the height of the monomer peak at 1320 cm −1 (C O bond stretch) above background at 1335 cm −1 at time t . Experiments were performed in triplicate.
2.2.2
X-ray diffraction (XRD)
An X-ray diffractometer (Brüker D8 Advance Diffractometer, Germany) was used to identify water sorption induced chemical changes of calcium phosphate fillers within polymerized composite formulations after placement in water for 24 h. The data was collected over a 2 range of 10–100° with a step size of 0.019° and count time of 18.9 s, using Ni filtered Cu Kα radiation (wavelength = 1.5406 A). The International Center for Diffraction Database and crystallographica search match software (Oxford Cryosystems, UK) were used to identify calcium phosphate species.
2.3
Compressive (CS) and biaxial flexure strength (BFS)
BFS and CS of the composite formulations, before and after 24 h and 1 week of storage in deionized water, were measured by means of a computer-controlled universal testing machine (Instron 4502, UK) using a crosshead speed of 1 mm/min ( n = 8). For BFS studies, a knife-edge ring support of 8 mm diameter and 4 mm diameter spherical ball indenter were used . BFS was determined using Eq. (2) :
where ρ refers to load measured at fracture, ν is the Poisson’s ratio (0.225 for composite resin ), a and l are the radius of the circular support and the specimen thickness, respectively.
CS was calculated using Eq. (3) :
r is the specimen radius.
2.4
Water sorption and component release
2.4.1
Mass and volume changes
The mass and volume of composite formulations were gravimetrically determined using a density kit and four-figure digital balance (Mettler Toledo). Each test sample was stored in separate sterilin tubes each containing 10 ml of deionized water at 37 °C. After 1, 2, 3, 4, 6, 24, 48 h and 1, 2, 3, 4, 5, 6 weeks specimens were removed, blotted dry, reweighed and replaced in new tubes containing fresh storage solution. The percentage volume and mass change were determined using
V t and M t are the volume and mass at time t after immersion, while V o and M o are the initial volume and mass, respectively. Results were obtained in triplicate.
2.4.2
Chlorhexidine release
Absorbance between 200 and 400 nm (resolution 1 nm) of all storage solutions from mass and volume change studies was obtained at each time point above using a UV spectrometer (Unicam UV 500, Thermo-Spectronic ® , UK). Results were compared with standard spectra from 0.001 to 0.004 wt% solutions of CHX to ensure that the drug was the only component giving significant absorbance at 255 nm. Storage solution absorbance at 255 nm was then used to quantify CHX concentration in each solution. This was converted to grams. To gain cumulative CHX release at each time point, as a percentage of that in the sample, the number of grams in each solution up to the time point was summed .
2.4.3
Ion release
For calcium (Ca 2+ ) and phosphate (PO 4 3− ) release studies, separate specimens ( n = 3) were stored at 37 °C in 10 ml deionized water. Storage solutions were replaced and analyzed using ion chromatography (IC) (Dionex, UK) after 1, 2, 3, 4, 6, 24, 48 h, 1 and 2 weeks. Calibration solutions of 5, 10, 25 and 50 ppm Ca 2+ and PO 4 3− were prepared using calcium chloride (CaCl 2 ·2H 2 O, BDH, UK) and sodium phosphate (Na 3 PO 4 ) (Sigma–Aldrich, UK) and used to calculate cumulative release. Results were converted to mM for ease of comparison.
2.5
Antibacterial studies
2.5.1
Agar diffusion assay
The antibacterial activity of composite formulations against Streptococcus mutans (NCTC 10449), Lactobacillus casei (NCTC 6375) and Actinomyces naeslundii (NCTC 10951) were evaluated using an agar diffusion assay. Each bacterial strain was cultivated on isosensitest agar (Oxoid, UK) plates. After aseptic addition of three discs of each composite formulation, plates with S. mutans and L. casei were incubated at 37 °C in a 5% CO 2 atmosphere for 48 h. Those with A. naeslundii were incubated anaerobically for 72 h. The diameters of inhibition zone around the samples were measured at three different points using calipers.
2.5.2
Biofilm study
A CDFF (Constant Depth Film Fermentor; AC Service Group, Poole, UK) was used to evaluate the efficiency of different formulations at inhibiting oral bacterial biofilm growth. Nine discs of each formulation were placed in the polytetrafluoroethylene (PTFE) CDFF pans. The samples were recessed to 300 μm to allow space for biofilm growth. Thereafter, the pans were inserted flush with a stainless steel turntable (rotated at 3 rpm during experiments) beneath fixed PTFE scrapper blades. The CDFF was then autoclaved at 121 °C for 15 min.
Equal volumes of saliva collected from 10 healthy individuals were mixed with 10% glycerol (v/v) and stored at −70 °C. When required, 1 ml was defrosted and added to 500 ml of sterile artificial saliva. This inoculated saliva was first introduced into the autoclaved CDFF (at 37 °C) via a peristaltic pump using a flow rate 0.5 ml/min (approximate salivary flow rate in humans) for 8 h. Thereafter, the inoculation vessel was disconnected and a medium reservoir delivering sterile artificial saliva at the same rate, connected. After 6, 24 or 72 h from start of saliva addition, three discs of each formulation were aseptically removed. These were vortexed for 1 min in 1 ml of phosphate buffer saline (Oxoid, UK). Following serial dilution, 25 μl of each phosphate buffer solution was plated onto fastidious anaerobic agar plates (Oxoid, UK). Following anaerobic incubation at 37 °C for 5 days the number of colony-forming units (CFU) was determined using Eq. (6) :
M CFU and D F are the mean number of colony forming units of the three samples and a dilution factor, respectively.
2.6
Mathematical and statistical analysis
2.6.1
Diffusion controlled processes
Diffusion controlled properties such as water sorption, CHX, calcium and phosphate release for flat discs are often described by the general equation :
2 l , D and t are the sample thickness, a diffusion coefficient and time, respectively. Δ M and M ∞ represent the change with time and maximum change in mass and volume or cumulative component concentration in solution. In the following these properties were therefore plotted versus square root (SQRT) of time. Initial gradients and maximum or final values were determined and for CHX release a diffusion coefficient calculated.
To quantify the effects of different variables on the degree of conversion, mass and volume changes, CHX release, CS and BFS, results were fitted to a factorial expression
ln P = ln 〈 P 〉 + F 1 a 1 + F 2 a 2 + F 3 a 3 + F 1 F 2 a 12 + F 1 F 3 a 13 + F 2 F 3 a 23 + F 1 F 2 F 3 a 123
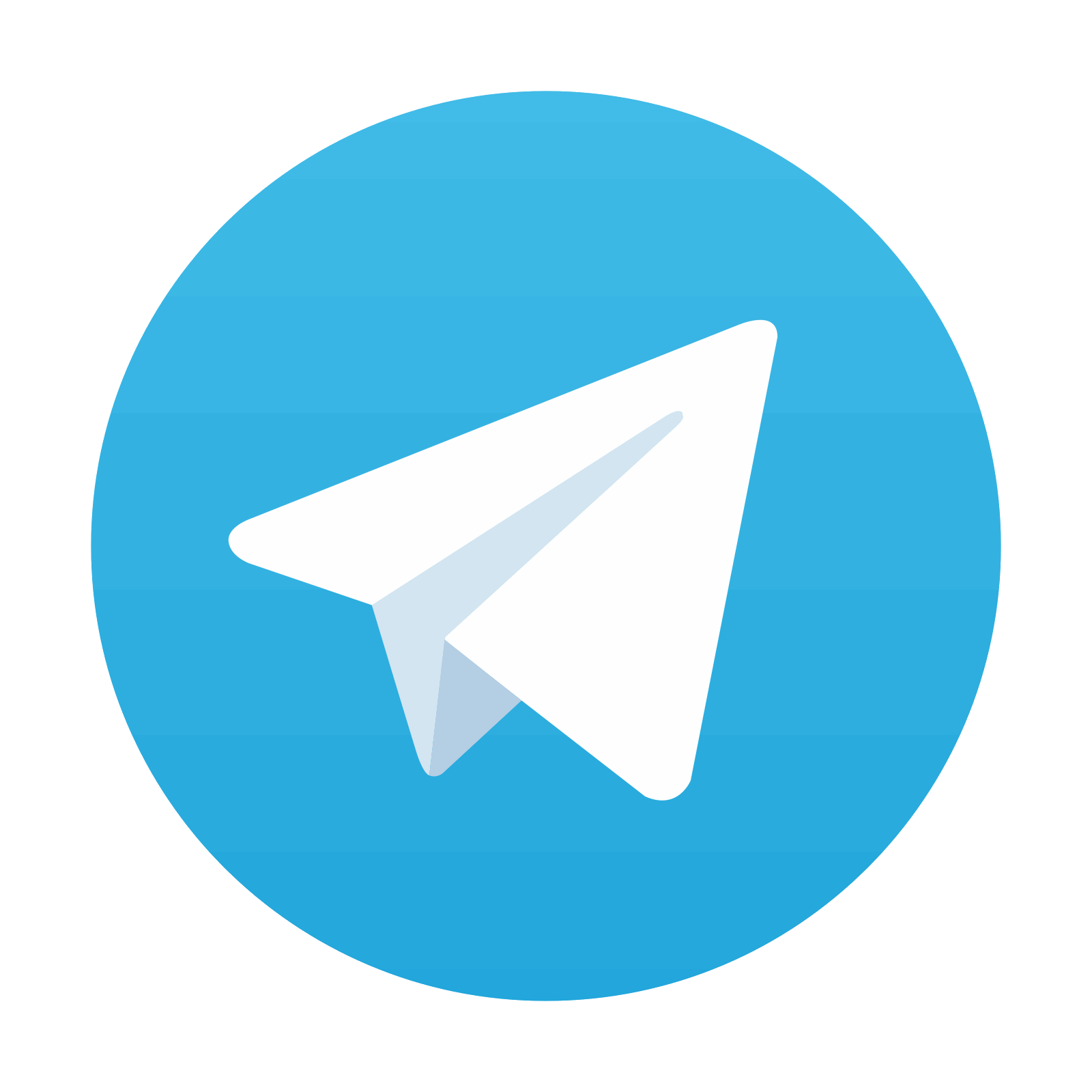
Stay updated, free dental videos. Join our Telegram channel

VIDEdental - Online dental courses
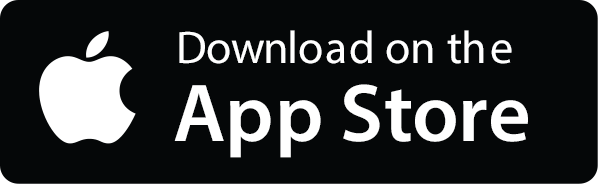
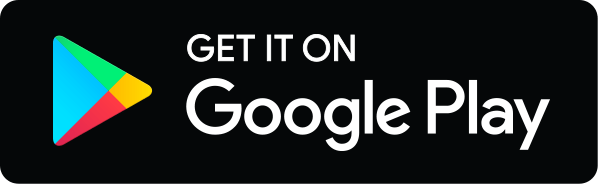