Abstract
We previously generated viable heterotopic bone in living animals and found that 3 months of intrinsic vascularization improved bone formation and matrix degeneration. In this study, we varied the pre-vascularization time to determine its effects on the kinetics of bone formation and ceramic degradation. Two 25-mm-long cylindrical β-tricalcium phosphate scaffolds were filled intraoperatively with autogenous iliac crest bone marrow and implanted in the latissimus dorsi muscle in six sheep. To examine the effect of axial perfusion, one scaffold was surgically implanted with (group C) or without (group D) a central vascular bundle. All animals were sacrificed 6 months postoperatively and histomorphometric measurements were compared to previous results. All implanted scaffolds exhibited ectopic bone growth. However, bone growth was not significantly different between the 3-month (group A, 0.191 ± 0.097 vs. group C, 0.237 ± 0.075; P = 0.345) and 6-month (group B, 0.303 ± 0.105 vs. group D, 0.365 ± 0.258; P = 0.549) pre-vascularization durations, regardless of vessel supply; early differences between surgically and extrinsically vascularized constructs disappeared after 6 months. Here, we describe a reliable procedure for generating ectopic bone in vivo. A 3-month pre-vascularization duration appears sufficient and ceramic degradation proceeds in accordance with bone generation, supporting the hypothesis of cell-mediated resorption.
In a previous study, our research group succeeded in generating heterotopic bone with a vascular pedicle by using β-tricalcium phosphate (β-TCP) scaffolds and bone marrow in experimental animals that served as natural bioreactors. Further experiments should show whether this method could be used to fabricate replacement bone in larger and perhaps even customized segments. Before taking this crucial step, it is necessary to closely assess the pre-vascularization time. Furthermore, the degradation kinetics of porous β-TCP have yet to be determined. Large animal experiments are very cost-intensive, mainly because of the per diem expenses for animal care. Therefore, ensuring that the pre-vascularization time is as short as possible, without compromising bone formation, is a major concern.
Various groups have investigated the manner in which time affects bone generation in bioartificial bone grafts. As early as 15 years ago, Schliephake and Langner prefabricated bone grafts for reconstructing mandibular defects in minipigs. They implanted a block of pyrolysed bovine bone near the mandible, pre-vascularized it for 5 months, and then successfully transplanted the newly formed bone into a critical-size defect of the mandible.
Terheyden et al. used xenogeneic bone mineral in combination with bone morphogenetic protein-7 (BMP-7) to generate ectopic bone in the latissimus dorsi region. Pre-vascularization times of 6, 12, and 24 weeks yielded no difference in bone formation.
In summary, a shorter prefabrication time appears sufficient; however, the optimal time likely varies among different animal models, matrix materials, osteogenic cells, and osteogenic substances. Therefore, it seems advisable to optimize the prefabrication time empirically for each model in order to minimize costs.
Handschel et al. reported nearly no resorption of β-TCP granules in non-loading calvarial defects in rats even after 6 months. There was also no new bone formation apparent in the defect and they therefore concluded that β-TCP is insufficiently osteoinductive in the rat model.
Von Doernberg et al. inserted blocks of porous β-TCP with different pore sizes into long-bone defects of sheep. Nearly 80% of ceramic resorption was evident 6 weeks after implantation and <5% of the ceramic remained after 24 weeks. The pore size seemed to have only a minor influence in this study. Bone ingrowth occurred rapidly in all specimens, and therefore the authors concluded that β-TCP was highly osteoconductive.
The resorption time of β-TCP ceramics can vary widely. This might be due to the complexity of the mechanism of degradation, which may involve several different processes. Discussions regarding these mechanisms are ongoing, but two main pathways have been described: (1) the resorption of calcium phosphate ceramics mainly involving solution-mediated processes and (2) cell-mediated processes involving phagocytosis by macrophages or giant multinucleated cells and resorption by osteoclasts. The primary mechanisms involved may depend upon the material properties of the calcium phosphate used.
In the present study, we aimed to examine whether an extended cultivation time leads to more viable heterotopic bone formation and whether matrix degradation is associated with the amount of newly formed bone. The findings of this study could provide important general information regarding the mechanism of β-TCP degradation.
Materials and methods
Cylinders (length 25 mm, diameter 14 mm) consisting of pure β-TCP with 60–80% porosity and a pore size of 100–500 μm were used as carriers (chronOS; Synthes, West Chester, PA, USA). The sterile cylinders were supplied with a preformed central tunnel with a diameter of 7 mm.
The animal experimental protocol received ethical approval in accordance with German federal animal welfare legislation.
Six healthy adult female German black-headed sheep with an average weight of 79.0 kg (maximum weight 85.0 kg, minimum weight 64.5 kg) were used in the study. Anaesthesia was induced via intravenous (i.v.) injection of midazolam 0.2 mg/kg and propofol 5 mg/kg, and was maintained with isoflurane delivered in 100% oxygen (1 l/min). Butorphanol (0.1 mg/kg, intramuscularly (i.m.)), carprofen (4 mg/kg subcutaneously (s.c.)), and buprenorphine (10 μg/kg, s.c.) were administered to induce analgesia. The systolic, diastolic, and mean blood pressure, electrocardiogram findings, rectal temperature, and haemoglobin oxygen saturation were monitored continuously during surgery.
Three bone biopsies (5 mm in diameter) were harvested from the iliac crest and morselized with an electric bone mill. The morselized bone was mixed with amorphous bone marrow aspirated from deep biopsy sites, and this osteogenic material was loaded into the central cavities of the predrilled blood-soaked cylinders. Each sheep received two cylinders, which were implanted in the same muscle. The first cylinder (group C) was implanted directly into the latissimus dorsi muscle after cell loading, using the whole animal as a natural bioreactor ( Fig. 1 ). In order to determine the effect of enhanced perfusion, the second cylinder (group D) was also implanted with a central vascular bundle, which was surgically prepared by using perforating vessels from the thoracodorsal trunk ( Fig. 2 ).


Six months postoperatively, all animals were euthanized after deep sedation (midazolam 1 mg/kg, i.m., propofol 5 mg/kg, i.v., and pentobarbital 80 mg/kg, i.v.). After explantation from the latissimus dorsi muscle, the cylinders were fixed in 3.5% neutral buffered formalin, embedded in methylmethacrylate, and sectioned perpendicular to the axis of the cylinder using a modified inner-hole diamond saw. Non-decalcified slices with a thickness of 30 μm were surface-stained with alizarin–methylene blue for standard light microscopy and histomorphometric analysis. Digital images of each slide were obtained using a Zeiss Axio Imager microscope fitted with an AxioCam MRc digital camera and AxioVision 4.5 software (Carl Zeiss, Oberkochen, Germany). The AxioVision module MosaiX was used to create images of entire cross-sections of the cylinders. The total bone area and residual ceramic area were quantified using the image analysis software analySIS 3.0 (Olympus Soft Imaging Solutions, Muenster, Germany). Six sections distributed evenly throughout each cylinder were used for histomorphometric evaluations.
Statistical analysis
The impact of axial perfusion on bone growth and ceramic resorption within the cylinders was examined by Student’s t -test. The data from the previous experiment (3-month pre-vascularization period) were re-evaluated in parallel. For further analysis, cylinders without and with a vessel bundle from the previous experiment were designated group A and group B, respectively. The influence of time was evaluated by Student’s t -test. To gain a better understanding of the degradation kinetics, a non-linear regression curve was fitted to the data. The probability level was based on the adjusted P -values; P < 0.05 was considered statistically significant. The statistical software SigmaPlot 10.0 (Systat Software, Inc., San Jose, CA, USA) was used for all data analyses.
Results
All animals survived the surgery without any incident, and no complications were observed during postoperative recovery. All animals resumed ingestion and rumination immediately after surgery. The swelling of the thoracic wall resolved completely within 1 week. The implantation site was examined daily until each animal showed no signs of infection or rejection. Thereafter the animals were sacrificed. When the constructs were explanted and split into halves, the ends of the cut surfaces bled and were solid; this provided macroscopic evidence of the presence of viable bone formation within the cylinders. The implanted vascular bundle in group D could easily be detected by expressing blood from the surrounding soft tissue. Microscopically, viable bone formation was observed inside the cylinders, and primarily filled their centres. Osteogenic activity was indicated by the presence of osteoblasts and osteoid seams aligned along the mineralized surfaces. However, the trabecular bone structure was penetrated by different amounts of fibrous tissue.
Ceramic resorption and transformation into bone was also observed in isolation from the inner, osteogenic material-filled cavity. The remaining pore spaces were completely filled with highly vascularized fibrous tissue ( Fig. 3 ).

According to the histomorphometric analysis, the pre-vascularization time (3 vs. 6 months) did not significantly affect ceramic resorption in the presence of a central vascular bundle ( P = 0.060). Bone formation was also unaffected ( P = 0.345 without the vessel bundle and P = 0.549 with the bundle). There was also no significant difference in bone formation between groups C and D ( P = 0.288), although the degree of ceramic resorption was significantly greater in group D ( P = 0.009) ( Tables 1–3 and Fig. 4 ).
Group | Ø relative area bone | Ø relative area ceramic |
---|---|---|
C (6 months without a vessel) | 0.237 ± 0.075 | 0.279 ± 0.074 |
D (6 months with a vessel) | 0.365 ± 0.258 | 0.144 ± 0.105 |
P -value | 0.288 | 0.009 * |
Group | Ø relative bone area | Group | Ø relative bone area |
---|---|---|---|
A (3 months without a vessel) | 0.191 ± 0.097 | B (3 months with a vessel) | 0.303 ± 0.105 |
C (6 months without a vessel) | 0.237 ± 0.075 | D (6 months with a vessel) | 0.365 ± 0.258 |
P -value | 0.345 | P -value | 0.549 |
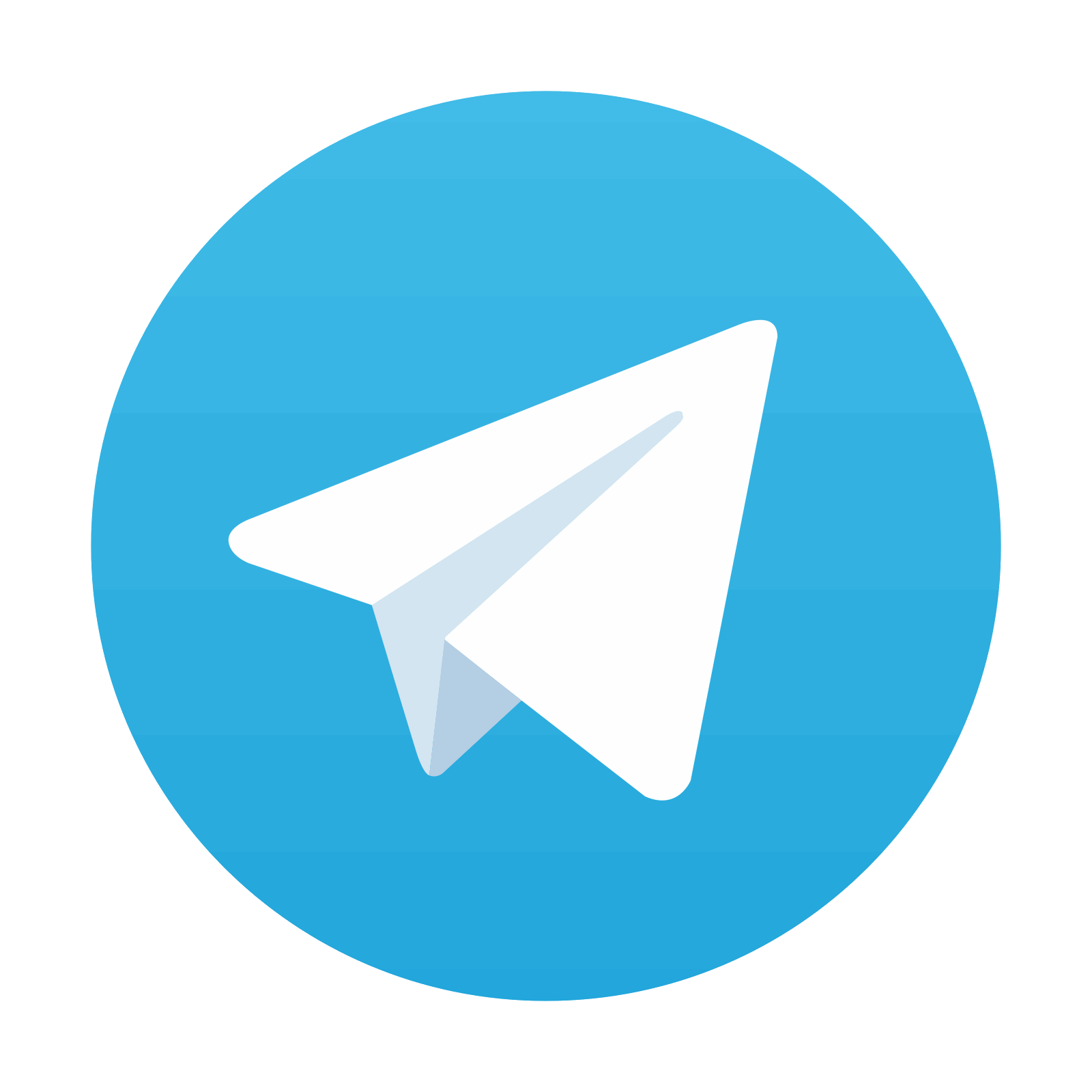
Stay updated, free dental videos. Join our Telegram channel

VIDEdental - Online dental courses
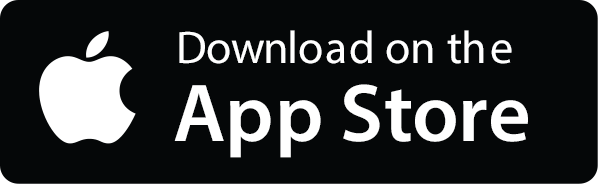
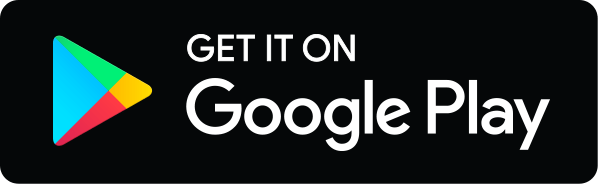
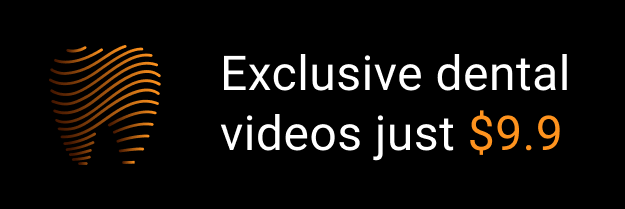