17
Hematologic Diseases
Vidya Sankar, DMD, MHS
Alessandro Villa, DDS, PhD, MPH
HEMATOPOIESIS
Hematopoiesis is the process of production of blood cells and platelets, which continues throughout life. This lifelong supply is derived from a rare population of multipotent hematopoietic stem cells (HSCs). Hematopoiesis has long been thought of as a hierarchal linear process where multi‐, oligo‐, and unipotent progenitors develop into mature hematopoietic cells. In the older model, long‐term hematopoietic stem cells (LT‐HSCs) located in the adult bone marrow are pluripotent, can self‐replicate, and are the progenitors from which all blood cell lineages arise. They are usually quiescent, but they become activated when exposed to a stress stimulus. Short‐term HSCs (ST‐HSCs) are derived from LT‐HSCs, can rapidly restore the hematopoietic system, and differentiate into multipotent progenitors (MMPs) with no detectible self‐renewal ability. MMPs further differentiate into lineage‐committed oligopotent progenitors: the common lymphoid progenitor (CLP) that only possesses lymphoid‐restricted ability, and the common myeloid progenitor (CMP), which further differentiates into megakaryocyte–erythrocyte progenitor (MEP) and granulocyte–monocyte progenitor (GMP). MEPs give rise to megakaryocytes that mature to platelets, erythroid cells that mature to red blood cells (RBCs), and GMPs that give rise to eosinophils, neutrophils, basophils, and macrophages. CLPs give rise to B lymphocytes, T lymphocytes, and natural killer and dendritic cells.1
It is now acknowledged through single‐cell transcriptome, epigenetic, and transplantation analysis, along with barcoding and in vitro clonal functional assays, that the process of lineage commitment is not strictly unidirectional, but more plastic and flexible, and lacks boundaries at the different hierarchical levels.2 In the revised hierarchical roadmap, the MMPs are divided into four subgroups (MMP1, MMP2, MMP3, and MMP4/LMPP). LT‐HSCs, intermediate‐term HSCS (IT‐HSCs), and ST‐HSC/MMP1s give rise to MMP2, MMP3, and MMP4/lymphoid‐primed multipotent progenitors (LMPPs). MMP2 and MMP3 mainly give rise to CMPs and MMP4/LMPP predominantly generate lymphoid lineages. The CMPs produce mature hematopoietic cells similar to the MEPs and GMPs of the old model, and the CLPs yield mature lymphocytes. Megakaryocyte differentiation can bypass the states of MMPs, CMPs, and MEPs coming directly from the HSCs (Figure 17‐1).1
Hematopoiesis is continuous, adaptive, and capable of responding to metabolic, infectious, and inflammatory challenges. Perturbations of the system lead to disease. Regulation of hematopoiesis is complex and only partially understood. It entails signaling through external factors such as cytokines and intracellular factors such as transcriptional signaling factors and microRNAs.3 While LT‐HSCs undergo several intermediate steps to produce mature blood cells, megakaryocyte lineage segregation occurs early during differentiation. The primary cytokines governing erythrocyte, platelet, and granulocyte production have been identified and are erythropoietin, thrombopoietin, and granulocyte colony‐stimulation factor (G‐CSF), respectively. Additional factors positively regulating erythropoiesis include insulin, insulin‐like growth factor, activin, and angiotensin II. Factors negatively regulating erythropoiesis include transforming growth factor beta‐ and alpha‐related ligand, growth and differentiation factor 11, inflammatory cytokines such as c‐interferon, tumor necrosis factor (TNF) alpha, and tumor necrosis factor‐related apoptosis‐inducing ligand.4

Figure 17‐1 Classical and revised roadmap of hematopoietic hierarchy. (a) In the classical hierarchy roadmap, LT‐HSCs and ST‐HSCs are both multipotent, but sit at different hierarchical levels owing to their distinct self‐renewal abilities. The HSCs give rise to the MPPs with an accompanying reduction of self‐renewal ability. Next there emerges a myeloid/lymphoid lineage segregation downstream of MPP. CLPs can produce lymphocytes. CMPs diverge into MEPs and GMPs. MEPs yield megakaryocytes and erythrocytes. GMPs generate granulocytes, macrophages, and dendritic cells. (B) In the revised hierarchy roadmap, LT‐HSCs, IT‐HSCs, and ST‐HSCs/MPP1 cells are all multipotent, but differ in their self‐renewal ability. In the branching trees, the HSCs differentiate into MPPs, which consist of MPP2, MPP3, and MPP4/LMPP subpopulations. MPP2 and MPP3 cells mainly give rise to CMPs, whereas MPP4/LMPP cells predominantly generate lymphoid lineages. Next, CMPs can produce mature hematopoietic cells via MEP and GMP stages. CLPs can yield mature lymphocytes, which also can derive from MPP4/LMPP cells directly. CLP, common lymphoid progenitor; CMP, common myeloid progenitor; GMP, granulocyte/macrophage progenitor; IT‐HSC, intermediate‐term hematopoietic stem cell; LMPP, lymphoid‐primed multipotent progenitor; LT‐HSC, long‐term hematopoietic stem call; MEP, megakaryocyte/erythrocyte progenitor; MPP, multipotent progenitor; ST‐HSC, short‐term hematopoietic stem cell.
Source: Zhang Y, Gao S, Xia J, Liu F. Hematopoietic Hierarchy – An Updated Roadmap. Trends Cell Biol. 2018;28(12):976–986. doi:10.1016/j.tcb.2018.06.001. © 2018, Elsevier.
The healthy human produces approximately 109 RBCs, 108 white blood cells, and 4 billion platelets every hour. Different cell types have different normal lifespans (e.g., 120 days for erythrocytes, 5–10 days for platelets, 6–8 hours for neutrophils, day to years for lymphocytes). Senescent or otherwise damaged erythrocytes are recognized and removed by the reticuloendothelial system. At least half of senescent RBCs are destroyed in the spleen by splenic macrophages and the remaining RBCs are destroyed in the liver, bone marrow, or other sites of the mononuclear phagocyte system. Aging platelets are also sequestered in the spleen and are subject to phagocytosis by macrophages. The death and removal of neutrophils and lymphocytes are less well understood.
RBCs are made up mostly of hemoglobin, a metalloprotein composed of heme (the iron‐containing portion that binds oxygen) and globin (amino acid chains that form a protein) groups. Normal hemoglobin types include adult hemoglobin A (HgbA; about 95%–98%) containing two alpha globulin chains and two beta globulin chains; HgbA2 (2%–3%) containing two alpha and two delta chains; and fetal hemoglobin (HgbF, up to 2%). HgbF has two alpha and two gamma chains. HgbF is the primary hemoglobin produced by the fetus during gestation. Its production usually falls to a low level shortly after birth.
Hemoglobinopathies occur when point mutations or deletions in the globin genes cause changes in the amino acids that make up the globin protein, resulting in abnormal forms of hemoglobin. The structure of hemoglobin may be abnormal in its behavior, production rate, and/or stability. Several hundred hemoglobin variants have been documented; however, only a few are common and clinically significant. The majority of these are beta chain variants that are inherited in an autosomal recessive fashion. Since a person inherits one copy of each beta‐globin gene from each parent, if one normal beta gene and one abnormal beta gene are inherited, the person is said to be a carrier or heterozygous for the abnormal hemoglobin. The abnormal gene can be passed on to offspring, but does not cause symptoms or disease in the carrier. If two abnormal beta genes of the same type are inherited, the person is considered to have the disease and is homozygous for the abnormal hemoglobin.
The initial laboratory test to assess hematopoietic health is a complete blood count (CBC) with differential (see Table 17‐1). When necessary, more specific testing to include a peripheral blood smear, bone marrow biopsy, molecular/cytogenetic analysis, and functional assessments may be ordered to further assess the patient.
Table 17‐1 Red blood cell indices.
Test Name | Normal Range (SI units) | Increased | Decreased |
---|---|---|---|
Red Blood Cell (RBC) | Adult male: 4.5–9.0 x 106/μAdult female: 4.5–5.1 x 106/μ | Polycythemia; erythrocytosis; fluid loss due to dehydration, diuretics, diarrhea, burns | Anemia |
RBC Indices | |||
Mean corpuscular volume | Adult: 80–93 μm2 | Vitamin B12 and folate deficiency | Iron deficiency anemia; thalassemia |
Mean corpuscular hemoglobin | 27.5–33.2 pg | Hyperchromia | Hypochromic anemia |
Mean corpuscular hemoglobin concentration | 33.4%–35.5% (concentration fraction 0.334–0.355) | Hyperchromia | Hypochromic anemia |
Hemoglobin | Adult male: 13–14.2 g/dL Adult female: 11.6–12.3 g/dL |
Same as RBC results | Same as RBC results |
Hematocrit | Adult male: 41.5%–50.4% Adult female: 35.9%–44.6% |
Same as RBC results | Same as RBC results |
RED BLOOD CELL DISORDERS
Erythrocytosis
Erythrocytosis is suspected when elevated hemoglobin (Hb) levels (>185 mg/dL for males, >165 mg/dL for females) or elevated packed RBCs (hematocrit [HCT]; >52% for males, >48% for females) is seen. As Hb and HCT are surrogate indicators of true red cell mass, absolute or true erythrocytosis is confirmed when the red cell mass exceeds 125% of the predicted value for body mass using specialized nuclear medicine tests (e.g., radioisotope RBC studies).5 Once an absolute erythrocytosis has been confirmed, it is desirable to identify the underlying etiology, which may be classified as either primary or secondary. Apparent erythrocytosis occurs in the presence of elevated venous HCT but RBC mass below 125% of predicted value. Relative erythrocytosis is when the RBC mass is in the normal reference range, but the plasma volume is decreased. This generally occurs with significant dehydration (e.g., from diuretics, persistent diarrhea, excessive use of alcohol, or burns).
Primary erythrocytosis is a condition in which the erythropoietic compartment of the bone marrow leads to increased red cell production. The predominant form of primary erythrocytosis is polycythemia vera (PV), discussed in detail below. Other causes of erythrocytosis include primary familial and congenital polycythemia, a rare primary form of erythrocytosis caused by mutations of the erythropoietin (EPO) receptor gene resulting in increased RBC mass and low EPO levels, tumors such as cerebellar hemangiomas or parathyroid adenomas causing increased EPO secretions, and rare genetic mutations to oxygen‐sensing pathways resulting in dysregulation of EPO synthesis.6
Secondary erythrocytosis is characterized by increased red cell production in response to something external to the bone marrow that causes tissue hypoxia, inappropriately increased erythropoietin production, or increased sensitivity to erythropoietin. Common causes of hypoxia include chronic lung disease, right to left cardiopulmonary vascular shunts, CO2 poisoning, high‐altitude habitat, smoking, and renal artery stenosis. Less common causes include EPO receptor mutations, Chuvash polycythemia (in which a mutation in the VHL gene affects the hypoxia‐sensing pathway), right to left arteriovenous shunts in the lungs, and proline hydroxylase 2 and hypoxia‐inducible factor 2 alpha (HIF‐2α) mutations. Secondary erythrocytosis may be congenital (high oxygen‐affinity Hb, EPO receptor‐mediated dysfunction, 2,3‐bisphosphogycerate mutase deficiency), or acquired. Acquired erythrocytosis may be due to central EPO‐mediated hypoxia driven, local EPO‐mediated hypoxia driven (end‐stage renal disease [ESRD], renal cysts), or pathologic EPO production (tumors or drugs).7 The term idiopathic erythrocytosis (IE) is reserved for cases in which all primary and secondary causes of increased red cell mass have been ruled out. As a consequence of increased recognition of primary and secondary causes of erythrocytosis, patients classified as having IE are on the decline.6
PV is a myeloproliferative neoplasm, a panmyelopathy with an estimated incidence of 1.9–2.3 cases per 100,000 persons/year and a slight male predominance. It is often caused by more than one genetic event in a single hematopoietic progenitor that results in clonal myeloid of pluripotent stem cell (expansion of red cell production). PV is characterized by an acquired Janus kinase 2 (JAK2) V617F mutation. JAK/STAT signaling plays a role in cellular proliferation and cell survival. The JAK2 V617F mutation accounts for 95%–97% of PV cases, while a similar mutation of JAK2 in exon 12 accounts for 3% of PV cases. The JAK2 V617F mutation is associated with dysregulation of tyrosine kinase receptor binding, resulting in increased RBC, platelet, and granulocyte production; mutation of JAK2 exon 12 results in increased RBCs only.8 PV shares several features with two other forms of myeloproliferative neoplasia: essential thrombocytosis (ET) and primary myelofibrosis (PMF). Collectively, these three conditions exhibit relatively normal cellular maturation, phenotypic and genotypic mimicry, JAK2 gene mutations, and a tendency to evolve into each other or develop myelofibrosis. There is growing evidence from studies in patients with sporadic PV as well as familial myeloproliferative neoplasms that the JAK2 V617F mutation is not the PV‐originating somatic mutation, but rather represents a late secondary change occurring in a clone initiated by as yet unidentified genetic change(s).9
The diagnostic criteria for PV were updated by the World Health Organization in 2016 and consist of both major and minor criteria. Major criteria are (1) Hb >16.5 g/dL in men, >16.0 g/dL in women, or HCT >49% in men, >48% in women, or increased red cell mass >25% above mean normal predicted value; (2) bone marrow biopsy showing hypercellularity for age with trilineage growth (panmyelosis), including prominent erythroid, granulocytic, and megakaryocytic proliferation with pleomorphic, mature megakaryocytes (differences in size); (3) presence of JAK2 V617F or JAK2 exon 12 mutation. Minor criterion: subnormal serum erythropoietin level. The presence of all three major criteria or the first two major plus the minor criterion is required for the diagnosis (see Table 17‐2).10
Clinical and Oral Manifestations
PV is usually asymptomatic and often only discovered incidentally. Its clinical course is measured in decades. It can be acquired at any age, but is rarely seen in children and increases exponentially in those aged >60 years.11 PV should be suspected in patients with elevated hemoglobin or HCT levels, splenomegaly, or portal venous thrombosis. When symptoms occur, they may include pruritis, vertigo, gastrointestinal (GI) pain, headache, paresthesias, burning pain in the feet and hands, fatigue, weakness, visual disturbances, tinnitus, and facial plethora. Pruritis following a bath or shower is often the predominant complaint and has been suggested to be due to mast cell degranulation.12
Major complications of PV (e.g., stroke, venous thromboembolism) are attributable to blood hyperviscosity and the qualitative and quantitative platelet alterations observed in the disease. Other complications include ocular migraine, transient ischemic attacks, thrombosis, leukocytosis, hyperuricemia, and splenomegaly. PV is an indolent disease with a lifespan that can exceed 40 years.11
PV can manifest intraorally with erythema (red‐purple color) of mucosa, glossitis, and erythematous, edematous gingiva.13 Spontaneous gingival bleeding can occur because the principal sites for hemorrhage, although rare, are reported to be the skin, mucous membranes, and gastrointestinal tract.
Treatment
Contemporary PV therapy is focused on reducing vascular risks and tailored to the thrombotic risk stratification of the patient. Low‐risk PV patients or intermediate‐risk PV patients with a high HCT level are treated with phlebotomies to reduce the HCT (<0.45% for males and <42% for females) plus low‐dose aspirin, if no contraindications are present. Poorly compliant patients or those who manifest progressive myeloproliferation warrant myelosuppressive therapy. Hydroxyurea is the primary drug of choice, with anagrelide or peginterferon‐alpha as alternatives. All of these agents have potential side effects. Hydroxyurea is a ribonucleotide reductase inhibitor and an effective agent in managing PV and is the drug of choice. It is important to remember that there is risk for leukemic transformation, so it is usually not recommended in young patients. Current nonmyelotoxic therapy is ruxolitinib, which targets JAK2 kinase.11 Busulfan has been shown to induce durable hematologic response in patients unable to tolerate hydroxyurea.14 Radioactive phosphorus (32P) has been used in the past, with a success rate of 80%–90%; however, its association with an increased incidence of acute leukemic transformation severely restricts its usefulness to patients >75 years of age.
Table 17‐2 Polycythemia vera diagnostic criteria.
Major Criteria | Minor Criterion |
---|---|
Hb >16.5 g/dL in men >16.0 g/dL in women |
Subnormal serum erythropoietin level |
Bone marrow biopsy: panmyelosis, prominent erythroid, granulocytic, and megakaryocytic proliferation with pleomorphic, mature megakaryocytes | |
JAK2 V617F or JAK2 exon 12 mutation |
Oral Health Considerations
There are no established guidelines addressing the delivery of dental care for the PV patient. The delivery of routine dental care for the well‐controlled PV patient likely incurs minimal risk. Low‐dose aspirin is rarely associated with hemorrhagic complications from dental extractions. Poorly controlled patients are at an increased risk for both thrombotic and hemorrhagic complications due to blood hyperviscosity and concurrent qualitative and quantitative platelet alterations.15,16 It is important to know that inappropriately high HCT can lead to spuriously high prothrombin time values.11
Anemia
Anemia is defined as a reduction in the quantity of the oxygen‐carrying pigment (Hb) in the blood. The normal range for hemoglobin is 13–14.2 g/dL for men and 11.6–12.3 g/dL for women.17 Data from large population studies suggest that hemoglobin levels for African Americans tend to be 0.8–0.7 g/dL lower, perhaps owing to the high frequency of alpha‐thalassemia in this population. Another important factor is the trend of hemoglobin. For example, a patient with previous hemoglobin values at the higher end of the normal range, who presents with a hemoglobin concentration at the lower end of the normal range, can now be considered anemic.17
The signs and symptoms of anemia occur as a consequence of the hypoxia and compensatory physiologic responses. Typical symptoms include fatigue and dizziness. The classic sign of anemia is pallor, which may be observed in the conjunctivae, face, nail beds, tongue, and palmar creases. Analysis of National Health and Nutrition Examination Survey (NHANES) data from 2003 to 2012 shows that 5.6% (95% confidence interval [CI]: 5.1–6.1%) of the US population had anemia.18 In the elderly, anemia is associated with decreased physical performance of daily activities, cognitive impairment, depression, diminished quality of life, greater hospital admissions, and impaired survival.19
The initial laboratory tests used to assess suspected anemia are the CBC and the blood smear. The blood smear is used to morphologically characterize the red cells (e.g., macrocytic, normocytic, microcytic, normocytic, hypocytic). Once discovered, it is essential to determine the underlying cause of the anemia. There are several broad causes of anemias, which can be due to impaired production of the RBC (EPO deficiencies, hypoproliferation, impaired maturation of the RBC, impaired heme or globin production), accelerated destruction, consumption or loss of RBCs due to morphologic changes (sickle cell, cell membrane defects, enzymopathies), reduced protein stability, and factors extrinsic to the RBC.
Anemias Due to Impaired Production
Erythropoietin Deficiency
EPO is a hormone secreted by the liver in fetal development and then primarily by peritubular fibroblasts in the renal cortex of the kidneys in response to hypoxia or low O2 tension (hemorrhage, hemolysis, etc.). It circulates in the plasma and binds to receptors on erythroid progenitor cells in the bone marrow to promote proliferation and differentiation of erythroid precursors to increase RBC production in a dose‐dependent manner. EPO mRNA is also detectable in liver, spleen, bone marrow, lung, and the brain, where it may play a paracrine role. Renal function impairment (i.e., chronic kidney disease) leads to insufficient EPO production in the kidney, either from direct damage to the EPO‐producing cells of the kidney or from suppression of EPO production by inflammatory cytokines in a patient with inflammatory disorders such as rheumatoid arthritis, cancer, or acquired immunodeficiency syndrome (AIDS).20
Impaired Hemoglobin Production
Hb, the protein in the RBC responsible for transporting oxygen, is made up of four subgroups, each containing a heme group with an iron atom. If there is no iron within the heme group (due to insufficient iron or inefficient delivery of iron), a defect in the synthesis of the heme group and resulting ability to bind, or lack of globin production (thalassemia), the RBC is smaller than normal. The term for this is microcytic anemia. To understand microcytic anemias, it is crucial to understand iron metabolism and homeostasis. The lifespan of a normal RBC is ~120 days, when senescent erythrocytes are removed from circulation by macrophages of the spleen. Most of the iron contained in the Hb is stored in macrophages or transported to the blood plasma bound to transferrin back to the myeloid tissue and used in the production of Hb for the new RBC. Hepcidin is a regulatory hormone produced by hepatocytes and functions to decrease plasma stores of iron. It does this by acting on enterocytes of the small intestine to inhibit the iron‐exporting activity of ferroportin, thereby promoting cellular storage of iron and lowering plasma iron concentration. Plasma iron levels are maintained at a range of 10–30 μM and whole‐body stores of 0.3–1 g. The following section describes microcytic anemias.
Iron Deficiency Anemia
Iron deficiency anemia (IDA) is the most common type of anemia worldwide. It is defined as a reduction in total body iron to an extent that iron stores are fully exhausted, and some degree of tissue iron deficiency is present in the presence of anemia. Iron deficiency affects approximately 40% of preschool children, 30% of menstruating females, and 38% of pregnant women. The most common cause of iron deficiency in children is malnutrition; in adult males, postmenopausal women, and the elderly, the cause is bleeding (cancers, GI bleed); and in women of childbearing age, menstruation, pregnancy, and, minimally, lactation. First, menstrual iron loss exceeds dietary intake. Second, dietary intake is insufficient to meet the demands of fetal development in the gravid or lactating female. Pathologic causes of IDA are more commonly observed in older adults and include gastritis, peptic ulcer disease, ulcerative colitis, GI carcinoma, achlorhydria, and celiac disease.
Iron resistance are disorders of the GI tract such as partial or total gastrectomy, Helicobacter pylori infection, celiac disease, and gluten sensitivity, another common cause of IDA. IDA affects up to 45% of people, particularly women, who have undergone gastric bypass, as the procedure removes active iron absorption from the gut and caloric intake is severely restricted.21
Plummer–Vinson syndrome, also called Paterson–Kelly syndrome, is rare and characterized by the classic triad of dysphagia, IDA, and upper esophageal webs or strictures.22,23 It usually affects middle‐aged white women in the fourth to seventh decades of life, but has also been described in children and adolescents. The dysphagia may be intermittent or progressive over the years, is usually painless and limited to solids, and may be associated with weight loss. Symptoms resulting from anemia (weakness, pallor, fatigue, tachycardia) dominate the clinical picture. Other potential findings include glossitis, glossopyrosis, glossodynia, angular cheilitis, koilonychia, fragility, thinning of nails, and brittle hair. Radiologic examination of the pharynx shows the presence of webs.24 The etiopathogenesis is unknown, but it is postulated that iron deficiency adversely affects iron‐dependent enzymes in the epithelium of the upper GI tract, increasing free radical stress, DNA damage, and malignant transformation.22
Iron deficiency anemias can often be treated effectively with iron supplementation. In cases of significant obstruction of the esophageal lumen by esophageal webs/strictures with persistent dysphagia in Plummer–Vinson anemia, rupture and mechanical dilation of the web may be required. Since Plummer–Vinson syndrome is associated with an increased risk of squamous cell carcinoma of the pharynx and the esophagus, these patients are monitored closely.22
Anemia of Inflammation/Chronic Disease
Anemia of infla mmation (AI), also known as anemia of chronic disease (ACD), is the second most prevalent anemia and the most common type of anemia seen in hospitalized or chronically ill patients. It affects up to 77% of the elderly. The most common associated diseases include infections, cancer, autoimmune diseases, chronic kidney disease, and rejection after solid organ transplant.25
The body needs 20 mg of iron per day. Only 1–2 mg is absorbed from the intestine; the rest is obtained from recycling senescent RBCs or iron stores in hepatocytes and macrophages, as it is not excreted from the body. One of the main processes that are associated with anemias of inflammation are those that affect iron metabolism via hepcidin. Hepcidin is the main iron‐regulatory hormone responsible for controlling the actions of ferroportin, a major iron export protein located on cell surfaces (enterocytes, macrophages, and hepatocytes) that release iron into the plasma. Hepcidin directly binds to ferroportin to decrease its functional activity. During iron overload, hepcidin synthesis increases and functions to shut down iron absorption by enterocytes, decrease recycling by macrophages, and increase storage into the hepatocytes.
Inflammation (acute, chronic, autoimmune conditions, and malignancy) acts as a potent stimulus for hepcidin production through JAK‐STAT signaling through proinflammatory cytokines (interleukin (IL)‐6, IL‐1, TNF‐alpha). Increased hepcidin results in increased stored iron into marrow, splenic, and hepatic macrophages and lowered serum iron levels, but not a true iron deficiency and otherwise known as a disorder of iron distribution. Increased cytokines produced in systemic inflammation also push hematopoiesis more toward myeloid production instead of erythroid production and activate erythrophagocytosis by macrophages, shortening the lifespan of erythrocytes by approximately 25%.26 IL‐6 is one of the most important cytokines involved in AI/ACD. It does this by inhibiting TNF‐alpha and inducing transcription of ferritin, which increases retention of iron and storage within reticuloendothelial cells, and inhibits erythropoiesis and hemoglobin synthesis.25–28
Sideroblastic Anemia
Sideroblastic anemias are inherited or acquired bone marrow disorders. Heme is assembled from iron and protoporphyrin IX in mitochondria by ferrochelatase. Iron within the mitochondria is also required to generate iron–sulfur clusters that regulate cellular iron uptake, heme synthesis, and iron storage. In sideroblastic anemia, there is pathologic iron accumulation in the mitochondria of erythroid precursors, which appear as coarse granules occurring in a perinuclear distribution that stains with Prussian blue. Iron metabolism is altered, leading to ineffective erythropoiesis to generate a systemic iron overload state that suppresses hepcidin to increase intestinal absorption of iron. Treatment consists of repeated transfusions for severe anemia, which adds to iron burden and then iron depletion preformed via phlebotomy or iron chelation. Pharmacotherapeutics include deferoxamine (Desferal), deferasirox (Exjade), and deferiprone (Ferriprox).29,30
Diagnosis
It is often difficult to serologically differentiate between IDA and ACD. IDA has three stages: the first stage exhibits iron depletion with decreased iron stores without decrease in serum iron levels or Hb concentration and low serum ferritin; the second phase exhibits abnormal iron serologies (reduced transferrin saturation, increased total iron‐binding capacity, and increased zinc protoporphyrin); and in the third phase there is Hb concentration of less than the lower limit of the normal range.17
ACD presents as a normocytic/normochromic anemia that changes with time to become hypochromic and microcytic. Less than 25% of cases will present as microcytic hypochromic anemia. There is decreased serum iron, total iron‐binding capacity, and transferrin saturation and an increase in serum ferritin and bone marrow iron stores. Table 17‐3 shows the differences between ACD and IDA.26 To complicate matters, the two conditions can coexist.
Table 17‐3 Differences in laboratory measures in iron deficiency anemia and anemia of inflammation.
Source: Ganz T. Anemia of inflammation. Longo DL, ed. N Engl J Med. 2019;381(12):1148–1157. doi:10.1056/NEJMra1804281. © 2019, Massachusetts Medical Society.
Laboratory Measure | Iron Deficiency Anemia | Anemia of Inflammation |
---|---|---|
MCV | Low | Normal |
MCH | Low | Normal |
Reticulocyte hemoglobin | Low | Normal |
% Hypochromic erythrocytes | High | Low |
Serum transferrin | High | Low |
Serum transferrin receptor | High | Normal |
Serum ferritin | Low | High |
Serum hepcidin | Low | High |
MCH, mean corpuscular hemoglobin; MCV, mean corpuscular volume.
Diagnosis of ACD is made in the presence of systemic inflammation, iron restriction not caused by systemic iron deficiency (low serum iron, low transferrin saturation, and low ferritin), and symptoms such as fatigue, exercise intolerance, and dyspnea. Indeed, a patient with an inflammatory condition such as inflammatory bowel disease (IBD) may manifest both IDA and ACD. However, patients with ACD have normal transferrin receptor levels and high hepcidin levels, while patients with IDA have high transferrin receptor levels and normal or low hepcidin levels. Iron stores are usually adequate, but there is impaired iron delivery and EPO production is usually suppressed. When the diagnosis remains ambiguous, further testing including a bone marrow biopsy may be necessary.17
Sideroblastic anemias can be congenital or acquired. The distinction between the two may not be apparent until adulthood. The clinical laboratory findings include microcytosis, hypochromia, increased red cell distribution width, and absence of evidence for common causes of microcytic anemia such as iron deficiency and thalassemia. Definitive diagnosis is based on showing a genetic defect by mutational analysis.
Clinical and Oral Manifestations
The most important clinical symptom of anemia is chronic fatigue. Outward signs may be subtle and may include pallor of the conjunctivae, lips, and oral mucosa; brittle nails with spooning, cracking, and splitting of nail beds; and palmar creases. This has traditionally been used by physicians in the diagnosis of anemia. Among 50 prospectively examined patients, a statistically significant correlation was noted between hemoglobin concentration and the following: color tint of the lower eyelid conjunctiva, nail‐bed rubor, nail‐bed blanching, and palmar crease rubor.31 Other findings may include palpitations, shortness of breath, numbness and tingling in fingers and toes, and bone pain.
Glossitis and stomatitis are recognized oral manifestations of anemia. In a study of 12 patients, oral signs and symptoms of anemia included angular cheilitis (58%), glossitis with different degrees of atrophy of fungiform and filiform papillae (42%), pale oral mucosa (33%), oral candidiasis (25%), recurrent aphthous stomatitis (8%), erythematous mucositis (8%), and burning mouth (8%) for several months to 1 year’s duration.32 IDA or ACI should be suspected in every case of glossitis, glossodynia, angular cheilitis, erythematous mucositis, oral candidiasis, recurrent oral ulcers, and burning mouth when no other obvious causes are identified.33–35 These findings are believed to be caused by impaired cellular immunity, deficient bactericidal activity of polymorphonuclear leukocytes, inadequate antibody response, and epithelial abnormalities attributed to lack of iron.36 Clinically evident atrophic changes of the tongue, giving a smooth red tongue appearance, in patients with IDA have been associated with a significant reduction in the mean epithelial thickness of the buccal mucosa as determined histologically.37
Treatment
Treatment is usually focused on treating the underlying disease (infection, autoimmune disorder, cancer), but most AI disorders and malignancies are chronic conditions. Otherwise, the goal is to improve the oxygen‐carrying capacity of blood. This is achieved through iron supplementation, blood transfusions, erythropoiesis‐stimulating agents, and downregulating the production of TNF‐alpha and IL‐6. Novel agents include hepcidin antagonists (monoclonal antibodies, siRNA, antisense oligonucleotides, hepcidin‐binding proteins, and aptamers) are in development. Vitamin D deficiency has also been associated with increased prevalence of ACD, so vitamin D supplementation is also a consideration.27,28
For IDA, oral iron supplementation is safe, cost‐effective, and convenient. The goal is to increase serum hemoglobin by 1–2 g/dL every 2 weeks, and ultimately restore iron stores in in about 3–4 months. Ferrous sulfate and ferrous gluconate have good bioavailability and contain 20% and 12% of elemental iron for absorption, respectively.38 The recommended dose for both is 325 mg three times per day. Foods and medications that inhibit iron absorption include tea, coffee, phosphate‐containing carbonated beverages, antacids, proton pump inhibitors, and H2‐blockers. Adverse effects of oral iron therapy are dose related, can adversely affect compliance, and include nausea, epigastric discomfort, and constipation. For such cases a lower dosage regimen should be attempted.
When oral iron supplementation is ineffective due to poor patient compliance or intolerance, intravenous iron therapy is indicated. Other possible indications for intravenous iron therapy are high iron requirements due to chronic uncorrectable bleeding or chronic hemodialysis; iron malabsorption secondary to a GI condition; IBD with ineffective erythropoiesis, poor iron absorption, and intolerance to oral iron supplementation; and the need for rapid restitution of iron stores (e.g., preoperative).39 Intravenous iron products are made up of nanoparticles of iron oxyhydroxide gel in colloidal suspension, held within a stabilizing carbohydrate shell.38 Available products include high molecular weight iron dextran, low molecular weight iron dextran, iron sucrose, ferric gluconate, and ferumoxytol. Serious and potentially life‐threatening hypersensitivity reactions may occur, with the highest risk associated with high molecular weight preparations.
Thalassemia
Thalassemias are a group of inherited disorders of hemoglobin synthesis leading to reduced‐production of globin chains. Gene clusters on chromosomes 16 and 11 code for alpha‐ and beta‐like globins, respectively. Hemoglobin A, the major hemoglobin component of adult RBCs, is made up of two alpha and two beta chains (Hb A α2 β2). Defects can occur in either the alpha or beta‐hemoglobin chain and these defects yield difficulties in transportation of oxygen. There are over 100 genetic forms of alpha‐thalassemias, with phenotypes ranging from asymptomatic to lethal. Clinically relevant forms of alpha‐thalassemia usually involve α0‐thalassemia, either coinherited with α+‐thalassemia and resulting in hemoglobin H (HbH) disease, or inherited from both parents and resulting in hemoglobin Bart’s hydrops fetalis, which is lethal in utero or soon after birth.40 Deletion of or mutations in three alpha‐chain genes lead to HbH disease, which presents with more prominent anemia.41 Worldwide, an estimated 5% of the population carry an alpha‐thalassemia variant trait.40 In the United States, the incidence of alpha‐thalassemia is 1 in 1,000,000 and the incidence of beta‐thalassemia is 1 in 100,000.42 Historically, alpha‐thalassemia is thought to be highly protective against severe malaria, therefore more prevalent in persons of African and Southeast Asian descent, and beta‐thalassemia is most common in persons of Mediterranean, African, and Southeast Asian descent. However, human migration greatly blurred these distinctions.43
In beta‐thalassemia, insufficient beta‐globin synthesis results in excess alpha‐hemoglobin production. There have been over 200 mutations in the beta‐globin gene that cause disease. Beta‐thalassemia has been categorized as minor, major, and intermedia based on alpha‐globin or beta‐globin chain imbalance, clinical signs, and the severity of anemia. Beta‐thalassemia minor trait or carrier manifests as microcytic anemia and is clinically asymptomatic. Beta‐thalassemia major presents with severe anemia in infants and is transfusion dependent. Both beta‐thalassemia major and beta‐thalassemia intermedia result from homozygous or compound heterozygous inheritance of a mutated beta‐globin gene. Beta‐thalassemia intermedia can also result from increased production of alpha‐globin gains.44
Clinical and Oral Manifestations
Clinical signs and symptoms of thalassemia are dependent on the severity of the disease and range from none to life‐threatening. With regard to alpha‐thalassemia, Bart’s hydrops fetalis is lethal in utero or shortly after birth due to severe hypoxia. If transfusions are administered intrauterine and after delivery, life expectancy can be extended to 5 years. HbH presents with variable phenotypes with varying severities, ranging from moderate anemia and splenomegaly to a more severe course with episodes of intercurrent infection, severe anemia, and need for transfusions. Other manifestations include jaundice, growth retardation in children, infections, leg ulcers, gall stones, folic acid deficiency, and drug‐induced hemolysis.
Patients with beta‐thalassemia trait are usually asymptomatic, but may have mild anemia. Patients with beta‐thalassemia major develop signs and symptoms as infants, including failure to thrive, pallor, weakness, jaundice, protruding abdomen with enlarged spleen and liver, dark urine, abnormal facial bones, and growth retardation. Compensatory hypertrophy of erythroid marrow with extramedullary erythropoiesis may lead to deformities of the long bones and typical craniofacial changes (see below). Beta‐thalassemia intermedia patients manifest variable signs and symptoms of anemia that are milder and occur later in life; however, they are prone to experiencing thrombotic events, especially if splenectomized. Such events include deep vein thrombosis, stroke, portal vein thrombosis, and pulmonary embolism.
Significant oral manifestations related to thalassemia appear to occur more frequently in the beta‐thalassemia group and are reflective of the underlying extramedullary erythropoiesis observed in the more severe phenotypes.45 Characteristic craniofacial deformities include a class II skeletal base relationship with a short mandible, reduced posterior facial height, increased anterior facial proportions, and “chipmunk facies.”46 Other reported potential findings include spike‐shaped and short roots, taurodontism, attenuated lamina dura, enlarged bone marrow spaces, small maxillary sinuses, absence of inferior alveolar canal, retained primary teeth, and thin cortex of the mandible.47 Dental arch morphologic changes include a narrower maxilla, high arched palate, and smaller incisor widths for the maxillary and mandibular arches.48,49 Consistent with general growth retardation, the dental development of 31 of 39 patients with Cooley’s anemia was delayed by a mean of 1.11 years and 0.81 years for boy and girls, respectively.49
Individuals with thalassemia appear to experience similar rates of gingivitis and periodontitis to healthy controls.50,51 An increased caries risk has been reported, which may be attributable to such factors as disease‐induced immunologic dysfunction, decreased access to care, and insufficient patient oral hygiene.50,52 While the parotid salivary flow rates in Cooley’s anemia are similar to controls, quantitative changes consisting of reduced levels of phosphorus, immunoglobulin (Ig) A, and urea have been reported.53,54 Increased oral cavity levels of Streptococcus mutans and Candida have also been reported.53,55
Diagnosis
Thalassemia is diagnosed using a battery of laboratory tests, including a CBC, qualitative and quantitative hemoglobin analysis, and DNA testing. Screening programs are considered for populations where thalassemias are most prevalent.40 People with thalassemia have microcytic anemia, lowered hemoglobin, and defects in alpha or beta chains of hemoglobin. The clinical signs and symptoms of severe thalassemia usually become apparent within the first 2 years of life.
Treatment
The prognosis for thalassemia patients has improved dramatically over the past decades, but medical management remains complex and multidisciplined. Appropriate genetic counseling and screening should be afforded all patients with thalassemia trait.40 Patients with alpha‐ or beta‐thalassemia trait generally require no special medical management.
Mild HbH patients may require intermittent transfusion therapy, mainly during intercurrent illness. More severely affected HbH patients may require chronic transfusions, iron chelation therapy, and eventual splenectomy. Management strategies for patients with beta‐thalassemia (Cooley’s anemia) usually entail periodic lifelong blood transfusions to maintain the hemoglobin level above 90–105 g/L, to prevent growth impairment, organ damage, and bone deformities. Transfusions are given sporadically to patients under acute stress as well. The need for transfusions to manage beta‐thalassemia patients is more episodic and dictated by the severity and potential progression of the disease. Splenectomies are traditionally performed as an alternative or adjunct to transfusion.44
Regularly transfused patients require iron chelation to resolve the inevitable iron accumulation that leads to dysfunction, primarily involving the heart, liver, and endocrine system. In the United States, there are three Food and Drug Administration (FDA)‐approved iron chelators: deferoxamine (Desferal), deferasirox (Exjade), deferiprone (L1) and Ferriprox‐L1 (Ferriprox). The most commonly used agent is the oral dispersible agent deferasirox, likely due to its good oral bioavailability and convenient once‐a‐day dosing. Common side effects include dose‐related GI symptoms (e.g., nausea, vomiting, diarrhea, abdominal pain) and mild skin rash. The most serious side effects with deferasirox are potential kidney damage and renal tubular acidosis.44
Hemopoietic stem cell transplantation is the only curative treatment for thalassemia. Disease‐free survival exceeds 80% with human leukocyte antigen (HLA)‐matched sibling donors. Cord blood transplantation may be successful, but there is a 5%–10% mortality from transplant conditioning, graft‐versus‐host disease, and graft failure. Clinical guidelines are available.44,56 Future therapeutic strategies involving normal gene transfer via suitable vectors, molecular targeting (i.e., BCL11A) using CRISPR/Cas9, ligand traps (sotatercept and luspatercept) for stimulation of late‐stage erythropoiesis, JAK2 inhibition (in patients who develop myeloproliferative disorders), and agents to stimulate hepcidin production are being investigated.44
Infections are major complications and constitute the second most common cause of mortality and a main cause of morbidity in patients with thalassemia. Predisposing factors for infections in thalassemic patients include severe anemia, iron overload, splenectomy, and a range of immune abnormalities.
Oral Health Considerations
There are no contraindications for providing routine dental care for thalassemia patients under proper medical management. Splenectomized patients are more susceptible to developing postsplenectomy sepsis from encapsulated bacteria (e.g., Streptococcus Pneumoniae, Haemophilus Influenzae, and Neisseria Meningitidis). Prevention of postsplenectomy sepsis includes immunization against the abovementioned bacteria, and early antibiotic treatment for fever and malaise. Additionally, patients with beta‐thalassemia intermedia may be on an antithrombotic agent, so one should caution about prolonged bleeding.57
Impaired Maturation (Macrocytosis)
The term macrocytosis refers to a blood condition in which RBCs are larger than normal and is reported in terms of mean corpuscular volume (MCV). MCV, the average volume of RBCs, is calculated as HCT × 1,000 divided by RBC (millions/μL). Normal MCV values range from 80 to 100 femtoliters (fL), depending on gender, age, and reference laboratory. Macrocytosis is identified by reviewing peripheral blood smears and/or by automated RBC indices, and is diagnosed when MCV is >100 fL. It is a relatively common finding with a reported incidence ranging from 2% to 4% and about 60% of those who have anemia. Potential causes include alcoholism and B12 or folate deficiency. Medications that interfere with DNA synthesis, such as methotrexate, trimethoprim‐sulfamethoxazole, nucleic acid analogs (5‐fluorouracil, zidovudine), and others (hydroxyurea, pentamidine, phenytoin, pyrimethamine, sulfasalazine, triamterene), may result in macrocytosis. Measures to address the underlying cause often result in normalization of the MCV. Other causes of macrocytosis are variants of associated conditions such as Down syndrome and pregnancy.58
B 12 and Folate Deficiency Anemia
Vitamin B12 and folate are cofactors necessary for the enzyme methionine synthase used in the conversion of homocysteine to methionine. As a byproduct of this reaction, methyl‐ tetrahydrofuran (THF) is converted to THF, which is converted to intermediates used in the synthesis of pyrimidine bases of DNA. In B12 deficiency, homocysteine cannot be converted to methionine, and thus methyl‐THF cannot be converted to THF and pyrimidine bases cannot be formed, slowing down DNA synthesis and affecting rapidly proliferating cell lines, resulting in a megaloblastic anemia, among other problems.59 From a hematologic perspective, lack of either B12 or folate results in an essentially identical megaloblastic anemia.
B12 deficiencies or pernicious anemia can be due to insufficient B12 absorption associated with an autoimmune‐related atrophy of the gastric mucosa, which reduces the number of parietal cells that produce intrinsic factor (IF). IF is a protein produced by the parietal cells of the fundic mucosa of the stomach that binds B12 to allow it to be absorbed through the GI tract. Decreases in IF may be due to gastric disease or surgery (complete or partial gastrectomy or gastric reduction), intake of drugs that affect gastric acid secretion resulting in increased stomach pH and inability for B12 to be released from the food matrix, and/or reduced B12 consumption (vegetarian/vegan diet, chronic alcoholism). More than 90% of patients with pernicious anemia have serum antibodies against gastric parietal cells and about 50% have antibodies against intrinsic factor.60 The predominant causes of folate deficiency involve scenarios of inadequate intake, such as malnutrition and alcoholism. The increased physiologic folate and B12 requirement associated with pregnancy or lactation and conditions of chronic hemolysis or hemorrhage may lead to an anemic state. Secretion of intrinsic factor decreases as the stomach acid pH becomes more alkaline, therefore long‐term use of proton pump inhibitors may result in pernicious anemia. Vitamin B12 deficiency prevalence varies by age range (3% ages 20–39, 4% ages 40–59, 6% ≥60 years), with marginal depletion occurring frequently (>20%) among those 60 years or older.61
Clinical and Oral Manifestations
Fatigue, decreased mental concentration, glossitis, and weakness are characteristic of folate or B12 deficiency. B12 deficiency may present as neurologic symptoms such as clumsiness, unsteady gait, and paresthesia. Prolonged severe B12 deficiency, as may be seen in pernicious anemia, may lead to demyelination of the dorsal columns of the spinal cord, resulting in more advanced signs and symptoms such as peripheral neuropathy and ataxia. Severe deficiency shows evidence of bone marrow suppression.62 Unfortunately, these advanced signs and symptoms are often not reversed with replacement therapy.
Oral signs of folate and B12 deficiency are similar to those observed with IDA or ACI and include a beefy red tongue with smooth or patchy areas of erythema. Symptoms include soreness or a burning sensation affecting the tongue, lips, buccal mucosa, and other mucosal sites. Paresthesia and taste alterations have been reported.63
Diagnosis
The diagnostic process begins by establishing the presence of vitamin B12 (cobalamin) or folate deficiency in the presence of clinical signs. The primary laboratory investigations include total B12, holotranscobalamin, metabolites, methylmalonic acid (MMA), and homocysteine.64 Serum cobalamin and folate levels are assessed in tandem. To determine differences between pernicious anemia and other causes of low cobalamin, anti‐IF antibody assay is the preferred test. Newer assays measure holotranscobalamin II (the proportion of transcobalamin II conjugated to vitamin B12). If tested after oral administration of cobalamin, it can be used to assess intestinal uptake and diagnose vitamin B12 malabsorption.62
Use of the Schilling test (which measures cyanocobalamin absorption by increasing urine radioactivity after an oral dose of radioactive cyanocobalamin) for detection of pernicious anemia has been supplanted for the most part by serologic testing for parietal cell and intrinsic factor antibodies.65
Treatment
Treatment strategies for folate or B12 deficiency are dictated by the underlying cause and B12 repletion. Protocols entail parenteral B12 administration of 1000 μg cyano‐B12 or hydroxy‐B12 intramuscular injections daily or every other day, followed by weekly injections for up to 8 weeks, then every 3–4 weeks. Oral administration (high‐dose cyano‐B12 2000 μg oral tablets daily until remission, then 1000–2000 μg daily) is an effective alternative to injections to manage deficiency. Even when intrinsic factor is not present to aid in the absorption of vitamin B12, as in pernicious anemia or in other diseases that affect the usual absorption sites in the terminal ileum, oral therapy is still effective. However, prescribed folate supplementation may still be necessary in scenarios of alcoholism and malnutrition.
Accelerated Destruction, Consumption, or Loss
The normal RBC lifespan is 110–120 days in the circulation. Hemolytic diseases result in anemia, if the bone marrow is not able to replenish adequately, the prematurely destroyed RBCs. The hemolytic anemias are classified as either inherited or acquired. Inherited forms include sickle cell disease (SCD), thalassemias (described above), hereditary spherocytosis, hereditary elliptocytosis, glucose‐6‐phasphate dehydrogenase (G6PD) deficiency, and pyruvate kinase deficiency. Acquired forms of hemolytic anemias are immune hemolytic anemia, mechanical hemolytic anemias, paroxysmal nocturnal hemoglobinuria (PNH), and exposure to certain infections, toxins, or snake venom.
With acute hemolytic disease, the signs and symptoms depend on the mechanism that leads to red cell destruction. The release of free hemoglobin occurring in intravascular hemolysis may present as acute flank pain, free hemoglobin in the plasma and urine, and renal failure. In patients with chronic or progressive anemias, symptoms depend on the patient’s age and adequacy of blood supply to critical organs. With moderate anemia, symptoms may include fatigue, loss of stamina, breathlessness, tachycardia, and, less commonly, jaundice and hemoglobinuria. Physical findings include jaundice of skin and mucosae, splenomegaly, and other findings associated with specific hemolytic anemias.
A careful history and physical examination provide important clues to the diagnosis of hemolytic anemias. Once a patient presents with clinical signs and symptoms of anemia, laboratory testing should be supported by a complete drug and toxin exposure history and family history. Laboratory tests in the anemic patient may be used initially to demonstrate the presence of hemolysis and define its cause. An elevated reticulocyte count is the most useful indicator of hemolysis, reflecting erythroid hyperplasia of the bone marrow. Assessment of RBC morphology, findings on the peripheral blood smear, and, rarely, bone marrow biopsy may provide additional clues to support the specific diagnosis.
Sickle Cell Disease
SCD, formerly known as sickle cell anemia (SCA), is an umbrella term that defines a group of inherited diseases (including SCA, HbSC‐ and HbS‐beta‐thalassemia) characterized by mutations in the gene encoding hemoglobin subunit beta, resulting in a glutamic acid‐to‐valine substitution in the sixth position on the beta‐hemoglobin chain. The resulting mutant protein generated from the sickle beta‐globin subunit is sickle Hb (HbS). When the Hb is not bound to oxygen, HbS can polymerize and cause the erythrocytes to assume a crescent or sickled shape.66 The sickled erythrocytes form distorted rigid sickle cells, which lose their ability to effectively transport oxygen to the microcirculation and are prone to hemolysis. The sickled cells also cause recurrent vaso‐occlusive episodes, leading to end‐organ ischemia and necrosis affecting nearly every organ in the body.
SCD is an inherited autosomal codominant trait. It affects an estimated 100,000 people in the United States and the global annual incidence of SCD exceeds 300,000.67 About 100,000 individuals in the United States have the disease and most are African American. Globally, there are over 300 million carriers of SCD. The life expectancy of adults with SCD in high‐income and middle‐income countries yields a median survival of 67 years. In lower‐income regions, life expectancy is reduced by about 30 years and patients have a poor quality of life. Treatments remain costly, limiting access to care to those of higher socioeconomic standing.
While vaso‐occlusive events clinically characterize SCD, the etiopathogenesis of SCD is far more complex and involves ongoing hemolysis; increased RBC dehydration and adherence to endothelium; vascular instability due to nitric oxide deficiency; inflammation, hypercoagulability, increased neutrophil adhesiveness, and platelet activation. Patients with SCD may have other hemoglobin genotypes including compound heterozygosity for the HbS gene and other Hb variants such as HbC, HbE, and HbD or variants of HbS‐beta‐thalassemia.67 Fetal hemoglobin (HbF) is excluded from the HbS polymer. It usually peaks by mid‐gestation and drops to about 1% by the age of 6 months. In SCD, it is maintained in adults at higher levels.
Endothelial activation, induced directly or indirectly by the proinflammatory behavior of sickle erythrocytes, is the most likely initiating step toward vaso‐occlusion. Stressors that can lead to vaso‐occlusion typically include viral and bacterial infection, hypoxia, dehydration, iron overload, and cell and fluid phase–related causes. Microvascular occlusion arises predominantly in localized areas of marrow, leading to necrosis. Inflammatory mediators activate nociceptive afferent nerve fibers, evoking the pain response. Commonly affected areas are the long bones, ribs, sternum, spine, and pelvis, often with multiple‐site involvement.
Clinical and Oral Manifestations
Nearly every organ is affected in SCD.67 Damaged erythrocytes cause vaso‐occlusion, which lead to acute complications including vascular–endothelial dysfunction, functional nitric oxide deficiency, inflammation, oxidative stress and reperfusion injury, hypercoagulability, increased neutrophil adhesiveness, and platelet activation, ultimately leading to ischemic damage to tissues.68 This damage results in severe pain and/or organ failure.
Pain is characteristic of SCD and the most common reason for hospitalization. Pain management is personalized and guided by pain severity. Home management with analgesics and hydration is first‐line therapy. If that is insufficient, rapid administration of opioids is recommended. The most frequently affect sites are the lower back, knee/shin area, and hips. One study describes four phases of the pain event: prodromal, consisting of mild localized pain without hematologic aberrations; initial/infarctive, consisting of acute pain lasting 2–3 days; a postinfarctive phase; and a recovery phase. Laboratory findings include elevated reticulocytes, lactate dehydrogenase, and C‐reactive protein (CRP). The resolving phase occurs when the pain intensity is decreased, usually as a result of appropriate care.69
Acute chest syndrome is the second most frequent cause of hospitalization and the leading cause of death in patients with SCD. It is associated with >10% fatality or association with neurologic events. It can be defined as a new infiltrate in at least one segment of the lung, along with fever and respiratory symptoms. Splenic dysfunction plays a key role in increased susceptibility to bacterial infections. The impaired immune function associated with SCD places children at risk of developing life‐threatening infections, especially from Streptococcus pneumonia and Haemophilus influenzae.70,71 Long‐term penicillin prophylaxis and vaccinations are recommended; however, concerns of penicillin resistance may lead to changes.66 Children with vascular velocity >200 cm/s are at risk for central nervous system (CNS) injury (stroke). Prevention consists of chronic transfusion therapy and/or hydroxycarbamide. Pulmonary and renal complications as well as depression are other comorbidities.
Numerous nonpathognomonic oral findings have been described in SCD and include mucosal pallor (70%), delayed eruption (23%), mandibular pain (20%), osteomyelitis (5%), and discolored and depapillated tongue.72 Radiographic changes have been noted in 70%–100% of patients.73 In radiographic assessment of SCD patients, higher prevalence of external resorption; number of teeth with pulp calcification; partial and total loss of lamina dura; changes in trabecular structure of maxilla and mandible; and hypercementosis was found when compared to controls.74
Diagnosis
Premarital, antenatal, and neonatal screening programs have been established in some high‐income countries, including parts of the Middle East and the United States, and areas with a high prevalence of the disease.67 Screening consists of DNA‐based testing for prenatal diagnosis, and after‐birth diagnosis by hemoglobin electrophoresis, thin layer isoelectric focusing, solubility testing, and examination of peripheral blood smear.
Treatment
Advances in medical therapies have dramatically improved the lifespan of SCD patients due to supportive care and hydroxyurea. General measures to provide palliation and reduce the myriad of SCD complications require a multidisciplinary approach and comprehensive patient education. Hydroxyurea has been used to prevent acute pain and acute chest syndrome. Preventive measures include penicillin prophylaxis in children, Doppler screening for stroke prevention, and regular blood transfusions. Clinical trials using small‐molecule therapies are underway. A New Drug Application has been submitted to the FDA for L‐glutamine to reduce the frequency of acute pain. Hematopoietic stem cell transplantation (HSCT) is curative, but the costs associated with it are prohibitive, particularly in lower‐income populations.67 There are currently several ongoing trials with emerging treatment approaches including L‐glutamine powder, rivipansel, hydroxycarbamine, prasugrel, vepoloxamer, L‐arginine, N‐acetylcysteine, and magnesium sulfate.
Penicillin prophylaxis for children with SCD under the age of 5 years reduces infection‐related morbidity and mortality, as does appropriate vaccination against pneumonia and influenza. Vaccination with both the 13‐valent pneumococcal‐conjugated vaccine (PCV13) and pneumococcal polysaccharide vaccine PPSV23 can prevent infections by most serotypes. Acute chest syndrome is the leading cause of death. Therapy is intensive and expansive, and possible interventions include transfusion, supplemental oxygen, continued respiratory therapy, antibiotics, bronchodilators, pain management, fluid management, corticosteroids, and mechanical ventilation.
Oral Health Considerations
Measures to reduce oral disease burden and infection in the SCD patient are clearly indicated. Long‐term penicillin prophylaxis in SCD children under age 6 years inhibits the acquisition of Streptococci mutans, resulting in significantly lower caries rates in these children.75 However, within 4 years of cessation of prophylaxis, the levels of mutans streptococci reached that of matched controls. While SCD is not associated with increased periodontal disease per se,76 cases of periodontal infections77 and mandibular osteomyelitis78 precipitating a sickle cell crisis have been reported.
Given the wide variability in disease severity, it is essential that a comprehensive history and medical consultation be obtained to determine the patient’s status. During non‐crisis periods, there are no contraindications concerning the delivery of routine dental care under local anesthesia with inhalational sedation.79,80 The avoidance of using a local anesthetic agent without a vasoconstrictor is unwarranted. The need for providing antibiotic prophylaxis before rendering dental care is controversial and no clear consensus or guidance exists.80,81 Most patients can be treated safely in an outpatient setting.82 For patients deemed high risk, dental therapy may be considered in a hospital setting where appropriate medical support is readily available.
Cell Membrane Defects
Another mechanism for an absolute decrease in the number of circulating RBCs is through increased hemolysis due to membrane defects. This results in morphologic changes of the RBC, leading to cell weakness, fragility, and/or rigidity. These conditions include hereditary spherocytosis (HS), hereditary elliptocytosis (HE), pyropoikilosis (a subtype of HE resulting in severe hemolytic anemia), stomatocytosis, and paroxysmal nocturnal hemoglobinuria (PNH).83,84
HS is the most common inherited red cell membrane disorder. It occurs in 1 of every 2000–3000 individuals and is most frequently seen in Europe and North America. There is a loss of surface area on reticulocytes and RBCs due to disruption of the vertical linkages between the phospholipid bilayer and the membrane skeleton as a consequence of mutations in genes encoding for various red cell membrane proteins, including ankyrin, band 3, protein 4.2, spectrin, and RhAg 0C. HE is a red cell membrane disorder characterized by mutations in genes coding for skeletal proteins involved with horizontal protein connections, including SPTA1 and SPTB and protein 4.1R, causing the morphologic changes. It affects 3–5 per 10,000 individuals.
Stomatocytosis is a rare red cell disorder divided into two entities, xerocytosis and dehydrated hereditary stomatocytosis (DHSt) and overhydrated hereditary stomatocytosis (OHS), in which there are alterations in intracellular cation content and cell volume alterations resulting in osmotic deformities of the RBCs.84
For all of the red cell membrane diseases, the clinical manifestations are variable and the diagnosis is made based on clinical signs (pallor, jaundice, splenomegaly) and examination of the blood smear. Treatments include splenectomy, transfusions, and clinical trials of senicapoc and PIEZO1 channel inhibitors for hydration disorders.
Paroxysmal Nocturnal Hemoglobinuria
PNH is a rare acquired condition characterized by episodes of hemolysis, bone marrow failure, thrombosis, and smooth muscle dystonia. It affects 1–1.5 cases per million worldwide.85 Patients with PNH are deficient in proteins that bind to the cell surface through a glycosylphosphatidylinositol (GPI) anchor due to mutations in the phosphatidylinositol glycan anchor biosynthesis class A gene (PIGA) gene. Several of these proteins (CD55, CD59) are complement‐regulatory proteins, and treatment with a humanized monoclonal antibody that inhibits terminal complement activation such as eculizumab can ameliorate hemolysis and symptoms. Many cells contain GPI anchors, but since erythrocytes lack a nucleus, they are more susceptible to lysis. Clonal expansion of HSCs harboring somatic mutations in the X‐linked gene renders lineage cells vulnerable to complement‐mediated intravascular and extravascular hemolysis. Intravascular hemolysis leads to release of free hemoglobin, a potent scavenger of nitric oxide. The subsequent lack of tissue nitric oxide is believed to underlie many of the clinical manifestations of PNH, including fatigue, pain, esophageal spasm, erectile dysfunction, and possibly platelet activation. Patients with classic PNH are at increased risk for thrombosis and other complications of intravascular hemolysis. Without therapy, the median survival rate is 10–20 years.86
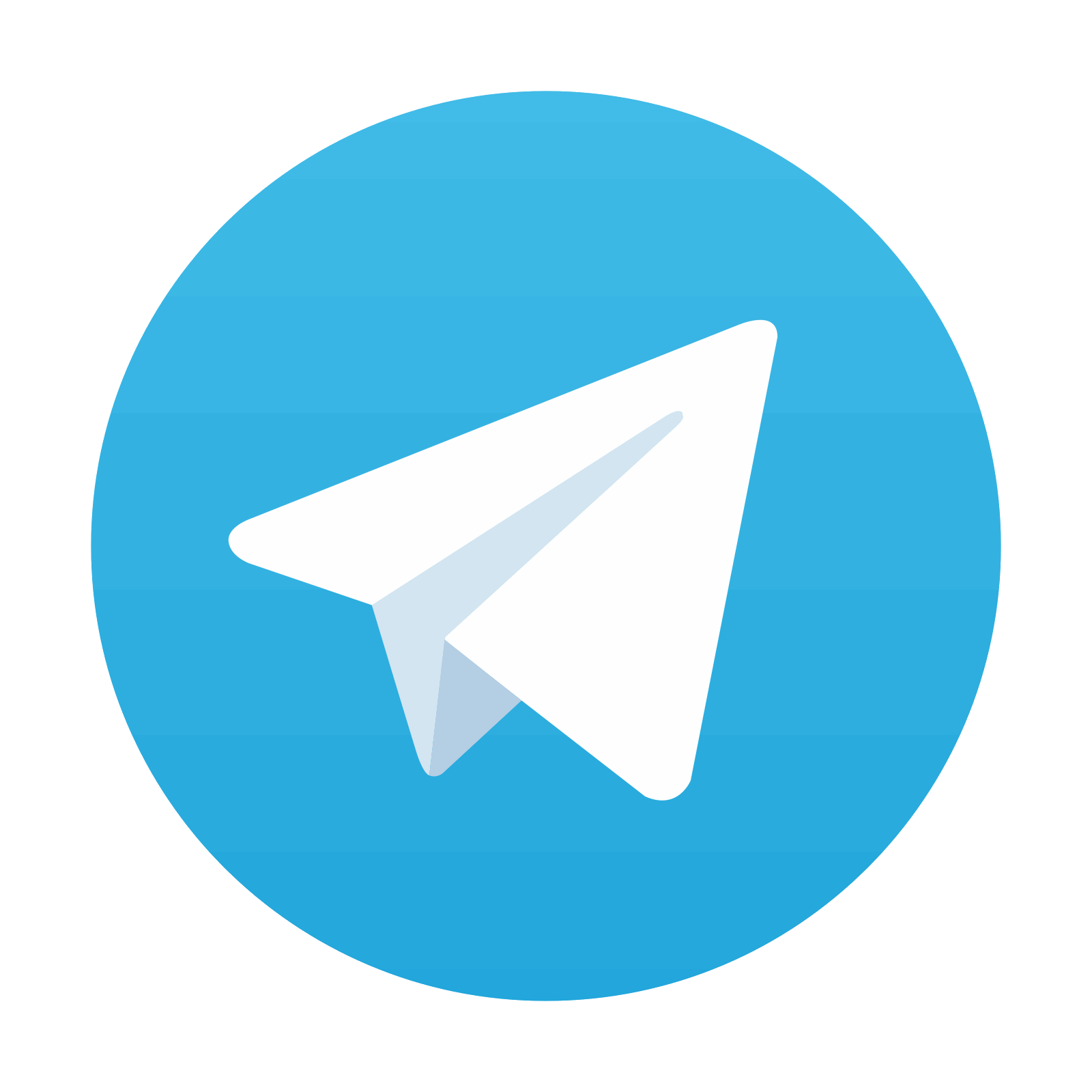
Stay updated, free dental videos. Join our Telegram channel

VIDEdental - Online dental courses
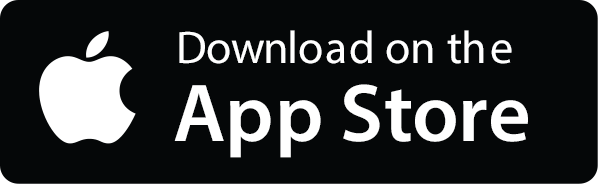
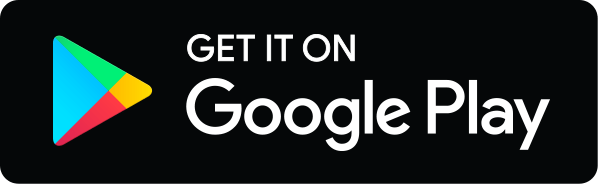