Introduction
Extreme patterns of vertical facial divergence are of great importance to clinicians because of their association with dental malocclusion and functional problems of the orofacial complex. Understanding the growth patterns associated with vertical facial divergence is critical for clinicians to provide optimal treatment. This study evaluates and compares growth patterns from childhood to adulthood among 3 classifications of vertical facial divergence using longitudinal, lateral cephalograms from the Craniofacial Growth Consortium Study.
Methods
Participants (183 females, 188 males) were classified into 1 of 3 facial types on the basis of their adult mandibular plane angle (MPA): hyperdivergent (MPA >39°; n = 40), normodivergent (28° ≤ MPA ≤ 39°; n = 216), and hypodivergent (MPA <28°; n = 115). Each individual had 5 cephalograms between ages 6 and 20 years. A set of 36 cephalometric landmarks were digitized on each cephalogram. Landmark configurations were superimposed to align 5 homologous landmarks of the anterior cranial base and scaled to unit centroid size. Growth trajectories were calculated using multivariate regression for each facial type and sex combination.
Results
Divergent growth trajectories were identified among facial types, finding more similarities in normodivergent and hypodivergent growth patterns than either share with the hyperdivergent group. Through the use of geometric morphometric methods, new patterns of facial growth related to vertical facial divergence were identified. Hyperdivergent growth exhibits a downward rotation of the maxillomandibular complex relative to the anterior cranial base, in addition to the increased relative growth of the lower anterior face. Conversely, normodivergent and hypodivergent groups exhibit stable positioning of the maxilla relative to the anterior cranial base, with the forward rotation of the mandible. Furthermore, the hyperdivergent maxilla and mandible become relatively shorter and posteriorly positioned with age compared with the other groups.
Conclusions
This study demonstrates how hyperdivergent growth, particularly restricted growth and positioning of the maxilla, results in a higher potential risk for Class II malocclusion. Future work will investigate growth patterns within each classification of facial divergence.
Highlights
- •
Understanding vertical facial divergence is critical to treatment optimization.
- •
Growth in 3 vertical facial types is quantified with geometric morphometrics.
- •
Normodivergent and hypodivergent growth are distinct from hyperdivergent growth.
- •
Anteroposterior maxillomandibular growth is restricted in hyperdivergent patients.
- •
Hyperdivergent growth patterns indicate a greater risk for Class II malocclusion.
Classifications of facial and dental morphology are frequently used in orthodontic practice for diagnosis, treatment planning, and prognosis. Schudy first characterized the interaction of vertical and anteroposterior growth in the face as patterns of facial divergence (ie, hyperdivergent, normodivergent, and hypodivergent), and since then, additional terminology has been used to describe similar morphologic patterns. The hyperdivergent skeletal pattern has been referred to as an open bite or long face syndrome, and the hypodivergent pattern as a deep bite or short face syndrome. , Although Schudy’s classification system was based on the mandibular plane angle (MPA), other measurements have been used to classify patterns of vertical facial divergence, including the anterior to posterior facial height ratio, the lower anterior to total anterior facial height ratio, and the angle between mandibular and Frankfort horizontal planes.
The clinical significance of these classifications relates to their associations with dental malocclusion and other functional or esthetic issues. The hyperdivergent facial type is commonly associated with an anterior open bite referring to a lack of vertical overlap in maxillary and mandibular incisors. Furthermore, skeletal hyperdivergence is related to reduced masticatory performance and airway constriction. The hypodivergent facial type has been linked to a deep or excessive vertical overbite of the maxillary and mandibular incisors, which can interfere with lateral and anterior mandibular movements and temporomandibular joint dysfunction. ,
Understanding differences in morphology and growth associated with vertical facial divergence patterns is critical for providing appropriate treatment and prognoses for participants. Morphologic differences among skeletal facial types are apparent around 5 or 6 years of age. , Most studies agree that facial type differences are predominantly found in the lower face and mandible, but the specific measurements that differ among facial types vary by study. , , ,
Previous cephalometric analyses of linear distance and angular measurements describing childhood and/or adolescent growth patterns across facial types have also found contrasting results. For example, Bishara and Jakobsen note parallel incremental growth curves for many craniofacial measurements from 5 to 25 years of age, indicating measurement differences among facial types were already present at 5 years of age, with similar subsequent growth patterns. Conversely, Karlsen , reported differences in growth up to 12 years of age among facial types, whereas Nanda found variation in the adolescent growth spurt among facial types leading to increased measurement differences during adolescence. These disagreements likely result from the use of different study samples, classification methods, or the age at classification, requiring further work to harmonize previous studies.
In this analysis, we use geometric morphometric methods (GMM), a multivariate, landmark-based approach to evaluate differences in craniofacial morphology and growth patterns among 3 skeletal facial types. The GMM approach allows for the evaluation of coordinated patterns of vertical and anteroposterior growth in craniofacial structures by preserving the geometric or spatial relationships of landmarks throughout the analysis, which traditional linear cephalometrics are poorly equipped to quantify. We used the strengths of this approach to clarify inconsistencies among previous studies of craniofacial growth patterns of hyperdivergent, normodivergent, and hypodivergent participants. The goals of this study are to (1) describe and compare growth trajectories for hyperdivergent, normodivergent, and hypodivergent facial types and (2) determine shape differences among facial types at different ages.
Material and methods
The Craniofacial Growth Consortium Study houses longitudinal, lateral cephalograms from 6 historical growth studies in the United States, which sampled populations of primarily European ancestry from 1930-1982. , , A total of 1855 cephalograms from 371 participants (183 females, 188 males) in the Craniofacial Growth Consortium Study were included in this analysis. Each individual had 1 cephalogram taken within each of the following age categories: 6-8, 9-11, 12-14, 15-17, and 18-20 years, for a total of 5 cephalograms per participant. If a participant had more than 1 cephalogram per age category, the cephalogram taken closest to the median age for that category was selected.
The age range of 6-20 years, and associated age categories, were chosen to maximize the available sample, which includes the primary period for clinical treatment related to facial growth. Some previous studies have considered the importance of postadolescent changes in facial dimensions, although the amount and clinical significance of this growth have been debated. , , The greatest magnitude of postadolescent growth is often observed in anterior facial height but rarely exceeds 1 mm over 10 years, a suggested threshold for clinical significance.
Facial type classification was based on adult morphology for all participants using their cephalograms in the 18-20 year age interval; these classifications were applied to juvenile cephalograms from the same participants. MPA, the angle between sella-nasion and gonion-menton, was calculated and used for facial type classification: hypodivergent (MPA <28°), normodivergent (28° ≤ MPA ≤ 39°), and hyperdivergent (MPA >39°) ( Table I ). A set of 36 cephalometric landmarks ( Table II ; Fig 1 ) was collected on each cephalogram, with no missing data, to quantify the morphology of the craniofacial skeleton and anterior dentition. This study was approved by the University of Missouri Institutional Review Board.
Classification | Females | Males | ||
---|---|---|---|---|
Participants | Cephalograms | Participants | Cephalograms | |
Hyperdivergent | 21 | 105 | 19 | 95 |
Normodivergent | 113 | 565 | 103 | 515 |
Hypodivergent | 49 | 245 | 66 | 330 |
Total | 183 | 915 | 188 | 940 |
No. | Landmark | Definition |
---|---|---|
1 | SELLA | The midpoint of the pituitary fossa |
2 | NASION | The most anteroinferior point on the frontal bone at the nasofrontal suture |
3 | ORBITALE | Inferiormost point of the orbital margin of the more anterior orbital image |
4 | U I APEX | Point of intersection between the long axis of the more anteriorly positioned maxillary central incisor and the contour of that tooth’s root-end curvature |
5 | POINT A | The deepest point on the curvature of the surface of the maxillary bone between ANS and the alveolar crest of the maxillary central incisor |
6 | U I EDGE | Tip of the incisal edge of the more anteriorly placed maxillary central incisor |
7 | L I EDGE | Tip of the incisal edge of the more anteriorly placed mandibular central incisor |
8 | POINT B | The deepest point on the curvature of the anterior border of the mandible between pogonion and the alveolar crest of the mandibular central incisor |
9 | L I APEX | Point of intersection between the long axis of the most anteriorly positioned mandibular incisor and the contour of that tooth’s root-end curvature |
10 | POGONION | Anterior-most point of the bony chin at the midline |
11 | MENTON | Inferiormost point on the mandible at the symphysis |
12 | CONDYLE | The point on the posterior-superior contour of the condyle that is the longest distance from pogonion |
13 | PNS | The intersection between the posterior extension of the superior surface of the palate and the downward extension of the pterygomaxillary fissure |
14 | BASION | Inferiormost point on the anterior margin of the foramen magnum in the midsagittal plane |
15 | PORION | Most superior point of the external auditory meatus of the right ear |
16 | ANS | Anterior-most point of the anatomic anterior nasal spine |
17 | GLABELLA | The most anterior point on the osseous forehead |
18 | SE ∗ | The midpoint between the intersections of the 2 great wings of the sphenoid bone with the sphenoid plane |
19 | POINT P ∗ | Point of greatest convexity between the anterior contour of sella turcica and the sphenoid plane |
20 | PTM | Pterygomaxillary fissure point: the intersection of the inferior border of the foramen rotundum with the posterior wall of the pterygomaxillary fissure |
21 | SIGMOID | The deepest point of the sigmoid notch |
22 | P RAMUS | Posterior ramus point where the inflection starts |
23 | CORONOID | Tip of the coronoid process |
24 | A RAMUS | The deepest point in the anterior ramus |
25 | INFRADENTALE | Most superior and anterior point of the mandibular alveolar process |
26 | LINGUAL L1 | Most superior and anterior point of alveolar bone on the lingual surface |
27 | LINGUAL POINT B | Intersecting point of a posterior extension of point B parallel to the mandibular plane connecting gonion and menton on the lingual cortical plate of the symphysis |
28 | L SYMPHY | Intersecting point of a posterior extension of pogonion parallel to the mandibular plane connecting gonion and menton on the lingual cortical plate of the symphysis |
29 | ANTEGONION | The deepest point of the antegonial notch |
30 | PROSTHION | Most inferior and anterior point of the maxillary alveolar process |
31 | FRONT | Point of intersection between the roof of orbit line and the internal cortical plate of the frontal bone |
32 | ROOF ∗ | Most superior point of the roof of the orbit, the middle point between FRONT and SE-S |
33 | SE-S ∗ | Point of intersection of the greater wing of the sphenoid bone and the roof of the orbit (midpoint between 2 greater wings of the sphenoid bone) |
34 | ETHMOID ∗ | Point of intersection between the lateral border of the ethmoid bone and the vertical extension of the most anteriorly positioned Key ridge |
35 | ZYGOMA | Most inferior point of the most anteriorly positioned key ridge |
36 | GONION | Average of upper and lower gonion points |

For each sex, a modified generalized Procrustes analysis was used to align landmark configurations by removing variation in location, rotation, and scale so that only differences in shape remained. , Each landmark configuration was superimposed to align 5 homologous landmarks of the anterior cranial base but scaled to the unit centroid size of the entire landmark configuration (see Table II ; Fig 1 ). The resulting aligned landmark coordinates were used as shape variables in subsequent analyses.
To model growth trajectories, multivariate regressions of the shape variables on age , were performed for each adult facial type, separately by sex. In this context, we specifically refer to growth trajectories as changes in landmark configurations (ie, shape change) with age. For each of the 6 facial type and sex combinations, 4 different models were fit (linear to fourth-order polynomial). Leave-one-out cross-validation and mean squared error values were used to select the best fit models. Predicted shapes were calculated for the 6 selected models at 1-year age intervals, from 6-20 years, to represent annual mean shape estimates for each trajectory. Pairwise permutation tests, described by Mitteroecker et al, were used to test identical trajectories among the 3 facial types using the residual sum of squares as a test statistic with 10,000 iterations.
Facial type growth trajectories were compared, by sex, using a principal component analysis of age-shape space, which is a principal component analysis of the matrix of predicted shape coordinates with an additional column for age (cf, Mitteroecker et al ). Growth patterns for each facial type were visualized using wireframe plots to compare the shape change within each trajectory at ages 6, 10, 14, and 18 years. Comparisons were also made between the hyperdivergent and hypodivergent landmark configurations at these ages to visualize morphologic differences between the most extreme facial types.
Three angular measurements, SNA (sella-nasion-point A), SNB (sella-nasion-point B), and ANB (point A-nasion-point B), were calculated for each cephalogram to demonstrate the change in anteroposterior (A-P) maxillomandibular relationships with age, across facial types. Although relative maxillomandibular relationships are visualized using GMM, the SNA, SNB, and ANB angles are provided for comparison as clinically important cephalometric measurements for identifying interactions with anteroposterior relationships (ie, Class I, II, and III). One-way analysis of variance with post-hoc Tukey’s Honest Significant Difference test was used to test significant differences in each angle among facial types by sex and age group. All analyses were performed in R using the Morpho, geomorph, and stats packages.
Results
For each of the 6 facial type and sex combinations, quadratic models were selected for multivariate regressions of shape variables on age ( Table III ). In females and males, the first principal component axis of age-shape space describes variation in age and patterns of age-correlated shape. When plotted against age, the second principal component axis distinguishes hyperdivergent and hypodivergent trajectories, with the normodivergent trajectory between them ( Fig 2 ). The differences in growth trajectories represent unique patterns of average craniofacial growth for each facial type. In females, the 3 trajectories become increasingly parallel at older ages, whereas, in males, the hyperdivergent and hypodivergent trajectories continue to diverge from the normodivergent trajectory. Raw data for each individual are projected onto the age-shape axes to visualize the individual variation within each facial type ( Fig 2 ). Individual trajectories overlap among facial types, but permutation tests indicate significantly different trajectories for each facial type ( P ≤0.0003 for all comparisons) ( Table IV ).
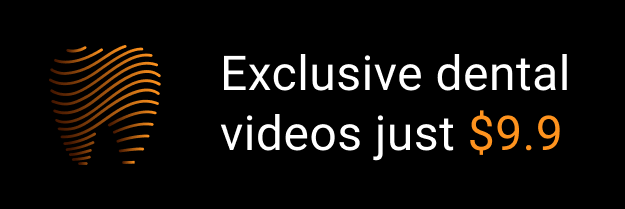