General principles of metabolism
Publisher Summary
This chapter focuses on the general principles of metabolism. Once the food has been digested and absorbed, the various nutrients are distributed to the tissues via the blood and, having passed through the cell membranes, are exposed to the metabolic machinery of the cell. The aim of this machinery is to release some of the energy contained in the nutrients and convert it into a form that can be used for the various functions of the cell, and to use the rest of the material for the synthesis of substances that the body needs for its growth and activities. To do this, the food materials are subjected to a variety of metabolic processes, each of which involves a well-defined sequence of reactions. Nearly every step is catalyzed by a different enzyme and results in a small but specific chemical change. This field of intermediary metabolism constitutes a major part of biochemistry. Metabolism may be divided into three areas. Catabolism deals with the breakdown of materials with the release of energy. Anabolism covers the processes by which complex substances are built up from simple precursors, and this utilizes much of the energy released during the course of catabolism. Between the clearly defined anabolic and catabolic pathways lies a central area of metabolism in which various simple compounds are interconverted.
1. Chemical syntheses and the process of growth in which complex, highly organized molecules are built and assembled from small molecular precursors.
2. The maintenance of the highly ordered structure of the cell.
3. Transport and the movement of materials against a concentration gradient, i.e. from a region of lower to one of higher concentration.
4. Mechanical work, e.g. the contraction of muscles and movement of cilia.
According to the Second Law of Thermodynamics, any organized collection of matter tends towards a state of maximum randomness or disorder. It is this tendency that determines the direction in which energy will move within a system which is not already in equilibrium. For example, the ordered condition of a gas at high pressure in contact with a gas at low pressure will not persist; gas will flow from the high pressure region to the low pressure region thereby replacing an ordered state by a random one. As explained in Chapter 16, similar considerations govern the direction in which chemical reactions will proceed, the tendency which any given reactants have to form products being expressed by the equilibrium constant (Keq). Reactions occur because the final state is more random than the initial state. This randomness is called entropy, and another way of expressing the Second Law of Thermodynamics is to say that all systems tend towards maximum entropy. Living systems are highly ordered, so that their development, at first sight, appears to be in direct conflict with this law. The localized ordering of living systems is achieved by increasing the randomness of their surroundings since a decrease in entropy (increase in orderliness) can occur provided that it is coupled with the release of energy and the overall ΔG0 is negative. Consequently considerably more energy must always be removed from the environment than is incorporated into the living organism.
Extraction of energy from the environment
Cells consist of about 70% by weight of water, so that all cellular reactions take place in a predominantly aqueous environment. The conditions therefore are quite different from those under which man-made machines operate. Furthermore, living cells can only survive if both the temperature and the pressure are maintained within a narrow range. As in the case of heat engines, when fuel is burnt oxygen is used up and carbon dioxide is produced; but in the body the energy is released in a controlled manner which makes it possible for a large part of the energy to be utilized for the synthesis of the special carrier of free energy, adenosine triphosphate (ATP), from its precursor adenosine diphosphate (ADP). When 1 mole of glucose is oxidized to carbon dioxide and water, 2878 kJ (688 kcal) of energy are released, whereas only 29–38 kJ (7–9 kcal) are required to convert 1 mole of ADP to ATP. These two quantities of energy are altogether disproportionate but by breaking down the glucose in a large number of small steps, the amount of energy released during certain of the reactions is converted to the same order of magnitude as that required for the formation on one high-energy bond (page 211). Even so, only about half of the energy released during the breakdown of foodstuffs is conserved in the form of ATP, the remainder appearing as heat. However, since the body is equipped with efficient temperature-regulating mechanisms, the heat is transferred to the surroundings with no appreciable rise in body temperature.
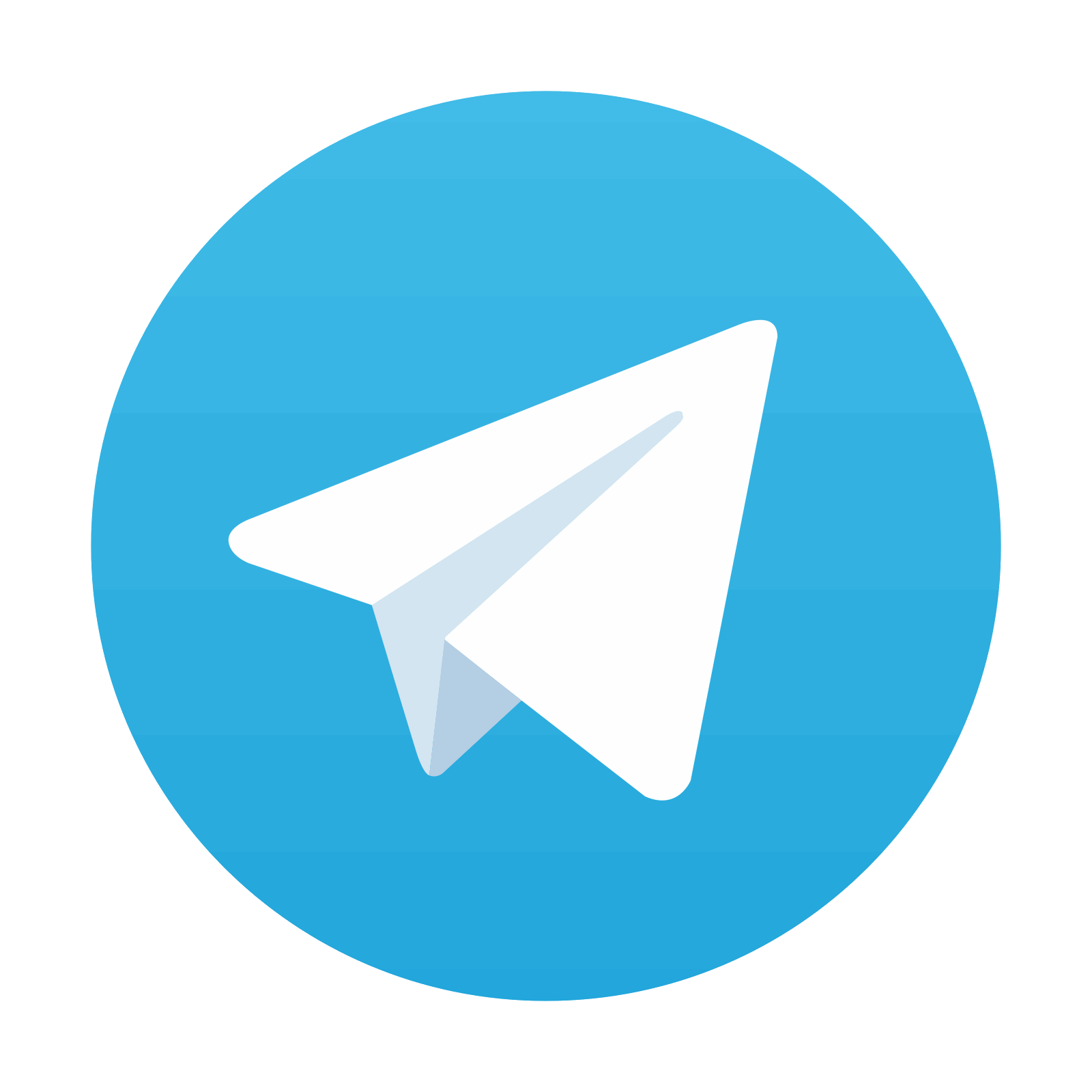
Stay updated, free dental videos. Join our Telegram channel

VIDEdental - Online dental courses
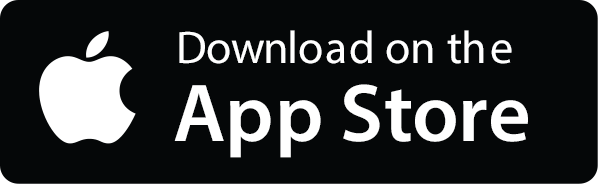
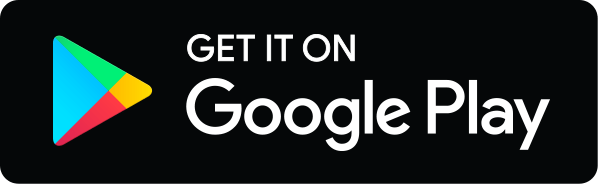