Abstract
Purpose
Test the hypothesis that a self-etching adhesive is more likely to fail at the dentin-adhesive interface than an etch-and-rinse adhesive.
Materials and methods
Forty-eight composite-dentin short rod chevron-notched specimens were prepared. XP Bond and G Bond were used as adhesives. After 7 days in distilled water at 37 °C, each specimen was tested (cross-head speed = 0.05 mm/min). Fractured surfaces were inspected and characterized as interfacial failures, composite failures or a combination of interfacial and composite failures. The fracture toughness values ( K IC ) of the two adhesives were compared (Student’s t -test and Weibull statistics).
Results
Of the specimens bonded with XP Bond, 50% failed at the dentin-adhesive interface, 42% at both the dentin-adhesive and composite interface and 8% in the composite alone. Of the specimens bonded with G Bond, 41% failed at the dentin-adhesive interface, 53% at both the dentin-adhesive and composite interface and 6% in the composite alone. The K IC values of the two adhesives differed significantly ( p < 0.05). XP Bond had a K IC of 0.77 ± 0.11 MNm −3/2 ( n = 17), while G Bond a K IC of 0.62 ± 0.21 MNm −3/2 ( n = 12).
Conclusion
The high percentage of mixed failures did not support the hypothesis that the dentin-adhesive interface is clearly less resistant to fracture than the adhesive–composite interface. The finding that cracks occurred in 6–8% in the composite suggests that defects within the composite or at the adhesive–composite interface are important variables to consider in adhesion testing.
1
Introduction
Most recorded “bond strength” values are likely not the highest stress the adhesive can carry before it fails. The reason is that “bond strength” values are usually generated by loading a sample in tension or in shear until failure occurs. The strength value is then determined by dividing the force value at failure by cross sectional area. A major flaw with such an approach is the assumption that the stress is evenly distributed over the entire cross sectional area when failure occurs. In reality, that is not the case because of differences in modulus of elasticity and differences in Poisson’s ratios of the different materials. These differences cause unequal cross-contractions in the adhesive region resulting in a quite complex stress field in the bonded region . Stress analyses have showed that the highest stresses occur in the periphery of the adhesive layer . In addition, flaws are also likely to be present in these high local stress regions, particularly if the samples have been cut or machined. These conditions suggest that the failure will start in these regions and propagate toward the central regions of the sample, which has also been shown on SEM pictures .
Considering the above arguments, failures do not occur instantaneously over the entire surface and are not due to a stress equal to the overall force divided by the cross sectional area. Instead, failures are triggered by substantially higher localized stress and locally formed cracks propagate along a path of lowest energy requirement to break the bonds . Such a least work-dependant behavior may result in a crack growth that even leads the crack into another material if that material is more favorable from an energetic point of view.
By using a fracture mechanics approach rather than focusing on a strength property, the energetic conditions become the most important variables to consider . In dentistry, fracture mechanics has been used in some studies to determine the fracture toughness of composites bonded to dentin . Unfortunately, these approaches have not been used as extensively as traditional strength tests, probably because they usually require more complex sample preparation techniques . However, despite their complexity, fracture toughness determinations have the advantage of recognizing that cracks propagate in a way that is most favorable from an energetic point of view.
Because fracture toughness determinations result in crack growth formation along the least energy requiring path, we decided to use a fracture toughness approach described by Tam and Pilliar to determine how cracks propagate at a composite–adhesive-dentin interface and use Weibull statistics to interpret the data . The reason Weibull statistics was considered was that fracture toughness studies of dentin adhesives have showed that the standard deviation value of a dentin adhesive is often 20–30% of the mean value. A possible explanation for such variability could be differences in flaw size distributions among specimens. According to Dickens and Cho , Weibull analyses reflect the flaw size distributions in specimens. Considering that Weibull analyses are based on Weibull’s statistical theory of the strength of materials , we felt it could be appropriate to use Weibull statistics to analyze our results.
The hypothesis we decided to test was that failures start easier at the dentin-adhesive interface of a self-etching system than with an etch-and-rinse system. The less aggressive etching achieved with a self-etching adhesive would simply result in a more superficial resin–dentin infiltration and possibly also a weaker mechanical retention at the resin–dentin interface.
2
Materials and methods
2.1
Dentin specimen preparation
Coronal dentin disks (∼1.0 mm thick) were cut perpendicularly to the long axis of molars by use of a water-cooled diamond disk. To control the thickness of the dentin, an iron block with a 0.85 mm deep cavity, sufficient large for the dentin disks, was used to trim the disks down to a thickness of 0.85 mm with an emery paper (600 grit) ( Fig. 1 ).
Throughout the cutting and thickness trimming process, water was used to keep each specimen wet and cool in order to prevent the dentin from degrading. Forty-eight specimens were selected based on quality and used in the study. These disks were then trimmed into rectangular 7 mm × 4 mm dentin specimens, and the long edges were slightly beveled to fit securely into a polyethylene specimen mold ( Fig. 2 ).
2.2
Short-rod chevron-notch specimen preparation
One of the large rectangular dentin surfaces of each of the 48 specimens was etched with 35% phosphoric acid for 15 s, rinsed under tap water for 20 s, blot-dried, coated with XP Bond ( Table 1 ), blown with a light air pressure for 5 s, and light-cured for 10 s. Light curing was performed with an LED light (Heraeus Kulzer, Ladestation, Translux Power Blue); the irradiance was approximately 1050 ± 50 mW/cm 2 throughout the experiment, as measured by a curing radiometer (Dentsply Caulk Cure Rite Visible Curing Light Meter; Model No. 644726; Milford, DE). After placing ∼1 mm thick layer of composite (Venus, Table 1 ) in a half cylindrical polyethylene mold, the dentin disk with the XP Bond coated surface was also placed in the mold, forming a half cylinder ( Fig. 3 ).
Resin composite |
1 Venus color adaptive matrix universal light curing composite, lot # 010118, color A2, 20 sec |
Dental adhesives |
1 G-Bond (One component self-etching light-cured adhesive): lot #0802151 |
2 XP Bond (universal total-etch adhesive): lot #0706002047 |
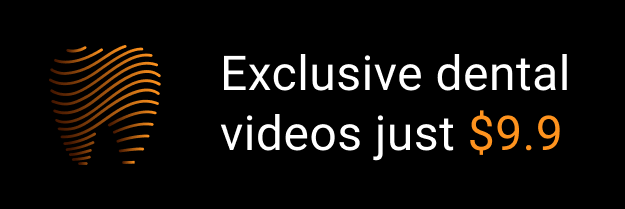