Abstract
Objectives
This study aims to evaluate the fracture load of differently fabricated 3-unit posterior fixed dental prostheses (FDPs) with an intermediate pontic.
Methods
Fifty sets of two stainless-steel abutments were randomly assigned to five groups ( n = 10 each) depending on the material and technique used for manufacturing the FDPs: (1) Metal-ceramic (MC, control); (2) Lava Zirconia (LZ, bi-layered); (3) Lava Plus (LM, monolithic); (4) VITA In-Ceram YZ (YZ, bi-layered); and (5) IPS e-max ZirCAD (ZZ, bi-layered). After being luted to the dies, all FDPs were submitted to thermo-mechanical cycling (120,000 masticatory cycles, 50 N; plus 774 thermal cycles of 5 °C/55 °C, dwell time: 30 s). Samples were then subjected to a three-point bending test until fracture in a universal testing machine (cross-head speed: 1 mm/min). Fracture load of the veneering ceramic (VF) and total fracture load (TF) were recorded. Microstructure and failure patterns were assessed. Data was analysed using one-way ANOVA and Tukey HSD post-hoc tests ( α = 0.05).
Results
MC restorations recorded higher VF and TF values than did zirconia FDPs ( p = 0.0001), which showed no between-group differences. Within the bi-layered groups, TF was significantly higher than VF. LM pieces registered lower average grain size than did LZ specimens ( p = 0.001). Overall, the connector was the weakest part.
Conclusions
All of the groups tested could withstand clinical chewing forces in terms of average fracture load. Zirconia-based samples performed similarly to each other, but showed lower mean fracture load values than did metal-ceramic ones.
Clinical significance
Monolithic zirconia may be recommendable for solving the chipping problem.
1
Introduction
Metal-ceramic fixed dental prostheses (FDPs) have widely been used since the early 1960s, showing good success rates . However, given the request for more aesthetic and biocompatible treatments , from the late 1990s, the research has been focused on CAD/CAM (Computer Aided Design/Computer Aided Manufacturing) high-strength ceramic systems for achieving improved metal-free prosthetic solutions .
Yttrium-oxide partially-stabilised zirconia (Y-TZP) has become an attractive high-toughness core material for posterior FDPs, as it may withstand occlusal forces in the molar region . Zirconia (also called ‘ceramic-steel’) has a completely crystalline microstructure, a stress-induced toughening (‘transformation toughening’) mechanism and physical features that are similar to those of stainless-steel . However, it generally requires to be veneered with glass ceramic for obtaining proper aesthetics .
Regrettably, the ‘chipping’ of the veneering porcelain creates an uncertainly as regards the durability of zirconia-based restorations . Minor chipping, consisting of little fractures, can be polished or repaired with composite resin. Conversely, major chipping of larger ruptures involving functional areas that would lead to a significant alteration of the anatomy after adjustments is considered ‘failure’, as the only option is the prosthesis’ replacement . Several efforts have been made to reinforce the fracture strength of the veneering porcelain, such as the use of high-density CAD/CAM ceramic , press ceramic , or the ‘Rapid Layer Manufacturing’ (RLM) technique that individually fabricates the CAD/CAM core and the veneer layers and applies low-fusing glass ceramic or resin-cement to bond the interface .
Monolithic zirconia was introduced to circumvent the drawbacks of bi-layered all-ceramic prostheses , although its chemical stability and long-term clinical behaviour still remain insufficiently documented. Moreover, there is very little research on the comparison of the fracture load of different zirconia systems and the selection criteria are usually based on personal preferences and experience rather than on sound scientific rationale. FDPs are subjected to complex clinical conditions that induce temporary deformations and internal stresses within the materials and their interfaces . For instance, water can act chemically at crack tips and decrease the ceramic strength, thus having influence on cyclic and static loading tests . Simulated moisture, thermal and mechanical fatigue may therefore approximate the influence of the oral environment before compressive static forces are applied to record the fracture load of the tested materials by means of a three-point bending test .
Hence, the aim of this paper is to evaluate the fracture load of the veneering ceramic (VF), the fracture load of the complete restoration or ‘total fracture load’ (TF), and the fracture pattern of monolithic and bi-layered zirconia-based 3-unit posterior FDPs after thermo-mechanical cycling using a metal-ceramic group as control. Recently marketed CAD/CAM technologies were tested in order to identify the most resistant system. No previous study has compared such parameters between monolithic and veneered zirconia FDPs after thermal and mechanical fatigue followed by three-point bending flexural test.
The null hypotheses tested were that (1) the fracture load values (VF and TF) did not depend on the restorations’ material and manufacturing technique, and that (2) VF and TF values coincided within each bi-layered method.
2
Materials and methods
2.1
Fabrication of the restorations
A hundred standardised stainless-steel master dies that simulated premolars prepared to be retainers of all-ceramic FDPs were fabricated. The abutments’ configuration was: 5 mm in height; convergence of 6°; chamfer of 120°, 1 mm in width and 8 mm in diameter; axial reduction of 1 mm; vestibular and lingual cuspids separated by a central groove of 1 mm in depth and rounded angles . The dies were randomly screwed in pairs onto fifty metallic bases customised as follows: 4.5 mm in height, 30 mm in length, 17 mm in width and two centred perforations separated by 7 mm.
The fifty sets of samples formed by the abutments fixed onto their respective bases were sandblasted with 100 μ m-alumina particles to eliminate the surface brightness and facilitate the scanning procedures and were randomly assigned to five groups ( n = 10 each) depending on the material and manufacturing technique to be used for constructing 3-unit posterior FDPs with an intermediate pontic: MC: Cast metal-ceramic (control); LZ: Lava Zirconia (3 M ESPE, Seefeld, Germany); LM: Lava Plus (3 M ESPE); YZ: VITA In-Ceram YZ (Vita Zahnfabrik, Bad Säckingen, Germany) and ZZ: IPS e.max ZirCAD (Ivoclar Vivadent, Schäan, Liechtenstein).
The LM group consisted of monolithic zirconia (ZrO 2 ) pieces, while the remaining zirconia groups, i.e., LZ, YZ, and ZZ were bi-layered restorations of yttrium-stabilised zirconium oxide frameworks veneered with glass ceramic. To date, the specific chemical identity and/or exact composition of the polycrystalline zirconia frames has frequently been withheld as a trade secret. Nonetheless, as a reference, the YZ cores include 91–94% of ZrO 2 , 4–6% of Y 2 O 3 , 2–4% of HFO 2 , and less than 0.1% of Al 2 O 3 , SiO 2 , and Na 2 O. The same occurs with the composition of the veneering porcelain. However, in all cases (MC, LZ, YZ, and ZZ), the coating material were feldspathic ceramic (or ‘glass ceramic’) and consisted almost entirely of an amorphous glass phase with crystalline constituents such as leucite crystallites. All FDPs were obtained according to the manufacturers’ instructions.
The restorations of the MC group (control) were fabricated using the traditional lost-wax casting technique. A base metal alloy of white Co-Cr for ceramics was selected (Ugirex C; Ugin Dentaire, Seyssinet-Paris, France). The alloy composition was: Co: 62%; Cr: 31%; Mo: 4%; and Si: 2.2%. The patterns of the structures were waxed-up onto the metallic abutments, which were varnished with three layers of die spacer beforehand to leave gap of 50 μ m for the luting material. The frameworks were modelled with a wall-thickness of 0.5 mm and round-shaped connectors of 9 mm 2 , and were invested. The MC structures were cast in an induction heated vacuum pressure machine (Jelrus Infinity L30; Whip Mix, Dortmund, Germany), at 1470 °C. The casts were then retrieved and cleaned using airborne-particle abrasion with alumina (Al 2 O 3 ) powders (125 μ m) for 10 s at a working distance of 5 mm and a pressure of 2 bars.
For the oxidation of the divested structures, two thin layers of opaque porcelain (Omega 900; VITA Zahnfabrik, Bad Säckingen, Germany) were applied to the metal surfaces and were fired to 900 °C under vacuum in a calibrated ceramic oven (Programat P500/G2; Ivoclar-Vivadent AG, Schäan, Liechtenstein). The metallic cores were then veneered with dental and incisal compatible glass ceramic (Omega 900; VITA Zahnfabrik) at 910 °C. Finally, the glazing procedure was carried out at 915 °C.
The LZ, LM, YZ, and ZZ samples were prepared by an experienced technician who was accustomed to work with the CAD/CAM systems tested. The fabrication of the Y-TZP structures started by scanning and digitizing each pair of abutments with a specific non-contact, optical scanner depending on the study group: Lava Scan (band projection/triangulation, precision in the range of 25 μ m; 3 M ESPE, Seefeld, Germany) for LZ and LM groups; and InEos X5 Scanner (5-axis technology with automatic positioning combined with auto focus, precision <12 μ m; Sirona Dental, Bensheim, Germany) for YZ and ZZ samples. A 3-D model was generated in each scanning cycle by using the next packages software for Windows: Lava System 3.01 CAD (3 M ESPE) for LZ and LM specimens and Sirona inLab 3D (Sirona Dental) for YZ and ZZ groups.
The following parameters were programmed with the abovementioned software. For the bi-layered groups (LZ, YZ, and ZZ): wall thickness of 0.5 mm in each plane. For the monolithic group (LM): total thickness of 1 mm at the axial walls and 2.5 mm at the occlusal surfaces. In all zirconia groups: an internal space of 50 μ m for the luting agent and rounded connectors of 9 mm 2 . An enlargement of 20% was envisioned in all zirconia specimens to offset the shrinkage derived from the final sintering procedure that permitted zirconia pieces to achieve an increased density at their final size .
Pre-sintered CAD/CAM blocks of Y-TZP that were compatible with each system were selected to mechanise the samples in the respective milling units, i.e., Lava Form (3 M ESPE) for the LZ group (obtaining milled structures) and LM group (obtaining milled FDPs with their final shape); and Sirona inLab (Sirona Dental) for the YZ and ZZ groups (obtaining milled structures). The sintering procedures of the enlarged pieces lasted for 8 h and were accomplished in specific ovens at high temperatures: Lava Therm (3 M ESPE) at 1500 °C for the LZ and LM groups; and VITA Zyrcomat (VITA Zahnfabrik) at 1530 °C for the YZ and ZZ groups.
The zirconia structures of the bi-layered groups were veneered in a specific oven (Programat P500/G2 M; Ivoclar Vivadent) with compatible hand-layered feldsphatic ceramic.
In the case of the LZ frameworks, a 0.1/0.2-mm film of liner (Zirconia Overlay Porcelain for Lava Frame; 3 M ESPE) was applied and submitted to a firing cycle at 820 °C. The structures were then covered with the corresponding ceramic (Lava Ceram; 3 M ESPE). 3 The first layer of dentine was submitted to 810 °C, while both the second layer of dentine and the incisal coating were fired at 800 °C. The glazing procedure was made at 790 °C.
With respect to the YZ structures a 0.1/0.2-mm layer of liner (VITA VM9 Effect Bonder; VITA Zahnfabrik) was spread with a brush and fired at 980 °C. The frameworks were then veneered with two layers of dentine ceramic (VITA VM9 Base Dentine; VITA Zahnfabrik) that were heated to 910 °C, and 900 °C, respectively. Both the incisal veneering (VITA VM9 Enamel ENL; VITA Zahnfabrik) and the glazing processes were performed at 900 °C.
Concerning the ZZ frames, a 0.1/0.2-mm film of liner (IPS e.max Ceram ZirLiner; Ivoclar Vivadent) was fired at 960 °C. The structures were then coated with two layers of dentine and one layer of enamel of compatible veneering ceramic (IPS e.max Ceram; Ivoclar Vivadent) that were heated to 750 °C. The restorations were glazed at 725 °C.
Thus, monolithic zirconia (LM group) was left unveneered. These samples had a total thickness of 1 mm at the axial walls, and 2.5 mm at the occlusal surfaces. In the bi-layered groups, the thickness of the veneering coating was 0.5 mm at the axial walls, and 2 mm at the occlusal surfaces. Hence, all of the samples had identical final dimensions. During fabrication, the thickness of each structure and veneering material were verified by taking measures at different locations with a digital micrometer (Mitutoyo Co., Tokyo, Japan) with an accuracy of 0.01 mm. Finally, both retainers of every restoration were numbered to identify the study group and specimen .
2.2
Material characterization
Prior to the mechanical test, the microstructure was analysed on flat-parallel 1.8 mm-thick samples ( n = 10 per zirconia group) that were cut from pre-sintered CAD/CAM blocks in a metallographic micro-cutting machine (Micromet; Remet, Bologna, Italy) with a diamond disk under irrigation. The specimens were sintered and veneered according to the manufacturers’ specifications. The final pieces were embedded in epoxi resin and polished to 1 μ m in an automatic device (Phoenix Beta; Buehler) by using different diamond suspensions. Finally, samples were thermal-etched at 1300 °C for 30 min. The specimens were then examined under Field emission gun scanning electron microscope (FEG-SEM, Nova NanoSEM 230; FEI, Eindhoven, Holland) at 40,000× magnifications. The average grain size was determined by applying the line intersection method to the representative FEG-SEM images of each group (ASTM E112 -96(2004)e2 linear intercept count method) .
2.3
Luting procedure
The FDPs were randomly luted to the master dies with glass-ionomer cement (Ketac-Cem EasyMix; 3M-ESPE) in standard fashion . The same powder-liquid proportion and mixing time (30 s) were used in all cases. All of the FDPs were handled by one operator at room temperature (RT: 23.0 ± 1.0 °C) and relative humidity (50 ± 5%) . A special clamp was designed to maintain a constant seating pressure during cementation . The axial surfaces of the abutments placed in the clamp were varnished with a thin layer of cement before inserting each FDP. The clamp press was unscrewed until its base made contact with the occlusal surface of the introduced restoration . The upper screw that controlled the press was fitted with a dynamometric key (USAG 820/70; SWK Utensilerie SRL, Milan, Italy) that permitted the application of a compressive force of 10 N for 10 min (thus surpassing the total working time of the luting agent, which was 7 min). This uniform axial load was applied to ensure a correct cement distribution and to counteract the thyrotrophic behaviour of the luting material . Finally, the excess cement was removed with a plastic scaler to avoid scratching or gouging the abutments and/or restorations .
2.4
Thermo-mechanical cycling
After 48 h of water storage, all cemented samples were subjected to thermo-mechanical cycling. Mechanical cycles of axial compressive loads were exerted by a masticatory simulator (Chewing Simulator CS-4.2 economy line; SD Mechatronik GmbH, Feldkirchen-Westerham, Germany) through a customised cone-shaped stainless-steel bar finished in a rounded tip that described a vertical displacement of 2.5 mm at a speed of 60 mm/s. The simulator applied forces of 50 N at the central fossa of each pontic, equidistantly from the abutments .
Thermal cycles were accomplished by using a specific machine (Thermo-cycling TC-3; SD Mechatronik GmbH) that was adapted to the chewing simulator. Therefore, both the mechanical and thermal cycling were simultaneously performed maintaining the functional independence of both equipments. The thermo-cycling was made in distilled water, with a dwell time of 30 s and temperatures ranging from 5 °C to 55 °C (41 °F to 98 °F) .
Each sample was thermo-mechanical cycled for 18 h at rates of 43 thermal cycles and 6550 mechanical cycles per hour. Hence, each restoration underwent 774 thermal and 120,000 mechanical cycles. These data simulated approximately 6 months of oral service . Afterwards, all pieces were analysed under a stereomicroscope (Nikon SMZ-10; Nikon Corporation, Tokyo, Japan) at 40× magnifications to verify that no fracture had occurred on the veneering ceramic.
2.5
Three-point bending test
After thermo-mechanical fatigue simulation, all FDPs were subjected to a three-point bending test until fracture according to the ISO 6872:2008 standard by means of a universal testing machine (UTM, ME 405/10; SERVOSIS SA, Pinto, Madrid, Spain) operated with a 2000 kgf load cell at a cross-head speed of 1 mm/min at RT .
A special metallic platen (height: 7 mm, length: 6 mm, width: 6 mm) was adapted to the UTM for accommodating the bases of the specimens. Axial compressive static loads were exerted by sliding a customised cone-shaped stainless-steel bar (length: 12 mm) finished in a rounded tip (diameter: 1 mm). The machined load piston was adapted to the UTM and was perpendicularly applied at the central fossa of each pontic, equidistantly from the abutments , until the fracture of the veneering material (VF) and the complete fracture of the restoration (TF) occurred. The fracture initiation point (VF) was determined by a sharp decrease in the stress plot of the loading curve and was perceived as visible signs of chipping and loud cracking sounds. Therefore, in this study, the VF variable represented the ‘major chipping’ that is clinically considered as ‘failure’. The TF was defined by a drastic drop in the loading curve together with the visible breakage of the piece . VF and TF values were registered for each specimen using inbuilt software for the testing machine (PCD2K; SERVOSIS SA) that allowed tensile-deformation or force (N)-displacement (mm) curves to be automatically created. 3 The load values were recorded in kilograms-force (kgf) and were converted into Newtons (N) before data analysis .
2.6
Statistical analysis
Ten observations were made per zirconia group for microstructure analysis, resulting in a total of 40 measures in the experiment. Ten VF values and ten TF values were recorded for each group tested except for the monolithic LM group, in which only TF values were taken. This yielded a total of 40 VF and 50 TF values.
Means and standard deviations (SD) of average grain size, VF and TF values were calculated for each study group. The normality of the variables was confirmed by the Kolmogorov-Smirnov and the Shapiro-Wilk tests and the homogeneity of variances was verified according to the Levene’s test .
The one-way analysis of variance (ANOVA) was run to assess the contribution of the material and manufacturing technique to: (1) the average grain size of each zirconia group, and (2) to the fracture load values (VF and TF) registered after thermo-mechanical fatigue and three-point bending test. The Tukey’s honestly significant difference (HSD) test was used for multiple post-hoc comparisons when the overall ANOVA was significant .
Data were processed using the Statistical Package for the Social Sciences (software v.22) (SPSS/PC+, Inc.; Chicago, IL, USA) taking in advance the cut-off level for statistical significance at α = 0.05 .
2.7
Evaluation of the failure modes
The broken samples were gently ultrasonicated for 1 h and air dried. The fracture patterns were visually inspected and evaluated under a stereomicroscope (Nikon SMZ-10; Nikon Co.) at 15× magnifications . Failure types were classified as ductile (D: plastic deformation and tearing of the material) or brittle (B: no significant plastic deformation, showing flat surfaces that fit each other along the fracture line) . Percentages of ductile and brittle fractures were calculated for each experimental group. The fracture location was also recorded .
2
Materials and methods
2.1
Fabrication of the restorations
A hundred standardised stainless-steel master dies that simulated premolars prepared to be retainers of all-ceramic FDPs were fabricated. The abutments’ configuration was: 5 mm in height; convergence of 6°; chamfer of 120°, 1 mm in width and 8 mm in diameter; axial reduction of 1 mm; vestibular and lingual cuspids separated by a central groove of 1 mm in depth and rounded angles . The dies were randomly screwed in pairs onto fifty metallic bases customised as follows: 4.5 mm in height, 30 mm in length, 17 mm in width and two centred perforations separated by 7 mm.
The fifty sets of samples formed by the abutments fixed onto their respective bases were sandblasted with 100 μ m-alumina particles to eliminate the surface brightness and facilitate the scanning procedures and were randomly assigned to five groups ( n = 10 each) depending on the material and manufacturing technique to be used for constructing 3-unit posterior FDPs with an intermediate pontic: MC: Cast metal-ceramic (control); LZ: Lava Zirconia (3 M ESPE, Seefeld, Germany); LM: Lava Plus (3 M ESPE); YZ: VITA In-Ceram YZ (Vita Zahnfabrik, Bad Säckingen, Germany) and ZZ: IPS e.max ZirCAD (Ivoclar Vivadent, Schäan, Liechtenstein).
The LM group consisted of monolithic zirconia (ZrO 2 ) pieces, while the remaining zirconia groups, i.e., LZ, YZ, and ZZ were bi-layered restorations of yttrium-stabilised zirconium oxide frameworks veneered with glass ceramic. To date, the specific chemical identity and/or exact composition of the polycrystalline zirconia frames has frequently been withheld as a trade secret. Nonetheless, as a reference, the YZ cores include 91–94% of ZrO 2 , 4–6% of Y 2 O 3 , 2–4% of HFO 2 , and less than 0.1% of Al 2 O 3 , SiO 2 , and Na 2 O. The same occurs with the composition of the veneering porcelain. However, in all cases (MC, LZ, YZ, and ZZ), the coating material were feldspathic ceramic (or ‘glass ceramic’) and consisted almost entirely of an amorphous glass phase with crystalline constituents such as leucite crystallites. All FDPs were obtained according to the manufacturers’ instructions.
The restorations of the MC group (control) were fabricated using the traditional lost-wax casting technique. A base metal alloy of white Co-Cr for ceramics was selected (Ugirex C; Ugin Dentaire, Seyssinet-Paris, France). The alloy composition was: Co: 62%; Cr: 31%; Mo: 4%; and Si: 2.2%. The patterns of the structures were waxed-up onto the metallic abutments, which were varnished with three layers of die spacer beforehand to leave gap of 50 μ m for the luting material. The frameworks were modelled with a wall-thickness of 0.5 mm and round-shaped connectors of 9 mm 2 , and were invested. The MC structures were cast in an induction heated vacuum pressure machine (Jelrus Infinity L30; Whip Mix, Dortmund, Germany), at 1470 °C. The casts were then retrieved and cleaned using airborne-particle abrasion with alumina (Al 2 O 3 ) powders (125 μ m) for 10 s at a working distance of 5 mm and a pressure of 2 bars.
For the oxidation of the divested structures, two thin layers of opaque porcelain (Omega 900; VITA Zahnfabrik, Bad Säckingen, Germany) were applied to the metal surfaces and were fired to 900 °C under vacuum in a calibrated ceramic oven (Programat P500/G2; Ivoclar-Vivadent AG, Schäan, Liechtenstein). The metallic cores were then veneered with dental and incisal compatible glass ceramic (Omega 900; VITA Zahnfabrik) at 910 °C. Finally, the glazing procedure was carried out at 915 °C.
The LZ, LM, YZ, and ZZ samples were prepared by an experienced technician who was accustomed to work with the CAD/CAM systems tested. The fabrication of the Y-TZP structures started by scanning and digitizing each pair of abutments with a specific non-contact, optical scanner depending on the study group: Lava Scan (band projection/triangulation, precision in the range of 25 μ m; 3 M ESPE, Seefeld, Germany) for LZ and LM groups; and InEos X5 Scanner (5-axis technology with automatic positioning combined with auto focus, precision <12 μ m; Sirona Dental, Bensheim, Germany) for YZ and ZZ samples. A 3-D model was generated in each scanning cycle by using the next packages software for Windows: Lava System 3.01 CAD (3 M ESPE) for LZ and LM specimens and Sirona inLab 3D (Sirona Dental) for YZ and ZZ groups.
The following parameters were programmed with the abovementioned software. For the bi-layered groups (LZ, YZ, and ZZ): wall thickness of 0.5 mm in each plane. For the monolithic group (LM): total thickness of 1 mm at the axial walls and 2.5 mm at the occlusal surfaces. In all zirconia groups: an internal space of 50 μ m for the luting agent and rounded connectors of 9 mm 2 . An enlargement of 20% was envisioned in all zirconia specimens to offset the shrinkage derived from the final sintering procedure that permitted zirconia pieces to achieve an increased density at their final size .
Pre-sintered CAD/CAM blocks of Y-TZP that were compatible with each system were selected to mechanise the samples in the respective milling units, i.e., Lava Form (3 M ESPE) for the LZ group (obtaining milled structures) and LM group (obtaining milled FDPs with their final shape); and Sirona inLab (Sirona Dental) for the YZ and ZZ groups (obtaining milled structures). The sintering procedures of the enlarged pieces lasted for 8 h and were accomplished in specific ovens at high temperatures: Lava Therm (3 M ESPE) at 1500 °C for the LZ and LM groups; and VITA Zyrcomat (VITA Zahnfabrik) at 1530 °C for the YZ and ZZ groups.
The zirconia structures of the bi-layered groups were veneered in a specific oven (Programat P500/G2 M; Ivoclar Vivadent) with compatible hand-layered feldsphatic ceramic.
In the case of the LZ frameworks, a 0.1/0.2-mm film of liner (Zirconia Overlay Porcelain for Lava Frame; 3 M ESPE) was applied and submitted to a firing cycle at 820 °C. The structures were then covered with the corresponding ceramic (Lava Ceram; 3 M ESPE). 3 The first layer of dentine was submitted to 810 °C, while both the second layer of dentine and the incisal coating were fired at 800 °C. The glazing procedure was made at 790 °C.
With respect to the YZ structures a 0.1/0.2-mm layer of liner (VITA VM9 Effect Bonder; VITA Zahnfabrik) was spread with a brush and fired at 980 °C. The frameworks were then veneered with two layers of dentine ceramic (VITA VM9 Base Dentine; VITA Zahnfabrik) that were heated to 910 °C, and 900 °C, respectively. Both the incisal veneering (VITA VM9 Enamel ENL; VITA Zahnfabrik) and the glazing processes were performed at 900 °C.
Concerning the ZZ frames, a 0.1/0.2-mm film of liner (IPS e.max Ceram ZirLiner; Ivoclar Vivadent) was fired at 960 °C. The structures were then coated with two layers of dentine and one layer of enamel of compatible veneering ceramic (IPS e.max Ceram; Ivoclar Vivadent) that were heated to 750 °C. The restorations were glazed at 725 °C.
Thus, monolithic zirconia (LM group) was left unveneered. These samples had a total thickness of 1 mm at the axial walls, and 2.5 mm at the occlusal surfaces. In the bi-layered groups, the thickness of the veneering coating was 0.5 mm at the axial walls, and 2 mm at the occlusal surfaces. Hence, all of the samples had identical final dimensions. During fabrication, the thickness of each structure and veneering material were verified by taking measures at different locations with a digital micrometer (Mitutoyo Co., Tokyo, Japan) with an accuracy of 0.01 mm. Finally, both retainers of every restoration were numbered to identify the study group and specimen .
2.2
Material characterization
Prior to the mechanical test, the microstructure was analysed on flat-parallel 1.8 mm-thick samples ( n = 10 per zirconia group) that were cut from pre-sintered CAD/CAM blocks in a metallographic micro-cutting machine (Micromet; Remet, Bologna, Italy) with a diamond disk under irrigation. The specimens were sintered and veneered according to the manufacturers’ specifications. The final pieces were embedded in epoxi resin and polished to 1 μ m in an automatic device (Phoenix Beta; Buehler) by using different diamond suspensions. Finally, samples were thermal-etched at 1300 °C for 30 min. The specimens were then examined under Field emission gun scanning electron microscope (FEG-SEM, Nova NanoSEM 230; FEI, Eindhoven, Holland) at 40,000× magnifications. The average grain size was determined by applying the line intersection method to the representative FEG-SEM images of each group (ASTM E112 -96(2004)e2 linear intercept count method) .
2.3
Luting procedure
The FDPs were randomly luted to the master dies with glass-ionomer cement (Ketac-Cem EasyMix; 3M-ESPE) in standard fashion . The same powder-liquid proportion and mixing time (30 s) were used in all cases. All of the FDPs were handled by one operator at room temperature (RT: 23.0 ± 1.0 °C) and relative humidity (50 ± 5%) . A special clamp was designed to maintain a constant seating pressure during cementation . The axial surfaces of the abutments placed in the clamp were varnished with a thin layer of cement before inserting each FDP. The clamp press was unscrewed until its base made contact with the occlusal surface of the introduced restoration . The upper screw that controlled the press was fitted with a dynamometric key (USAG 820/70; SWK Utensilerie SRL, Milan, Italy) that permitted the application of a compressive force of 10 N for 10 min (thus surpassing the total working time of the luting agent, which was 7 min). This uniform axial load was applied to ensure a correct cement distribution and to counteract the thyrotrophic behaviour of the luting material . Finally, the excess cement was removed with a plastic scaler to avoid scratching or gouging the abutments and/or restorations .
2.4
Thermo-mechanical cycling
After 48 h of water storage, all cemented samples were subjected to thermo-mechanical cycling. Mechanical cycles of axial compressive loads were exerted by a masticatory simulator (Chewing Simulator CS-4.2 economy line; SD Mechatronik GmbH, Feldkirchen-Westerham, Germany) through a customised cone-shaped stainless-steel bar finished in a rounded tip that described a vertical displacement of 2.5 mm at a speed of 60 mm/s. The simulator applied forces of 50 N at the central fossa of each pontic, equidistantly from the abutments .
Thermal cycles were accomplished by using a specific machine (Thermo-cycling TC-3; SD Mechatronik GmbH) that was adapted to the chewing simulator. Therefore, both the mechanical and thermal cycling were simultaneously performed maintaining the functional independence of both equipments. The thermo-cycling was made in distilled water, with a dwell time of 30 s and temperatures ranging from 5 °C to 55 °C (41 °F to 98 °F) .
Each sample was thermo-mechanical cycled for 18 h at rates of 43 thermal cycles and 6550 mechanical cycles per hour. Hence, each restoration underwent 774 thermal and 120,000 mechanical cycles. These data simulated approximately 6 months of oral service . Afterwards, all pieces were analysed under a stereomicroscope (Nikon SMZ-10; Nikon Corporation, Tokyo, Japan) at 40× magnifications to verify that no fracture had occurred on the veneering ceramic.
2.5
Three-point bending test
After thermo-mechanical fatigue simulation, all FDPs were subjected to a three-point bending test until fracture according to the ISO 6872:2008 standard by means of a universal testing machine (UTM, ME 405/10; SERVOSIS SA, Pinto, Madrid, Spain) operated with a 2000 kgf load cell at a cross-head speed of 1 mm/min at RT .
A special metallic platen (height: 7 mm, length: 6 mm, width: 6 mm) was adapted to the UTM for accommodating the bases of the specimens. Axial compressive static loads were exerted by sliding a customised cone-shaped stainless-steel bar (length: 12 mm) finished in a rounded tip (diameter: 1 mm). The machined load piston was adapted to the UTM and was perpendicularly applied at the central fossa of each pontic, equidistantly from the abutments , until the fracture of the veneering material (VF) and the complete fracture of the restoration (TF) occurred. The fracture initiation point (VF) was determined by a sharp decrease in the stress plot of the loading curve and was perceived as visible signs of chipping and loud cracking sounds. Therefore, in this study, the VF variable represented the ‘major chipping’ that is clinically considered as ‘failure’. The TF was defined by a drastic drop in the loading curve together with the visible breakage of the piece . VF and TF values were registered for each specimen using inbuilt software for the testing machine (PCD2K; SERVOSIS SA) that allowed tensile-deformation or force (N)-displacement (mm) curves to be automatically created. 3 The load values were recorded in kilograms-force (kgf) and were converted into Newtons (N) before data analysis .
2.6
Statistical analysis
Ten observations were made per zirconia group for microstructure analysis, resulting in a total of 40 measures in the experiment. Ten VF values and ten TF values were recorded for each group tested except for the monolithic LM group, in which only TF values were taken. This yielded a total of 40 VF and 50 TF values.
Means and standard deviations (SD) of average grain size, VF and TF values were calculated for each study group. The normality of the variables was confirmed by the Kolmogorov-Smirnov and the Shapiro-Wilk tests and the homogeneity of variances was verified according to the Levene’s test .
The one-way analysis of variance (ANOVA) was run to assess the contribution of the material and manufacturing technique to: (1) the average grain size of each zirconia group, and (2) to the fracture load values (VF and TF) registered after thermo-mechanical fatigue and three-point bending test. The Tukey’s honestly significant difference (HSD) test was used for multiple post-hoc comparisons when the overall ANOVA was significant .
Data were processed using the Statistical Package for the Social Sciences (software v.22) (SPSS/PC+, Inc.; Chicago, IL, USA) taking in advance the cut-off level for statistical significance at α = 0.05 .
2.7
Evaluation of the failure modes
The broken samples were gently ultrasonicated for 1 h and air dried. The fracture patterns were visually inspected and evaluated under a stereomicroscope (Nikon SMZ-10; Nikon Co.) at 15× magnifications . Failure types were classified as ductile (D: plastic deformation and tearing of the material) or brittle (B: no significant plastic deformation, showing flat surfaces that fit each other along the fracture line) . Percentages of ductile and brittle fractures were calculated for each experimental group. The fracture location was also recorded .
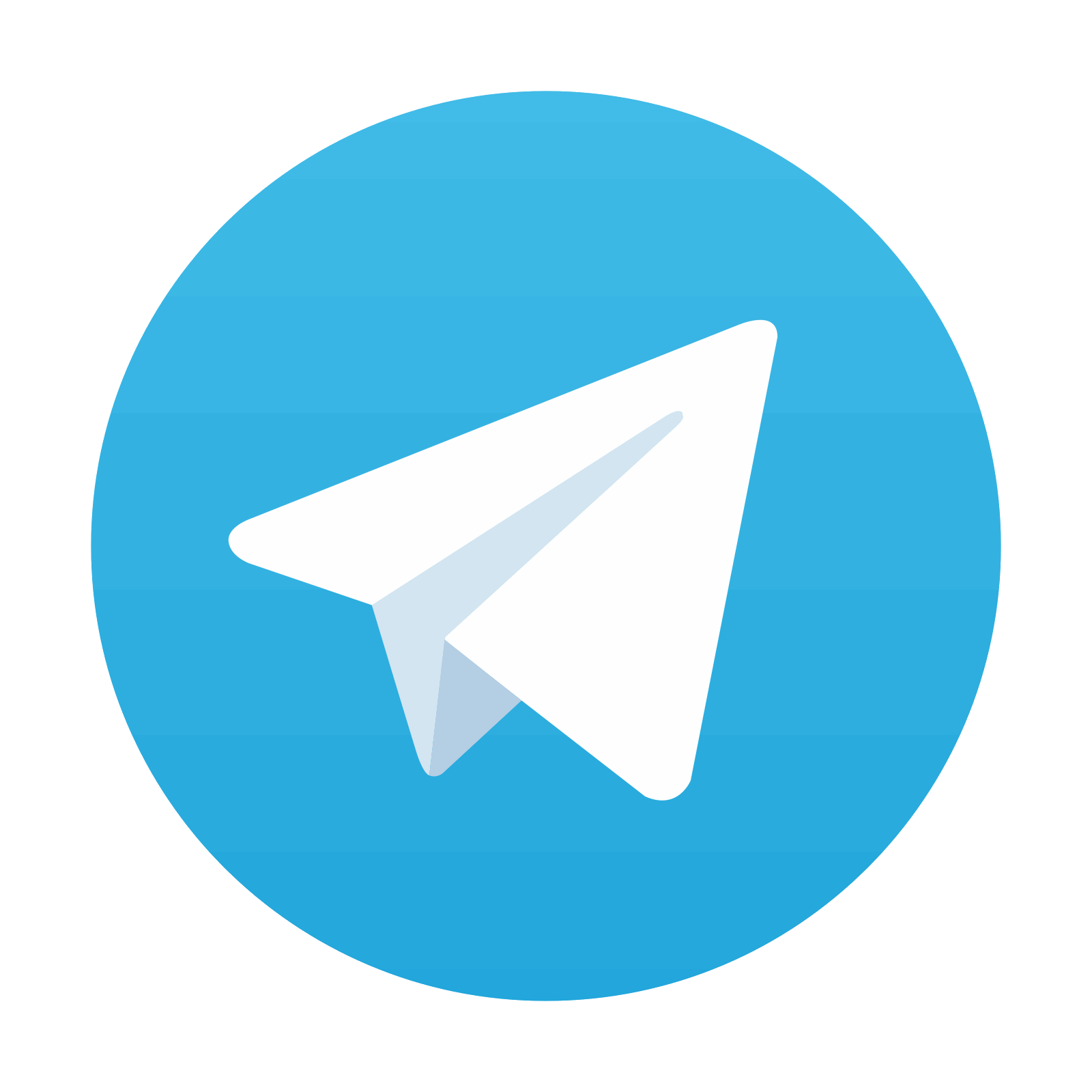
Stay updated, free dental videos. Join our Telegram channel

VIDEdental - Online dental courses
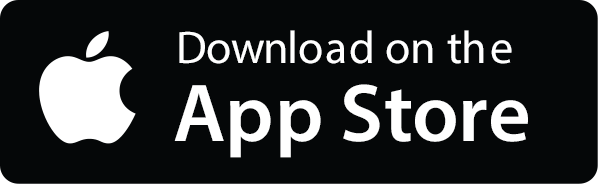
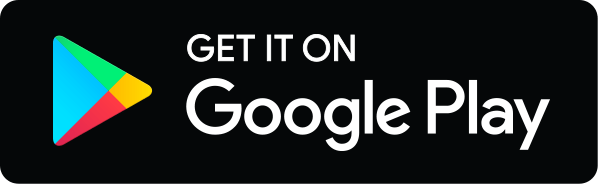