Fig. 9.1.
Bone multi-cellular units (BMUs) consist of osteclasts and osteoblasts which are combining their activities to a cutting cone that “eats” bone ahead of the osteon and depositons bone inside the newly formed cavity. The zone between cutting and deposition is called reversal zone. The tunnelling takes place at approx. 40 μm a day. On histological sections BMUs may be seen in from the side (as in the lower picture) or they may be cut as shown in the upper three sections. Newly deposited bone can be labelled with several stains, usually with tetracycline. This substance deposits in high concentration in newly mineralized bone, thus telling us which osteons or which areas of woven bone are new and refilling at the time of labelling (compare Figs. 23.32a, 23.33). (Drawing from Martin et al. 1998)
Regardless of the type of early bone healing at the implant site, osteonal bone will have formed in due course and will increasingly mature and mineralize. The life cycle of osteons covers the following stages:
-
Activation phase. Precursor cells develop into differentiated cells (mainly osteoclasts) within around 3 days.
-
Resorption phase. Newly formed osteoclasts give rise to bone resorption at a speed of 40 μm/day. A “cutting cone” of cells (≈300×200 μm in length/width) is advancing through the bone structure, leaving behind a non-mineralized tunnel filled with fluid. The bone is becoming increasingly porous in the process. This increase in porosity is associated with higher elasticity and low mechanical fracture resistance.
-
Reversal phase. Within several days, the osteoclasts give way to osteoblasts as the prime source of activity, which gives rise to a cylindrical space emerging between the tip of the “cutting cone” and the “tail”. The length of this space depends on the delay from bone resorption to bone formation. In finished secondary osteons, the cement line coincides with the location forming the bone surface during this reversal phase. The duration of this reversal phase in humans is around 30 days.
-
Phase of new bone formation. Osteoblasts first appear in the periphery of the tunnel, where they start forming concentric matrix lamellae. This involves a diameter loss of about 1.2 μm/day. A central (haverian) canal needs to remain, since the osteoblasts need to be nourished. In humans, these haverian canals reach a diameter of around 40–50 μm by the end of the filling phase. Additional closure of the canals is not planned. The duration of this phase of bone formation is around 3 months.
-
Mineralization phase. After non-mineralized matrix (“osteoid”) has been deposited, a gradual process of mineralization is started. There is a delay of around 10 days between the end of matrix formation and the beginning of mineralization. The “primary mineralization” occurring within the first few days after the process has been initiated accounts for around 60% of all mineralization. The secondary mineralization phase, which gives the bone its definitive hardness, will then take another 6–12 months, depending on the local functional requirements.
-
Dormant phase. After the tunnelling and filling processes have been completed, a dormant phase ensues in which the osteoblasts will develop either into osteocytes or into the cells lining the haverian canal. In this phase, the cells take up their mechanical, metabolic and homeostatic function.
This cascade of processes will always develop in a uniform manner if nothing interferes with them. The term BMU (bone morphological unit) as used hereinafter is commonly used to refer to this “cutting cone”. BMU covers the whole process outlined above in its cellular and spatial entirety – i.e. the activity of osteoclasts as well as the deposition of matrix and the process of mineralization.
Once we have understood this cascade of processes, it becomes very hard to explain the “miraculous” PRP (platelet-rich plasma) treatment, which is supposed to yield benefits in a matter of days. Most of the properties commonly ascribed to PRP therapy today cannot possibly exist as stated. The marketing claims made for PRP – which have been highly effective and are supported by many dental schools – is unfounded from a scientific viewpoint. In fact, it is a telling example of how inadequately trained general practitioners and, indeed, numerous specialized periodontologists and implantologists, are duped by industry into acquiring “new clothes for the emperor”. If for no other reason, PRP cannot become effective due to platelet aggregation. Furthermore, as later chapters of this book will show, there can be no greater incentive for bone formation and maintenance than continuous function. PRP certainly is not a substitute for this either.
What does make sense, however, is to fill extraction sockets with bone-grafting materials such as Bio Oss or tricalcium phosphate – indeed, even inexpensive calcium sulphate or (tri–)calcium phosphate from the pharmacy may do. After all, these extraneous materials will prevent reorientation (i.e. straightening) of the osteons surrounding the extraction socket like a belt by their sheer physical barrier function in the initial phase of bone healing, which would otherwise cause the alveolar socket walls to collapse. It is irrelevant whether the filling material is going to be resorbed down the road, as the osteonal system will be back in a state of equilibrium in the later stages of healing (particularly in adults), so that the morphological changes of the cortical bone segments are no longer as pronounced as previously.
A more important issue for the successful outcome of implant placements concerns the direction in which the BMUs are moving through the bone.
BMUs can start and end at any cortical bone surface (periosteum, endosteum, haverian canals) that is not covered by cartilage or excluded from remodellation for other reasons (e.g. osteoid). They usually move through the bone in longitudinal direction, without being affected by any primary or secondary osteons that may exist by that time. BMUs are capable of developing into separate prongs in completely different directions. It is assumed that the direction ultimately depends on the degree of mechanical stresses and on the bone structure. Note that the very formation of BMUs will alter the stress distribution inside the local bone as stable mineralized areas are being dissolved. Therefore, no clear line can be drawn between the cause and effect of BMU activity with regard to its direction and the distance covered. BMU can catch up any substances inside the bone during their travel. They will then unload them under the periosteum, which serves as a exchange plane. Some substances, like metal oxides, may travel with the BMUs out of the bone but they will not be able to pass the barrier of the periosteum into the blood. Instead, they will remain like a tattoo on the inside of the periosteum, which could then alter the permeability of the periosteum for other substances. Keep this in mind, when reading Chapter 9.6.
Whether the filling of tunnels is accomplished by one generation or several generations of osteoblasts is uncertain. At any rate, the filling phase does not proceed in a uniform manner but includes delays during which the osteoblasts may either remain at rest or may separate.
Osteons are supply canals with a very large surface. In non-vascularized bone areas, they take care of nutrient exchange by way of diffusion. Elastic bone structures promote the intraosteonal conveyance of nutrients by movement-related deformation. This beneficial effect is absent in more rigid bone structures; therefore, the osteons in long tubular bones have a larger lumen than the osteons in the ribs to compensate for the lack of deformation.
With respect to BOI implants (and implants in general), this means that definitive “stable” incorporation of implants into the functional processes inside the oral cavity can be expected after 1 or 2 years at the earliest. After that time, the complication rate will decrease, as both the bone trajectories and the masticatory function will have approached a stable upper limit. A true steady state is not attainable, however, for two reasons. Firstly, the residual functional stimulus is too small (note also that long tubular bones are never completely straight) for this transformation to be overcome. Secondly, any trajectorial adaptation will give rise to muscular adjustments and vice versa, which will keep any systems of this type “oscillating” on a suboptimal level. Trajectorial adaptation is always a consequence of past functional stresses, and any functional stresses occurring at present will be reflected in bone morphology with a long delay involved.
9.1.2 Why Does BMU Activation Occur?
BMUs can be activated through various stimuli:
-
BMUs may be activated to repair any cracks and microcracks inside the bone that have been caused by excessive loads (Mori and Burr 1993; Burr et al. 1985). At first, the repair work will be confined to the site of initial injury. However, since the repair mechanism involves a greater degree of porosity, which will alter the tension of the bone as a whole, situations may arise in which the porous zone propagates over a large area. Bone damage and trauma will initiate additional repair mechanisms beyond the physiological routine. The repaired bone shows a smaller density of secondary osteons at a higher number of BMU activations. In other words, the bone is literally “rejuvenated” in the process of remodelling – a process that may spread to extended bone areas (Atkinson et al. 1977).
-
Disuse will give rise to a higher degree of porosity as well. After all, BMUs are formed at a certain frequency in an age-dependent manner. In the event of disuse, the tunnels are not refilled quickly, and bone porosity will increase, which will eventually cause the overall degree of mineralization to decrease. Unlike in bone trauma, however, where the remodelling remains largely confined to the crack area, disuse-related porosity invariably causes the bone structure to remodel in a uniform manner.
-
Disregarding trauma and disuse as causative factors, BMU activation is normally an age-dependent phenomenon controlled by hormones. Activation frequencies are given in BMU activated per mm2 and year. They are highest in childhood (up to 60 BMU/mm2/year) and lowest in old age (down to 1–2 BMU/mm2/year). BMU activation frequencies in rib bones have been shown to drop to their first valley at age 35, followed by a slight recovery until they drop once again later in life. The curve resembles a damped oscillation pattern (Fig. 9.4).
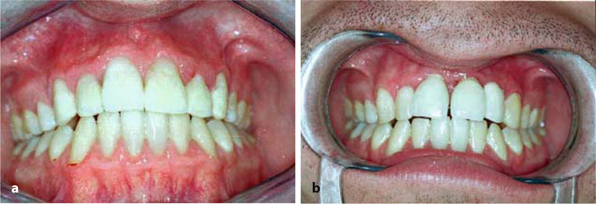
Fig. 9.2.
a Insertion of three crestal implants replacing teeth 11, 21 and 22, followed by immediate loading with three single crowns, b Four months later, the osseointegrated implant replacing tooth 11 has started to migrate in a lateral vestibular direction. The vestibular cortical bone was remodelled (Atkinson et al. 1977), with the result that the implant-supported crown has undergone reorientation due to the equilibrium of forces between the tongue and upper lip. We have observed the same development of bone after immediate loading of single-tooth BOI implants. Immediately loaded single-tooth restorations using BOI implants might require overnight splinting during the remodelling period of the vestibular cortical wall, which is expected between the 3rd and 6th months postoperatively
Another lesson that Fig. 9.4 holds for our work is that any implant treatment of patients past the age of 35 will be performed on a relatively inactive, resting osteonal system characterized by a very low degree of remodelling per se. The remodelling triggered by the implant procedure will then first induce remodelling of the surgical site, but the processes will extend throughout the entire jaw segments. The main factors determining the direction of this remodelling are force-related effects by macrotrajectories and in the interface area, but also hormonal influences as far as the direction and extent of bone remodelling are concerned. Implant treatments performed on patients under 25 years, by contrast, are not likely to trigger a lot of additional remodelling, so that the effects on macrotrajectories will be smaller as well (see also Marx and Garg 1998). Recently preoperated sites, however, show a higher BMU activation rate due to ongoing remodelling. The placement of BOI implants in these areas is still possible, but immediate loading may not be advisable.
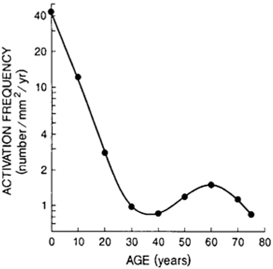
Fig. 9.3.
Remodelling in response to dynamic loading (Rubin 1984). Four loading cycles per day (cpd) were sufficient to keep the bone mass constant in this experiment
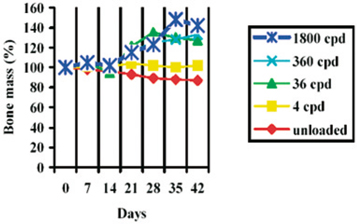
Fig. 9.4.
BMU activation plotted against age (Burr et al. 1998)
Histological studies are an important element of implantological research. Another notable implication of Fig. 9.4 is that the implantological data obtained in animal experiments cannot be readily translated to humans, since they are usually performed on young adult animals (i.e. shortly after growth has been completed). Those young animals are thus in another “phase of bone life”, corresponding to the leftmost segment of our graph, while our dental implant patients tend to be past 40, thus showing a small degree of spontaneous BMU activity; their osteonal systems are more or less at rest.
The following conclusions can be drawn:
-
Strengthening of the masticatory force will cause any cracks and microcracks in the bone around load-transmitting disk areas to increase and give rise to a greater degree of porosity associated with increased mobility of the implant. This does not necessarily mean, however, that direct contact at the implant-bone interface is lost. Porosities can accumulate to a point where perfusion inside the repair area is so strong that the degree required of mineralization can not be maintained: we call this state “overload osteolysis”. It is a reversible situation, as long as no infections affects these areas, modifies the properties of the tissue and induces granulation.
-
Weakening of the masticatory force may give rise to generalized remodelling of the jawbone, which will also be accompanied by a decrease in bone mineralization. Moreover, any unloaded implants offering no functional stimulation of the surrounding bone structure will weaken the bone rather than strengthen it. Thereby, any force-transmitting trajectories are likely to circumvent these implants in their development (also see the discussion of 0–1 properties at the end of this chapter).
-
Any implants that do not participate in mastication may not be able to stimulate the bone at the interface in an adequate manner. Unloaded implants are thus more likely to be lost, which may take years. In that case, the demineralizing effect of bacteria in the collar area gains the upper hand and will eventually give rise to subacute chronic osteomyelitis associated with a massive decrease in mineralization.
9.1.3 Interactions Between Joints and Bone Changes
Hyaline cartilage can be neither mineralized nor processed by osteoblasts; they undergo no modelling or remodelling whatsoever. By contrast, remodelling does take place in fibrous cartilage, such as the cartilage covering the TMJs.
This difference appears logical if we consider the following facts:
-
Unlike any other joint in the human body, the TMJs are two joints on both ends of the same bone articulating with the same opposing bone (= the articular fossae of both temporal bones for the purpose of this discussion).
-
At the same time, both jaws are continuously subjected to morphological changes by the dentition – i.e. the formation, growth, eruption, use and loss of teeth.
-
These changes are so extensive that they would disengage the high-precision fit of the hyaline joint. Only fibrous cartilage is flexible enough to adapt accordingly. This adaptation also explains why unilateral chewing patterns give rise to radiographically manifest alterations of the TMJs. Significant flattening of the joints will, in its turn, increase the importance of muscular guidance. The ramifications of this short outline are discussed in Chapter 10.
-
Being arranged in a slanted position to the sagittal plane, the TMJs are designed neither for purely lateral nor for anterior rotational movements. Rather, this type of joint excels in lateroanterior dislocation, resulting in a grinding movement that is optimized for chewing. This automatically involves a long articular pathway on the balancing side, whereas the condyle on the working side performs what is better described as a rotational movement. These functional accomplishments are outside the scope of hyaline joints.
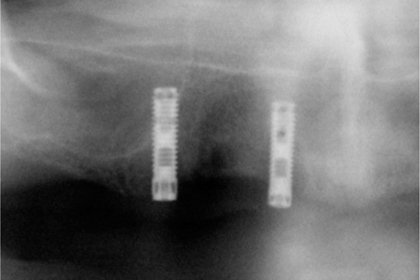
Fig. 9.5.
Unloaded screw implants (featuring no surface enlargement) 8 years after insertion. Those implants could be easily removed by just “picking them up”. They were characterized by chronic inflammation after having been repeatedly covered by mucosa. The macrotrajectories avoid these implants according to the 0–1 rule, as no bone-preserving stimulus is present at the enossal implant surface
9.1.4 Hormonal and Mechanical Influences During Growth and Aging
The age of bone samples of known topographical origin (e.g. from tibial bone) can be accurately determined based on the number of secondary osteons in any given cross-section. On this basis, the age of a patient can be pinpointed to ±5–7 years (Hattner and Frost 1963). Thus the number of secondary osteons gradually rises throughout life; over the years, they become increasingly overlapped and condensed (Kerley 1965). The long tubular bones have a tendency to “straighten out” in accordance with their function over time. 1 Anyone who has had an opportunity to monitor anatomical developments in the first years of life may have noted that the thighs will straighten out all of a sudden once the child is beginning to walk. In this situation, the strong flexion is not longer acceptable from a mechanical viewpoint, but this problem is solved amazingly fast in accordance with Frost’s flexural neutralization theory (FNT). The crucial mechanism is periosteal modelling along the concave bone segments.
In phases of substantial growth, the rate of bone remodelling is greater in children than in adults, which is naturally due to the hormonal situation. Due to the fast speed of growth in adolescents, the degree of mineralization may sometimes lag behind. The formation of plexiform bone as a temporary corsage is one of the precautions that the live bone may take against fracture as the stresses are continuously changing both in degree and in direction. Because the activity is usually decreased in adults as well, the frequency of BMU activation drops quickly. Apparently, this results in a shortage of activation which is then counteracted by a new rise in frequency.
9.1.5 Disuse Atrophy, Blood Supply and Periodontal Problems
Contrary to a widespread misconception, jawbones show resorption not because they are subjected to (denture) pressure, but because they suffer from undernourishment combined with a lack of drainage. 2 Pressure, as a rule, creates bone.
The observation that the vertical bone volume (particularly in the posterior segment of the mandible) rapidly decreases after insertion of a denture (Blatterfein and Payne 1985) has prompted explanations to the effect that the bone is “pushed away”. This notion of a denture-related pressure atrophy continues to be taught at dental schools and is firmly planted in dentists’ heads, despite the well-known fact that there is no relationship between the surface area of the denture base and the extent of atrophy, although such a relationship would be logical if the pressure per surface unit were a relevant factor (Suenaga et al. 1997).
Consequently, the reduction in bone volume is not due to the chewing pressure but to a combination of individual factors such as lack of intrabony blood supply in the absence of a drainage pathway from the crestal mucosa, which affects periosteal regeneration. Subsequently, the mandibular artery will be more susceptible than most other arteries to sclerosis and partial closure (Bradley 1975). This is particularly true after the teeth have been extracted. This artery is around 15 years ahead of the major carotid artery in terms of its degree of sclerosis. Therefore, the central blood supply to the mandible will be significantly reduced particularly in situations of advanced resorption (Mercier and Vinet 1983). Following tooth loss, the bone-stimulating function along the dental roots is gone, so that the alveolar jaw areas will simply succumb to disuse atrophy. The reason why the blood supply to the mandible is greatly reduced is that no more drainage pathways are present following tooth loss. While teeth are present, the bloodstream to the mandible is distributed to the dental roots and the periodontal ligament – where great amounts of blood are needed on a continuous basis to maintain the local immune system and nourish the tissues – and is drained through the subperiosteal tissue. After the last tooth has been extracted, the bone will close in a definitive manner, and the interior of the mandible becomes congested. The last remaining drainage pathway through the mental artery is overly narrow. Even while teeth are still present, the increase in mineralization in the mandible will significantly raise the resistance to intravasal pressure of the mandibular artery in the 4th decade of life, so that the direction of the blood supply may conceivably be reversed, thus going from the periphery to the centre, in some people. In heavily resorbed mandibles (i.e. in the remaining basal segments) it is known anyway that the bulk of the blood supply is guided from the peripheral zone through the periosteum. In other words, a number of points can be made to support the notion that the pattern of blood supply changes in the wake of bone loss along the alveolar crest. The main purpose of the extensive intrabony blood supply, which is without parallel in the human skeleton, is to nourish the teeth and to fight any infections associated with their presence. Otherwise, the mandibular artery would have to communicate with the “mandibular vein” after the terminal branches and capillaries contained in the teeth and periodontium have been lost.
Against the background outlined above, one of the essential problems with crestal implants in resorbed ridges appears to be that the interior blood supply needed to offer immune defence and enossal force transmission has been almost or completely drained by the time of implant placement. BOI implants, by contrast, mainly require the periosteal blood supply and drainage to be integrated and for force transmission to be supported. This blood supply will be available equally well at any age, even in situations of advanced ridge resorption. More importantly, it can be regulated much better and faster than the intrabony blood supply of the maxilla and mandible.
Any removable restorations inserted in the posterior segment will influence jaw movements by significantly reducing the vertical range of masticatory movements and reducing the lateral excursions in a matter of only around 2 years (Tallgren et al. 1989). These functional alterations will give rise to the qualitative changes in jaw morphology commonly observed in patients wearing dentures. Similar results are seen when the occlusal table on fixed restorations is small.
Conversely, the same functional alterations can give rise to a gain in bone volume after BOI treatment. The reader is referred to Chapter 9.3 for an exhaustive discussion of this mechanism.
Evidence for the existence of a relationship between drainage conditions and bone volume has been provided by Yaffe et al. (1994), who unilaterally mobilized mucoperiosteal flaps in the mandibles of rats, which were then immediately repositioned without suturing after only 30 s. The jaws were histologically followed up by comparing both sides after 3, 7, 10, 21 and 120 days. Massive resorption of the alveolar and endosteal bone was present after only 10 days. The impressive pictures in that report clearly show how much internal damage can be caused by external flap formation if no draining osteotomy is left after closing the flap. Bilateral (i.e. lingual and vestibular) flaps resulted in a greater degree of resorption than unilateral flaps, as the bloodstream is cut off altogether. After 120 days, the alveolar ridges were largely restored. It follows that this atrophy of internal bone structures is due to insufficient drainage, which in turn will cause the incoming blood supply to be reduced as well, thereby causing undernutrition. The open slots following the insertion of BOI implants are presumably capable of preventing this stasis.
9.1.6 Aspects of Periodontal Disease
During the development of periodontitis, the overpressure generated by the external periodontal tissue appears to obstruct drainage from the interior of the bone, which eventually leads to a deleterious state of stasis. Wang et al. (2003) showed that the average interstitial pressure will be elevated in situations of venous stasis and thus may influence at least the formation of periosteal bone.
In addition to directly influencing bacterial cultures, dental ultrasonic therapy apparently has a mechanical effect on this stasis and helps to restore regular flow conditions particularly in those regions that are not subject to the rhythmical bloodstream – i.e. in the osteonal structures where the internal flow of nutrients and waste products are conveyed by capillary action and mechanical deformation of the bone.. The reason why peri-implantitis therapy often fails may be that the rough surfaces are re-populated by microorganisms already during the healing phase that will immediately enhance the blood supply and cellular granulation, thereby impeding drainage. Compression screws featuring wide windings might therefore be less susceptible to peri-implantitis than solid screw implants, as the flanks of the windings will direct the intrabony bloodstream away from the implant. Moreover, osseointegration is synonymous with vertical corticalization in crestal implant designs. However, since cortical bone structures decelerate the flow of blood and nutrients, periosteal nourishment and periosteal drainage are important, but they are automatically disrupted after surgical treatment of periodontitis with flaps. Around implants, the transport of nutrients is greatly impeded by the implant itself, the blockage depending on their diameter and length. Short implants featuring surfaces that allow internal perfusion (e.g. Osseopore implants) have been successfully employed both in dental implantology and in orthopaedic surgery. These designs overcome the problem of nutrient conveyance by having a permeable surface. The longer these implants, the less likely they will survive, since the perfusion effect is reduced with increasing length, and mineralization in the interface area is reduced as well as bone areas of different macrotrajectorial orientation are crossed by the implant.
As soon as plaque-induced gingivitis results in a swelling of the gums, there is a risk that the flow of blood from the periodontal perfusion space is halted or at least impeded. If this happens, and if the perfusion of the bone is compromised as a result, gingivitis will turn periodontitis, accompanied by a bone reaction (atrophy) caused by reduced perfusion. At the same time, the local pH changes, and immunological competence is decreased.
For the reasons stated above, we regard it as an open question whether bacteria are really causally involved in the progression of periodontitis in the way we are taught to think. Ximenez et al. (2000) showed that the bacterial composition observed in periodontal pockets is also present in the supragingival plaque of patients with healthy periodontium. After all, it would be conceivable that those opportunistic microorganisms may be attracted by the degradation products of the increasingly undernourished periodontal pocket and may advance deeper for different reasons at a later time. In other words, the stasis that will eventually give rise to bone resorption may be caused by a gingivitis-related pressure reversal in the peripheral periodontal vessels. By way of analogy, no observer would assume that the vultures sitting on a dead gnu in the African savannah have actually killed the gnu. Current periodontological thinking does, however, assume that the bone is actually attacked or by the bacteria. Now, while there is no doubt that bacteria will facilitate the progression of periodontal disease, there are numerous indications arguing against their causal role in bone resorption.
It is well known that the anchorage of functional teeth in their bony bed is increasingly lost as periodontal disease progresses. As a consequence, microcirculation is reduced. Loginova and Krechina (1998) showed that microcirculation following the onset of masticatory function (as assessed with chewing gum) increased by 70% in the absence of periodontal disease. In moderate disease, the increase was 19–31%. In cases of severe deep periodontitis, however, the situation was reversed, i.e. circulation would even decrease by 6% (Loginova and Krechina 1998). The authors attributed this finding to changes in vascular micro-architecture involving, among other things, a reversed pressure relationship in the periodontal vessels. In this situation, the crestal segments of the periodontium are supplied from the periosteum and mucosa, while the basal segments continue to be supplied from the enossal space. Under normal pressure conditions, the enossal blood supply would drain to the periosteum and mucosa, but in the specific situation of acute infection, drainage is obstructed by the internal pressure increase in the crestal periodontium. Therefore, periodontitis is essentially a disease in which the bone is depleted of nutrients as the blood flow is undermined by blocking the drainage. The volume of the undernourished bone structures is reduced and it will be replaced by soft-tissue structures. These tissues are susceptible to inflammation caused by the numerous degradation products of the bone in general (enzymes, etc.) and the osteolysis-related high ion levels in particular. The newly formed periodontal tissue structures at the depleted alveolar crest are, in their turn, not particularly well suited to resorb these massive ion concentrations and bone degradation products. In order to ensure drainage of these products, the blood circulation through the crestal periodontium needs to be increased, which becomes clinically manifest as a heightened bleeding tendency.
Some indications to this effect are known to all dentists. Whenever teeth surrounded by large granulomatous regions are extracted, the granulomatous tissue will bleed heavily. The bleeding will stop quickly once the granuloma tissue has been removed. Thus the bleeding originates not in the bone but in the soft tissue. The bone frequently shows large cribriform openings in the contact area with the granuloma without bleeding from these openings when this tissue is removed. The same effect can be observed at childbirth where uterine haemorrhage is stopped quickly after the placenta has been dislodged; if the separation, however, fails or is incomplete, the haemorrhage may persist to the point of becoming life-threatening. Bleeding from the placenta itself is negligible because the lumen of the bloodstream from the newborn organism is too small, which is similar to the blood supply situation in the jawbone; the pressure is thus too low and, more importantly, cannot be regulated upward. In placenta, separate bloodstreams are exchanged at the interface. Jaw granulomas, by comparison, basically stem from one single bloodstream, but the pressure relationships in the area of the terminal branches are such that the pressure coming from the soft tissue prevails. This is the cause of the progressive development unfolding towards the bone, but originating at the soft tissues. The ancient wisdom of bone surgeons, «God protect us from the soft tissues», expresses the recognition that the bone itself is a reliable partner of our implantological work, but that the development and the healing process of the soft tissues are often cause for concern. This is equally true in periodontology, where the displacement of the hard tissues by the proliferating soft tissues is the very essence of the disease. Bone, as a rule, tolerates less nutrition and a lack of oxygen better than too much of both.
The bone is normally unable, for anatomical reasons, to change its internal blood supply. We doubt that bone that is as well-perfused as soft tissue could remain highly mineralized and that it would remain a substance which would be recognized as «bone» at all. It seems likely that overload osteolysis at disk plates occurs due to high perfusion initiated by the healing process after numerous cracks have occurred in the same region of the cortical bone. An accumulation of porosities due to pronounced remodelling may also contribute to the development of cortical defects of this type.
Quite a few questions remain open about the nature of bone at the nano scale. It is clear that the molecular structure of bone is responsible for the strength of the final product (Landis 1995; Huajian et al. 2003). This may imply, however, that the widely used engineering concept of stress concentration at flaw locations may not be applicable at the level of those nanoparticles of which bone consists. The results of the investigations and ideas of Frost presently form a sufficient basis for the implantologist’s work within the jawbones.
9.1.7 Role of Direct Bone Stimulation in Crestal Implants
From today’s vantage point, it is doubtful that vertically osseointegrated implant surfaces can stimulate the bone sufficiently to ensure that the bone volume is maintained. At least in the collar area, the forces that would cause the bone to remineralize will often be weaker than the forces promoting demineralization as a consequence of bacterial attack. This process gives rise to the crater-style collapses seen with crestal implants. In order to prevent bacterial retention, some crestal implant types come with a hybrid design in which the crestal segments do not feature surface enlargement. These designs have mainly been used for implants with very small threads and without horizontal load-transmitting surfaces. From the fact that these smooth surfaces are not capable of transmitting loads, it follows that even implants featuring vertical load-transmitting surfaces need to undergo basal osseointegration if they are to survive in an environment like the jaw characterized by high bacterial loads.
Although electric potentials are known to be capable of influencing the mechanism and direction of bone healing, it has not been demonstrated that electromagnetic fields applied directly on titanium implants can promote «healing» of the peri-implant bone structures (Buzza et al. 2003).
9.1.8 Force, Deformation, Pressure and Flow
As BMUs are formed, the bone becomes more porous and thus less resistant. The degree of mineralization in remodelled bone is 20–35% lower than in fully mineralized primary bone structures. The loss in tensile strength is greater than the loss in shear strength. When the elastic range is exceeded, plastic deformation will occur even in osteonal bone, and it will occur earlier in remodelled areas. The attainable level of plastic deformation is highest if the direction of the incoming load is parallel to the osteons. If an equivalent load meets the osteons axially, the attainable level of plastic deformation is smaller.
Osteonal perfusion is caused by the pressure and frequency of blood circulation (10 mm Hg at 2 Hz) and muscle contraction (30 mmHg at 1 Hz); the most important aspect, however, is mechanical loading (100 microstain at 1 Hz). Capillary forces play a role as well (Wang et al. 1999). Permeability across cement lines is, however, irrelevant. An increase of stress cycles per time unit will, however, result in a more pronounced fluid pressure gradient near the osteonal canals.
9.1.9 The 0–1 Property and the Self-Trabeculation Model – Micromodelling
Bone is a material that is capable of developing a trabecular structure by itself. Bone is also capable of changing the distribution of the load transmission task between different areas inside the bone or within the cortical bone (Mullender et al. 1994). A hypothetical perfectly homogeneous isotropic bone block would become increasingly anisotropic on being subjected to functional loading. In this process, it would develop both areas of heightened and areas of reduced mineralization. This behaviour is known as the «0–1 property». This behaviour is actually quite amazing, as the contact areas of the trabeculae, if for purely mathematical considerations, are always likely to be associated with peak stresses and hence with osteolytic tendencies. This effect, which is known as «checkerboarding», clearly indicates that we are unable to capture the true crux of the matter with our mechanistic thinking and mathematical systems such as FEM models, since all these approaches fail to account for the fuzzy behaviour of live bone. Mechanical testing of bone properties will per se induce bone remodelling, thereby causing the mechanical properties to change.
9.1.9.1 Clinical Application
The high clinical relevance of the 0–1 property becomes clear if we consider the following points:
-
When the implant is inserted into the bone, the macrotrajectories in the slot area are interrupted. In the healing phase, the situation may arise that the bone areas near the disk are mineralized better than the immediate area of insertion due to self-trabeculation of the cortical bone. In other words, the immediate area of insertion becomes the 0-area, while areas in the extended vicinity become 1-areas. The risk that this situation may arise is smaller in the mandible than in the maxilla. The stress-related mineralization pressure in the mandible is higher at strategic implant positions.
-
Whenever a large number of implants are inserted in the maxilla (particularly triple-disk or double-disk BOI implants in conjunction with long screws), the vestibular macrotrajectories are largely destroyed, and a healing process ensues that is undefined in terms of trajectory orientation and in which the effects of the implant surface prevail. Biomechanically, the situation can be compared to the grafting of large bone blocks (e.g. from the skull). Bone grafts of this type are not subject to any defined function during healing either. Therefore, implants to be loaded at a later time can basically be inserted right away in the same session. However, treatment with BOI implants offers greater advantages compared to grafting because these implants are inserted into native vital bone that will recover its macrotrajectorial properties faster.
-
The anisotropic properties of bone are massively changed or neutralized by inserting BOI implants in large numbers, so that the bone becomes virtually isotropic. When the anisotropic properties are later recovered, some portions of the BOI disks or even the implants themselves come to be located in 1-areas, while other parts will be located in 0-areas. This will, of course, influence the clinical mobility of individual implants. As functional alterations converting 1-areas to 0-areas and vice versa are always possible over the years, implant mobility does not change the probability of implant survival in any significant manner. Therefore, it takes a lot of experience to appreciate whether a BOI implant should be removed.
-
As additional implants are placed next to slightly mobile (ailing) BOI implants, the newly forming macrotrajectories are destroyed once again, which will normally result in both basal disks being integrated. Any granulation tissue that may have formed naturally has to be removed from the mobile disk. Bone grafting material can also be added, provided that the slot area does not communicate with the oral cavity. The longer remodelling phase of the bone grafting material may, at least in theory, cause the area to be once again trajectorially demarcated as a 0-area.
-
The 0-1 concept is also reflected in implant designs. Clinical results had shown that double-disk BOI implants with a disk-to-disk distance of 3 mm tended to give rise to bone loss on the crestal plate in 10–25% of all cases. This effect may have been due to the fact that the two plates interfered with bone healing as they defined the direction of the trajectories. For example, both plates may have generated overly adjacent 1-areas. These problems were no longer observed when the disk-to-disk distance was increased from 3 mm to 5 mm or when two disks of different resilience were used (the crestal disk has featured only one web bar ever since).
-
If BOI implants are inserted immediately after extraction, chances are that the entire bone area will be remodelled completely according to new macrotrajectorial criteria, so that direct interface contact will prevail in the early postoperative phase. This will promote implant healing. It is important, however, to reduce protruding alveolar walls from vestibular direction since the sockets will not be refilled with bone to the same width than when the tooth was still present. If reduction is not required for aesthetical reasons, membranes may be considered as additional treatment option. Secondary augmentation is also a good treatment alternative. The threaded pins have to be advanced far into the palatal native bone, taking the asymmetrical shape of the alveolar bone into consideration. A negative effect on the preservation of equal mastication can be expected from unilateral extractions, as the masticatory forces and new distribution of 0- and 1-areas will sometimes intrude on the implanted side, but staying clear of the level of the contralateral side. Therefore, these cases must be adequately and periodically followed up. Treatment concepts based on multiple implants need to be considered as well.
-
If mobile disk areas are observed primarily in the vestibular aspect of the distal maxilla, additional vestibular load transmission areas can be added with the help of clips (see Fig. 2.34). This approach takes advantage of nearby 1-areas of the bone and enables new bone formation to take place from the site of bleeding:
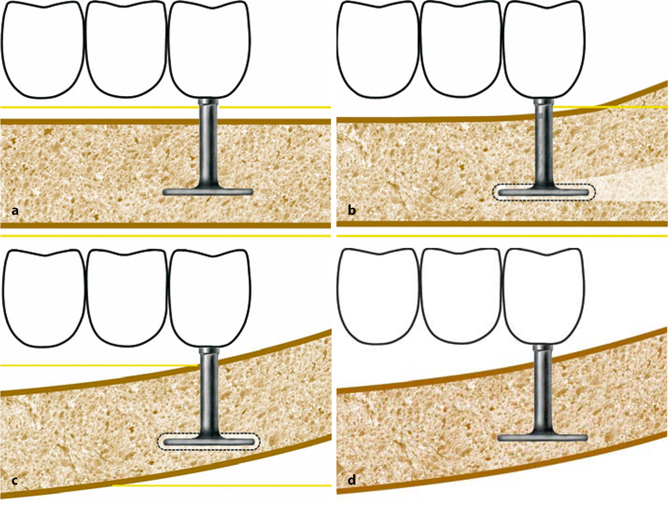
Fig. 9.6a–d.
These diagrams show a strictly horizontal trajectorial bone segment into which a BOI implant is inserted (a). Within the framework of bone defect healing, the orientation of that part of the bone changes (b). This means that the BOI implant may temporarily find itself in a remodelling area (0-area) and become mobile (a–c). However, as long as the BOI implant was inserted into a region of the bone that will remain stable in the long term, the bone areas located around the basal plate will remineralize and unite to form a new macrotrajectory, and the implant will stabilize (d). The bridge must keep all external forces constant and within acceptable dimensions. Internal structural changes in the jawbone will not always result in changes in shape that are easily discernible from outside
The following facts should be noted in this context:
Areas characterized by lower mineralization can also have their origin in so-called «acid regions» e.g. in the apical region of endodontically treated teeth. These bone areas are unable to transmit any loads. In their place, other bone areas mature to form 1-areas, while yet others develop into 0-areas. From a biomechanical point of view, then, it does not make a difference if a 0-zone develops because of excessive flexion (i.e. pronounced remodelling) or because of hyperacidification or changes in vascularization.
The development of 0-areas may change the load transmission paths across the entire jaw, even to the point where the survival of implants may be at stake. If implants in 1-areas suddenly end up in 0-areas, their stability must suffer. This in turn may have negative consequences for the stability of the entire superstructure and indeed possibly even the opposing jaw. To some extent, the distribution of 0-areas and 1-areas can be diagnosed preoperatively by radiographic examination. However, the preoperative distribution of these areas will be changed by the osteotomy of the implantation process, with the metabolic situation of the bone changing as well (suppuration/drainage, see Chapter 8).
If the masticatory function is equilibrated soon after implantation, for example by immediate loading, the bone healing process and the redistribution of the 0-areas and 1-areas can be steered in the direction of a normal distribution. Bilaterally imbalanced mastication, by contrast, will result in an atypical distribution of mineralization zones.
9.2 Form Follows Function
Will Zurich stop at this train?(Albert Einstein)
This chapter will certainly be the most interesting part of the book for the knowledgeable reader, as the dental literature does not contain a great many synoptic discussions of the facts herein described. The conclusions drawn from these observations, if applied in a consistent manner, would impact upon the entire field of dentistry and would call into question many aspects of what we have come to regard as best practice in our everyday clinical work into question. The compilation of individual factors in the middle of the chapter will provide the reader with more food for thought but will take a great amount of clinical perceptiveness and technical dexterity to be implemented. The quotation above characterizes the theory of relativity. With regard to the morphological changes caused by dental implantology and subsequent prosthetics, we might want to use a different wording: Where is the real reference point within the jaw bones?
May the quotation above sharpen the reader‘s awareness of the consequences of absolute relativity and of the all-embracing interdependence of all changes in jaws with intact dentitions and in jawbones with implant-supported restorations. Due to the gradual development of morphological changes, dentists and patients are often not aware of their extensive implications. As a result, these changes are not followed up properly, and they are utilized much too rarely for therapeutic purposes.
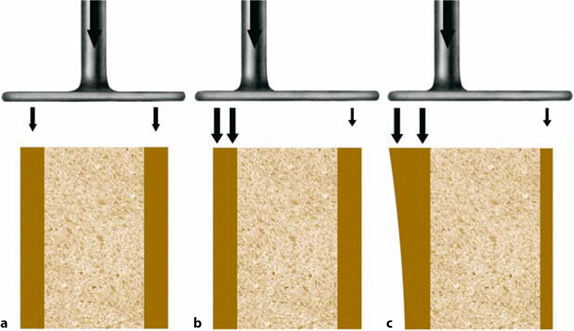
Fig. 9.7a–c.
Another aspect of the self-trabeculation model of the bone is observed if masticatory loads are applied via asymmetrical BOI implants; a shows the situation with a balanced load: the thickness of the cortical tissues remains unchanged in the long term both on the left and right sides. Asymmetrical BOI implants increase the load on the side on which the disk is shorter, creating more bone, even allowing the side that is less heavily loaded (here: right side) to develop into an 0-area. This situation is often found in the maxilla in the regions of the second premolar or the first molar
Our daily work with BOI has taught us to focus our attention primarily on masticatory function, since the role of mastication is much more important than the concept of static occlusion. The differences of BOI compared to conventional prosthetic approaches relying on natural teeth and crestal implants are substantial. For example, the usefulness of reducing the width of implant-supported teeth has never been demonstrated, although the demand has frequently been raised (Mericske-Stern 2000).
In situations where well-anchored teeth with long roots or long crestal implants are available in adequate numbers, the points discussed in this chapter might seem not all that important. After all, long-lasting structures (i.e. structures lasting out the warranty period) can be built on foundations like this even when any masticatory considerations are completely ignored. Nevertheless, any screw fractures, porcelain chipping, periodontal tooth mobility and/or implants lost will indicate that forces are present that are not tolerated well or that biomechanical developments do not seem to unfold according to plan.
Anyway, the BOI implantologist rarely gets to work under such favourable conditions but is usually faced by advanced vertical ridge resorption allowing only extremely small force-transmitting areas to be inserted into the bone. Therefore, any excessive prosthetic loading needs to be avoided or eliminated. On the other hand, BOI implants will allow some freedom with regard to the length and nature of the chewing surfaces.
In addition, the effects of the masticatory function on bone morphology need to be considered as well.
The following list provides some food for thought:
-
Jaw areas that are not per se stimulated by the load-transmitting disks are nevertheless subjected to functional loading. In the presence of equal muscle function on both sides of the jaw, any morphological differences are slowly evened out. As a prerequisite, the prosthetic restoration has to ensure a bilaterally uniform function (chewing function, number of chewing surfaces, length and position of chewing surfaces, muscle function and, by implication, muscle strength). In order to maintain this balanced function, occlusal adjustments have to be performed on a regular basis.
-
The rigid bridge will become part of the system itself and actively change its morphological surrounding. If, for example, complete bridges are inserted into jaws that are very dissimilar in shape, a process of morphological assimilation will take place. During this process, the osseointegrated implants are subjected to considerable stress. Patients themselves might not notice this stress as, e.g. subacute „pain“. Some patients will notice the tension as soon as the bridge is temporarily removed.
-
Primary restitution in the healing phase following implant placement, notably in the mandible, is achieved by deposition of periosteal bone on the one hand, and by formation of woven bone in the osteotomy slots on the other. Subsequently, the osteonal belt is rebuilt by BMU tunnelling across the jaw, which now undergoes a process of thorough remodelling. Increasing chewing forces and changing masticatory patterns will also prompt the jaw to change its morphology. The patients report similar stresses as patients wearing freshly readjusted fixed orthodontic appliances.
-
Areas that had been reduced in response to the insertion of dentures are able to develop in all directions defined by the modified function. Thus they can also develop towards the occlusal aspect (see Chapter 9.3 for a more detailed discussion), provided that the stimulus is not pre-empted by stronger stimuli coming from the prosthetic superstructure or implants. As a case in point, expansion (apposition/modellation) of the bone in crestal direction, which would be possible from a functional standpoint, is thwarted by chronic peri-implantitis in the presence of large-diameter crestal implants, but also by the overly deep and chronically infected transition zones between the abutments and those implants. This is not the case with BOI implants, as we shall learn in the section on histology.
-
Particular attention must therefore be devoted to cases in which the load-transmitting surfaces of BOI implants come to be located in bone areas that will undergo relative migration in the postoperative course. Due to their osseointegration, the implants themselves will move along with the bone. With regard to the relative vertical increase or migration of the crestal cortical bone in the distal mandible, this means that excessive prosthetic loading may occur in this area. After all, premature contacts are likely to emerge as the implants migrate upwards unless other factors offering vertical compensation come into play as well, such as elongation of the mandibular ramus or relocation of the associated TMJ.
-
Bone morphology changes as masticatory forces are being restored to their full potential. These changes will depend on the patient‘s age and metabolism. Any changes in bone shape and function, or in bone function alone, is followed by specific defined changes in internal bone architecture and by equally defined secondary changes in external shape. All of this is governed by mathematical laws (Wolff 1892). While there is no doubt that this statement is true, it remains confined to the changes going on inside the bone. The restorative dentist is faced with a longer chain of consequences. The changed bone shape is likely to be followed by changes in the shape of TMJ structure and in muscle function (chewing muscles, neck muscles), which will once again influence shape of the bone.
-
Reactive migration of the TMJs is frequently observed only several months after BOI-supported complete restorations were installed. Of course, one might contend at this point that the dentist simply may have been unable to properly register the occlusion. Based on our observations, it is more plausible that the TMJ structures may be decompressed as the jawbone is being remodelled, notably in its vertical dimension. The disk can thus change its position, and the masticatory system with its ever-present tendency of self-healing actually gets a chance to exploit this potential. At any rate, the technique of basal osseointegration offers unprecedented possibilities of functional therapy by allowing load transmission sites to be restored in the distal jaw areas, which are anatomically designed to carry the main burden of mastication. This type of therapy is not confined to influencing muscular structures but specifically offers an opportunity for extensive bone remodelling, for bone volume compensation, and for influencing the biomechanical situation in a physiological manner.
-
As we shall learn in the section on histology, morphology is also influenced by the osteotomy slots. Bone slots inserted into convex bone areas will reinforce the convexity as the bone, which is continuously kept under tension by the chewing muscles, will elastically yield to the slot. Subsequently, the original bone morphology will be restored by way of modelling as the osteotomy slots are being repaired. Since remodelling will also be going on in addition to modelling, mineralization in the affected jaw areas will decrease at large. After all, the tunnel-forming BMU will also extend to well-ossified areas, thereby reducing their osteonal density. If entire jaws are treated with a large number of BOI implants, the overall mineralization can be expected to change dramatically. Presumably, these effects are more pronounced in the mandible than in the maxilla.
-
Thus we are faced with a rather complex system of regulatory mechanisms that are governed by the laws of bone formation but also by the neurophysiological regulatory mechanisms of muscle function. Both mechanisms are not per se directed at accomplishing the clinically desirable healing process, but they sometimes require heavy dental adjustments to be made.
-
In terms of its bony architecture, the mandible can be compared to a rachitic long tubular bone.
-
The mechanical properties of bone are mainly a function of porosity, degree of mineralization, and orientation of collagenous fibres. Morphological changes results from muscle forces and their effect on individual areas.
-
From today’s standpoint, the concept of bone transformation as originally defined by Wolff includes the following elements:
-
Optimizing strength for weight
-
Arranging trabeculae in accordance with the main thrust of forces
-
Autoregulation of bone structures by cells reacting to mechanical stress
-
-
The bone initially rebuilt in vertical direction is characterized by porosities that are visible to the naked eye, at least in the superficial area, indicating that different levels of stimulation has taken place at different sites. Bone formation is locally excessive. Principal force directions are formed by remodelling only later. This remodelling can only come to pass if the newly formed areas are mechanically challenged, i.e. if they are put into “clinical service”.
-
By observing the strengthening effect of including or excluding specific enossal BOI implants, it becomes clear that “osseointegration” really means “osseoadaptation”. The bone has different mechanisms of adaptation at its disposal. For one thing, the distance between the mineralized matrix and the titanium surface can change. The mineralized matrix can act like a “smear layer” to overcome excessive differences in elasticity. On the other hand, it is conceivable that the degree of bone mineralization in the immediate or extended vicinity of the implant may be kept low as well (e.g. by strong continuous remodelling). At any rate, considerable differences in elasticity are possible, and the degree of bone mineralization can vary considerable depending on the requirements present.
-
It is reasonable to assume that the degree of mineralization in bone structures undergoing several surgical procedures will change at large. This change in local mineralization will, in its turn, change the overall elasticity of the jaw.
-
It is also reasonable to assume that each jaw segment has its own preoperative baseline elasticity as a consequence of cumulative mechanical, inflammatory, hormonal and genetic influences. This baseline elasticity may show considerable intraindividual differences.
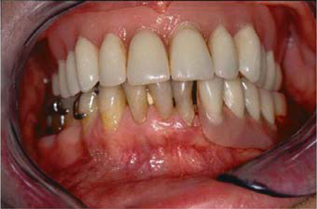
Fig. 9.8.
Preoperative clinical situation. The maxillary is restored with a complete denture; a cross-bite has been adjusted on the right. Natural dentition in the right mandible, partial denture in the left mandible (supported by a clasp on the mesial and by periodontium on the distal end)
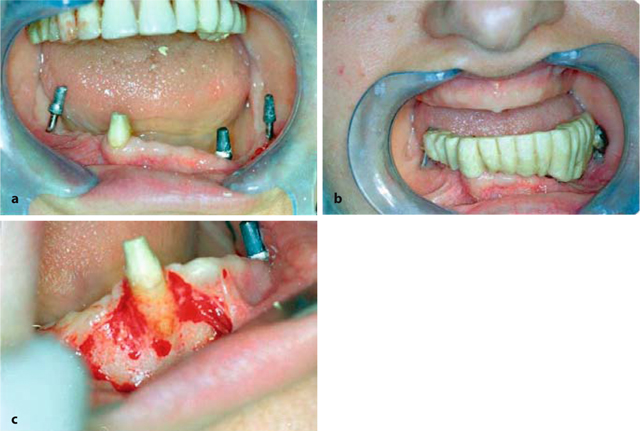
Fig. 9.9.
a The postoperative situation is characterized by considerable vertical differences within the mandible. If the residual natural tooth is preserved, it will be impossible to balance the volume by reducing the right side, as this would cause the tooth to intrude. Accordingly, the volume of the left ramus has to be increased, b At the patient’s request, tooth 43 was left in place for the primary fixed restoration. The bridge includes a cast framework and is covered with porcelain. Note the midline shift, which is already reflected in the bone structure. For functional reasons, the area 44–46 had undergone substantial resorption within a short time, giving the bony surroundings of the roots of tooth 43 a prominent appearance. The tooth has a very exposed position, c The cortical bone on the vestibular aspect of tooth 43 is extremely thinned out and partially fenestrated. Nevertheless, this tooth was kept until the new equilibrium in the mastication was found
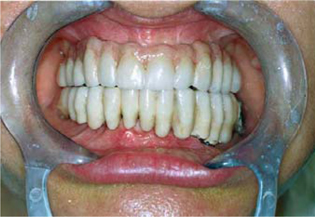
Fig. 9.10.
Tooth 43 was eventually replaced with another BOI implant prior to inserting the second bridge because it was likely to succumb to resorption sooner or later despite the considerable stimulus coming from the periodontal ligament. Note that the distal pontics must be located well above the mucosa, since the mandible is going to grow in a crestal direction, which may result in pressure spots or lever forces
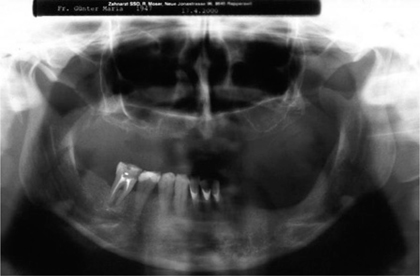
Fig. 9.11.
Baseline OPG. Extreme resorption on the left; the right ramus is, if anything, hypertrophic
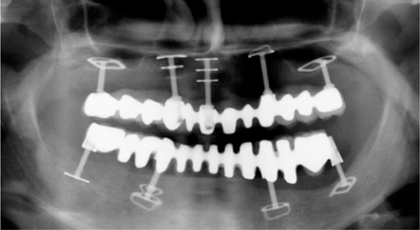
Fig. 9.12.
OPG obtained after insertion of the second mandibular bridge and restoration of the maxilla. The process of mutual jaw volume compensation can now be accurately monitored and needs to be facilitated by periodic occlusal adjustments with abrasive instruments (e.g. in the third quadrant). The fixed reference point of these implant-restoration systems is the occlusal plane. Spontaneous reconstruction of the vertical bone volume may take place either above the disks, or the implant has to move upwards together with the surrounding bone, which is only possible if an adequate occlusal clearance is established by abrasive instruments. The implant at 33 cannot osseointegrate in a definitive manner under these conditions. It is continuously under stress, which is noticed by the patient
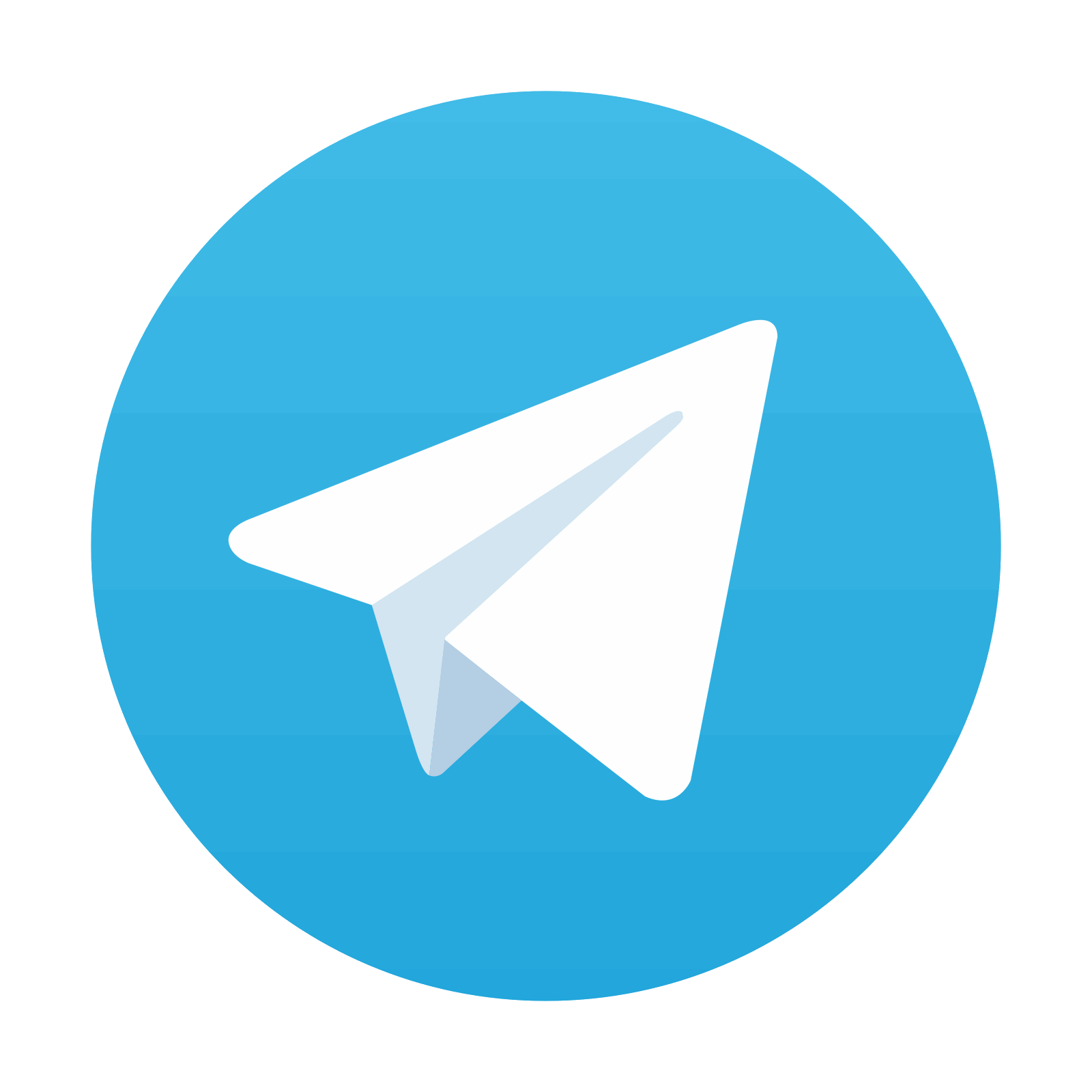
Stay updated, free dental videos. Join our Telegram channel

VIDEdental - Online dental courses
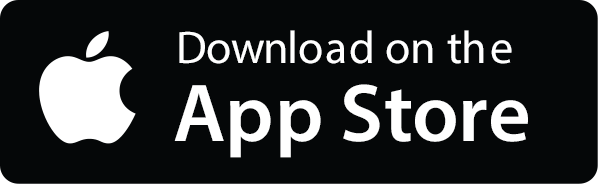
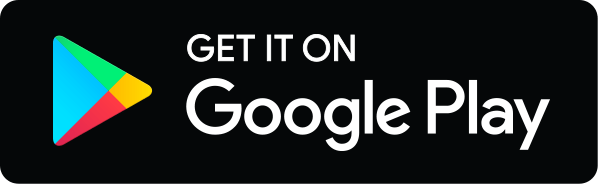