Abstract
Objective
To compare flexural strength of CAD-CAM and heat-pressed lithium disilicate.
Methods
For Pressed specimens (Group A), acrylate polymer blocks were cut with a saw in bars shape. Sprueing, investing and preheating procedures were carried out following manufacturer’s instructions. IPS e.max Press ingots (Ivoclar-Vivadent) were divided into subgroups (n = 15) according to translucency: A.1 = HT-A3; A.2 = MT-A3; A.3 = LT-A3; A.4 = MO2. Ingots were then pressed following manufacturer’s instructions. For CAD-CAM specimens (Group B) blocks of IPS e.max CAD (Ivoclar-Vivadent) were divided into subgroups: B.1 = HT-A3; B.2 = MT-A3; B.3 = LT-A3; B.4 = MO2. Specimens (n = 15) were obtained by cutting the blocks with a saw. Final crystallization was performed following manufacturer’s instructions. Both Press and CAD specimens were polished and finished with silica carbide papers of increasing grit. Final dimensions of the specimens were 4.0 ± 0.2 mm, 1.2 ± 0.2 mm, and 16.0 ± 0.2 mm. Specimens were tested using a three-point bending test. Flexural strength, Weibull modulus, and Weibull characteristic strength were calculated. Flexural strength data were statistically analyzed.
Results
The overall means of Press and CAD specimens did not differ significantly. Within the Press group different translucencies were found to have similar flexural strength. Within the CAD group, statistically significant differences emerged among the tested translucencies (p < 0.001). Specifically, MT had significantly higher flexural strength than HT and MO. Also, LT exhibited significantly higher flexural strength than MO.
Significance
The choice between IPS e.max Press and IPS e.max CAD formulations can be based on different criteria than flexural resistance. Within each formulation, for IPS e.max Press translucency does not affect the flexural strength while for IPS e.max CAD it is an influential factor.
1
Introduction
Metal-free restorations are largely used for prosthodontic rehabilitation. As a result of the increased esthetic demand, ceramic biomaterials have become more popular in the daily practice . According to their composition, ceramics can essentially be divided into two categories: glass- and polycrystalline-ceramics. As a general rule, glass matrix bestows esthetic characteristics, whereas crystals improve mechanical properties .
Since the introduction by McLean and Hughes of the first high strength ceramic in which alumina (Al 2 O 3 ) was added to feldspathic porcelain, research has pursued the objective of obtaining an ideal restorative material, which would combine natural tooth-like appearance and high mechanical performances . With this aim lithium oxide was added to the glass matrix, and a new material based on SiO 2 –Li 2 O–P 2 O 5 –Al 2 O 3 –K 2 O–ZrO 2 was used to fabricate dental restorations .
Among the lithium disilicates, IPS e.max Press and IPS e.max CAD are available for the heat-pressed and CAD-CAM technique respectively. For IPS e.max Press, ingots are already crystallized. By heating, the ingots become viscous and pressable . Conversely, for IPS e.max CAD the blocks exhibit an intermediate status (Li 2 SiO 3 ), necessary for the milling procedures. After milling, the restoration undergoes a heat-mediated chemical reaction, resulting in the lithium disilicate crystallization (Li 2 Si 2 O 5 ) . This crystallization process consists of two major events, nucleation and crystals growth . Due to the nucleating agents, the reaction is controlled and the final crystals shape, size, and content are determined . By using different concentrations of different agents, the microstructure is modified and the glass ceramic changes its mechanical and esthetic properties . Particularly, in order to render the desired shade and translucency, some oxides are used, acting as co-nucleating agents. These oxides interact with the described nucleation and crystallization processes, thus affecting the size of the crystals and, consequently the mechanical and physical properties .
It can therefore be expected that for IPS e.max changes in translucency reflect into modifications of the mechanical properties. Nevertheless, the information provided by the manufacturer does not discriminate on this regard.
Then, it seemed of interest to assess whether IPS e.max Press and IPS e.max CAD with different translucencies perform differently in terms of flexural strength.
Particularly, a study with a twofold objective was conducted. The first objective was to verify the manufacturer’s claim that IPS e.max Press has higher flexural resistance than IPS e.max CAD. The tested null hypothesis was that IPS e.max Press and CAD measure similar flexural strengths. The second objective was to assess the flexural resistance of specimens of IPS e.max Press and IPS e.max CAD with different translucencies. The tested null hypothesis was that no statistically significant differences in flexural strength exist among the different translucencies available for IPS e.max Press or CAD.
2
Materials and methods
2.1
Specimen preparation
For the heat-pressed technique (Group A), acrylate polymer blocks (IPS AcrylCAD, Ivoclar Vivadent, Schaan, Liechtenstein) were perpendicularly cut with a low-speed water-cooled diamond saw (ISOMET 1000, Buehler, Lake Bluff, Illinois), in order to obtain 60 bar-shaped specimens with dimensions of 4.0 in width, 1.2 mm in thickness and 16.0 mm in length . The specimens were then randomly divided into 4 subgroups ( n = 15).
Sprueing, investing, preheating, pressing, and finishing procedures were carried out according to the manufacturer’s recommendations. In particular, for each press cycle, 6 specimens were fixed to the ring base (IPS Investment Ring System 200 gr., Ivoclar Vivadent, Schaan, Liechtenstein) with a 3-mm long, 3-mm diameter extra-smooth wax wire (S-U-Wax wire colorless, Schuler-Dental, Ulm, Germany). Bars were kept at least 3 mm away from each other and oriented in such a way that a distance of 10 mm from the silicone ring was ensured laterally and upwards. The hole on the ring’s base was finally filled with red modeling wax (Tenatex, Kemdent, Wiltshire, United Kingdom). The investment procedure was carried on following manufacturer’s instruction and then the selected IPS e.max Press ingot (Ivoclar Vivadent, Schaan, Liechtenstein) was processed for pressing. Based on the translucency of the pressed ingots, 4 subgroups were defined: Subgroup A.1 = HT (High Translucency) shade A3; Subgroup A.2 = MT (Medium Translucency) shade A3; Subgroup A.3 = LT (Low Translucency) shade A3; Subgroup A.4 = MO (Medium Opacity) shade 2. After cooling, rough and fine divestments (Microjet, Simed, Baranzate, Italy) were carried out using polishing beads, respectively at 4-bar and 2-bar pressure. The reaction layer was removed by submerging specimens in a <1% hydrofluoric acid ultrasonic bath (IPS e.max Invex Liquid, Ivoclar Vivadent, Schaan, Liechtenstein) for 15 min, followed by polishing beads at 2 bar pressure. Finally, specimens were separated by cutting the base of the sprue with a low-speed water-cooled diamond disc.
For the CAD formulation (Group B), 4 lithium disilicate blocks (IPS e.max CAD, Ivoclar Vivadent, Schaan, Liechtenstein) were selected: Subgroup B.1 = HT shade A3; Subgroup B.2 = MT shade A3; Subgroup B.3 = LT shade A3; Subgroup B.4 = MO shade 2. Specimens ( n = 15) were obtained by cutting the blocks with a low-speed water-cooled diamond saw. Firing paste (IPS Object Fix Putty, Ivoclar Vivadent, Schaan, Liechtenstein) was used to avoid contact of the specimen with the firing tray. The final crystallization was performed with the EP 600 Combi (Ivoclar Vivadent, Schaan, Liechtenstein) furnace following manufacturer’s instructions.
Both CAD-CAM and heat-pressed specimens were polished and finished with water-cooled silica carbide papers of #600, #1200 and #2400 grit, until obtaining the desired dimensions of 4.0 ± 0.2 mm in width, 1.2 ± 0.2 mm in thickness and 16.0 ± 0.2 mm in length . A 45° edge was made at each major sharp edge, by keeping the specimens at 45° with metal tweezers .
2.2
Test method
A three-point bending test (3PBT) appliance was used . A support milled from a stainless steel block (A.I.S.I. type 316L), with two Cobalt-HSS (high speed steel) roller supports (Ø 2 mm, 13.00 span width) was used. Tests were performed in a universal testing machine (Triax 50, Controls, Milano, Italy), equipped with a Cobalt-HSS (high speed steel) loading tip, and operating at a cross-head speed of 1 mm/min. Specimens were tested in dry conditions and at room temperature. The fracture load was recorded in N and the flexural strength ( σ ) was calculated in MPa by using the following equation:
σ = 3 P l / 2 w b 2
where P is the fracture load in N, l is the span (distance between the center of the supports) in mm, w is the width in mm, and b is the height in mm of the specimen.
The Weibull characteristic strength ( σ 0 ) and the Weibull modulus ( m ) were calculated according to the following equation:
P f = 1 − exp [ − ( σ / σ 0 ) m ]
where P f is the probability of failure between 0 and 1, σ is the flexural strength in MPa, σ 0 is the Weibull characteristic strength in MPa (the value at the 63.2% of the specimens fail), and m is the Weibull modulus.
2.3
Statistical analysis of flexural strength data
In order to test the first formulated null hypothesis, all the Pressed specimens (Group A) were cumulatively compared with all the CAD specimens (Group B), using the t-test for Independent Samples, having verified that in either group data distribution was normal (Kolmogorov–Smirnov test), and variances were homogeneous (Levene test).
In order to test the second formulated null hypothesis, within each group a Kruskall–Wallis Analysis of Variance, followed by the Dunn’s Multiple Range test for post hoc comparisons, was run to compare the flexural strengths measured by the different available translucencies (Subgroups A.1–A.4; Subgroups B1–B4). The choice of non-parametric tests was dictated by the finding that the data did not meet the requirement of normal distribution according to the Kolmogorov–Smirnov test.
In all the analyses the level of significance was set at α = 0.05 and PASW Statistic 18.0 software (SPSS, Chicago, IL, USA) was used.
2.4
SEM evaluation
IPS e.max Press and IPS e.max CAD were qualitatively evaluated before and after the furnace-mediated heat treatment. Specimens ( n = 2) were polished and finished with water-cooled silica carbide papers of #600, #1200 and #2400 grit, etched for 60 s with 4.9% hydrofluoric acid (IPS Ceramic Etching Gel, Ivoclar Vivadent AG, Schaan, Liechtenstein), rinsed out with running water for the acid removal, ultrasonically vibrated in a 95% alcohol solution for 3 min (CP104, CEIA, Italy), and air dried with an oil-free stream. Each bar was secured to SEM (JSM-6060LV, JEOL, Tokyo, Japan) tabs with gold conducting tape, and gold coated in a vacuum sputter coater (SC7620 Sputter Coater, Polaron Range, Quorum Technologies, Newhaven, UK). Surfaces were observed at ×5000 magnifications for crystals morphology and orientation.
2
Materials and methods
2.1
Specimen preparation
For the heat-pressed technique (Group A), acrylate polymer blocks (IPS AcrylCAD, Ivoclar Vivadent, Schaan, Liechtenstein) were perpendicularly cut with a low-speed water-cooled diamond saw (ISOMET 1000, Buehler, Lake Bluff, Illinois), in order to obtain 60 bar-shaped specimens with dimensions of 4.0 in width, 1.2 mm in thickness and 16.0 mm in length . The specimens were then randomly divided into 4 subgroups ( n = 15).
Sprueing, investing, preheating, pressing, and finishing procedures were carried out according to the manufacturer’s recommendations. In particular, for each press cycle, 6 specimens were fixed to the ring base (IPS Investment Ring System 200 gr., Ivoclar Vivadent, Schaan, Liechtenstein) with a 3-mm long, 3-mm diameter extra-smooth wax wire (S-U-Wax wire colorless, Schuler-Dental, Ulm, Germany). Bars were kept at least 3 mm away from each other and oriented in such a way that a distance of 10 mm from the silicone ring was ensured laterally and upwards. The hole on the ring’s base was finally filled with red modeling wax (Tenatex, Kemdent, Wiltshire, United Kingdom). The investment procedure was carried on following manufacturer’s instruction and then the selected IPS e.max Press ingot (Ivoclar Vivadent, Schaan, Liechtenstein) was processed for pressing. Based on the translucency of the pressed ingots, 4 subgroups were defined: Subgroup A.1 = HT (High Translucency) shade A3; Subgroup A.2 = MT (Medium Translucency) shade A3; Subgroup A.3 = LT (Low Translucency) shade A3; Subgroup A.4 = MO (Medium Opacity) shade 2. After cooling, rough and fine divestments (Microjet, Simed, Baranzate, Italy) were carried out using polishing beads, respectively at 4-bar and 2-bar pressure. The reaction layer was removed by submerging specimens in a <1% hydrofluoric acid ultrasonic bath (IPS e.max Invex Liquid, Ivoclar Vivadent, Schaan, Liechtenstein) for 15 min, followed by polishing beads at 2 bar pressure. Finally, specimens were separated by cutting the base of the sprue with a low-speed water-cooled diamond disc.
For the CAD formulation (Group B), 4 lithium disilicate blocks (IPS e.max CAD, Ivoclar Vivadent, Schaan, Liechtenstein) were selected: Subgroup B.1 = HT shade A3; Subgroup B.2 = MT shade A3; Subgroup B.3 = LT shade A3; Subgroup B.4 = MO shade 2. Specimens ( n = 15) were obtained by cutting the blocks with a low-speed water-cooled diamond saw. Firing paste (IPS Object Fix Putty, Ivoclar Vivadent, Schaan, Liechtenstein) was used to avoid contact of the specimen with the firing tray. The final crystallization was performed with the EP 600 Combi (Ivoclar Vivadent, Schaan, Liechtenstein) furnace following manufacturer’s instructions.
Both CAD-CAM and heat-pressed specimens were polished and finished with water-cooled silica carbide papers of #600, #1200 and #2400 grit, until obtaining the desired dimensions of 4.0 ± 0.2 mm in width, 1.2 ± 0.2 mm in thickness and 16.0 ± 0.2 mm in length . A 45° edge was made at each major sharp edge, by keeping the specimens at 45° with metal tweezers .
2.2
Test method
A three-point bending test (3PBT) appliance was used . A support milled from a stainless steel block (A.I.S.I. type 316L), with two Cobalt-HSS (high speed steel) roller supports (Ø 2 mm, 13.00 span width) was used. Tests were performed in a universal testing machine (Triax 50, Controls, Milano, Italy), equipped with a Cobalt-HSS (high speed steel) loading tip, and operating at a cross-head speed of 1 mm/min. Specimens were tested in dry conditions and at room temperature. The fracture load was recorded in N and the flexural strength ( σ ) was calculated in MPa by using the following equation:
σ = 3 P l / 2 w b 2
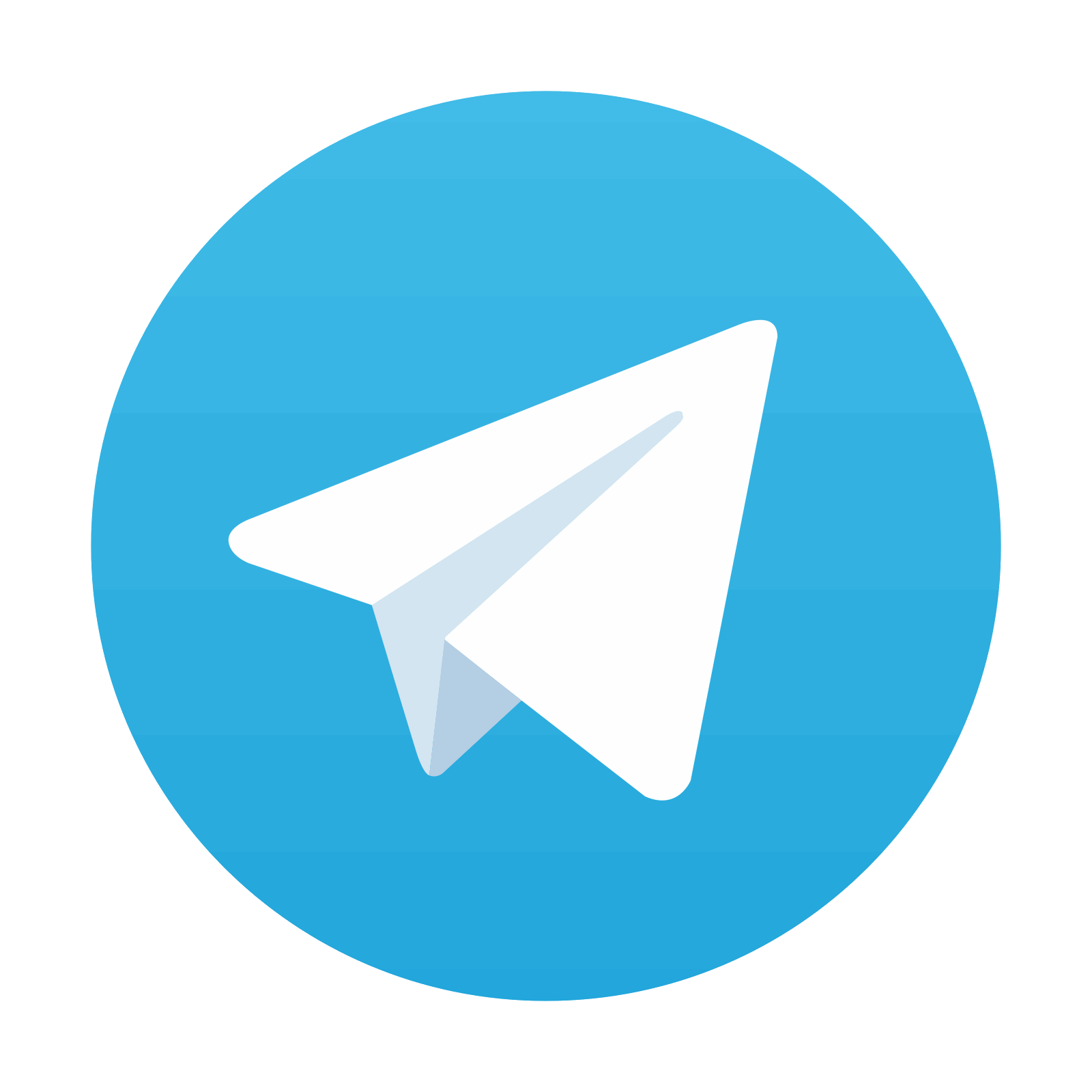
Stay updated, free dental videos. Join our Telegram channel

VIDEdental - Online dental courses
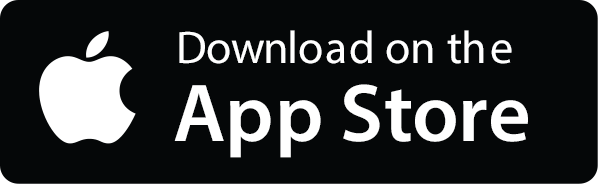
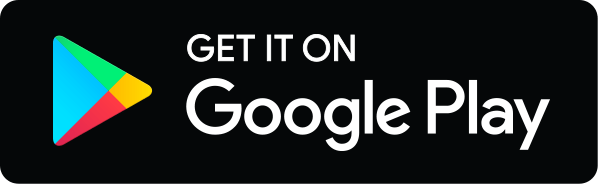