Abstract
The aim of this study was to compare two different types of surgical approaches, intrasinus and extramaxillary, for the placement of zygomatic implants to treat atrophic maxillae. A computational finite element simulation was used to analyze the strength of implant anchorage for both approaches in various occlusal loading locations. Three-dimensional models of the craniofacial structures surrounding a region of interest, soft tissue and framework were developed using computed tomography image datasets. The implants were modelled using computer-aided design software. The bone was assumed to be linear isotropic with a stiffness of 13.4 GPa, and the implants were assumed to be made of titanium with a stiffness of 110 GPa. Masseter forces of 300 N were applied at the zygomatic arch, and occlusal loads of 150 N were applied vertically onto the framework surface at different locations. The intrasinus approach demonstrated more satisfactory results and could be a viable treatment option. The extramaxillary approach could also be recommended as a reasonable treatment option, provided some improvements are made to address the cantilever effects seen with that approach.
The number of edentulous or toothless patients has increased over the last decade. The prevalence of edentulism is usually proportional to age or may be due to tooth extraction. Traditionally, patients with edentulous maxillae and mandibles are treated with conventional, complete dentures to restore aesthetics, function and comfort, but the denture wearers always report dissatisfaction due to uncomfortable and inefficient oral function.
The use of partial or complete dentures also results in accelerated bone loss. Denture wearers have reported dissatisfaction with their dentures; greater dissatisfaction being reported by patients with lower dentures than by those with upper dentures. Dental surgeons have introduced a new alternative to rehabilitate the edentulous atrophic bone with osseointegrated dental implants. The maxilla is a difficult arch to restore with osseointegrated implants because of its morphology and configuration. The limited bone quantity caused by bone resorption, especially in the posterior region of the maxilla, has resulted in low success rates for such implants. The availability of bone quantity in edentulous sites is crucial in planning treatment, deciding on surgical approaches, implant design, healing time and initial progressive bone loading during prosthetic reconstruction.
An advanced surgical technique of bone augmentation has been suggested to increase bone volume for the placement of dental implant in the posterior region of the maxilla. Bone grafting is normally chosen as a standard procedure to treat atrophic maxillae, and bones from the iliac crest are normally used as bone graft, but this procedure is resource-intensive and requires a relatively longer treatment time and a longer healing period for the patients. Harvesting of bone grafts could also cause morbidity in the donor site. Although bone augmentation can improve the configuration for potential placement of the implant into affected maxillae, lower success rates have been reported for grafted maxillae compared with non-grafted maxillae. The success rate of implants placed in the anterior maxilla depends primarily on the pre-treatment of the bone present. Brånemark System ® has introduced an alternative of utilising zygomatic implants to overcome these problems by anchoring the implants to bone regions free from bone regeneration or remodeling. The original purpose of zygomatic implants was to rehabilitate patients who had undergone maxillectomy due to tumour resection, trauma or congenital defects. The function of this implant has been expanded for the rehabilitation of patients with edentulous resorbed maxillae.
The zygomatic bone has been chosen as an implant anchorage site and has been evaluated in terms of its anatomical and biomechanical aspects. By using this implant, the use of the bone augmentation procedure can be eliminated or slightly reduced because of the strength of the zygomatic arch to retain the implant and the prosthesis in position. Four types of surgical procedures are available for the placement of zygomatic implants: intrasinus; sinus slot; extrasinus; and extramaxillary. In the intrasinus approach, the position of the implant body has to be maintained within the boundaries of the maxillary sinus, which results in a bulky dental prosthesis as the implant head emerges in a more palatal aspect. The extrasinus approach is mainly used to treat patients who have a pronounced buccal concavity. In the extrasinus approach, the zygomatic implant head is positioned closer to the alveolar crest bone, which reduces the size of the prosthesis. The extramaxillary approach is significantly different from the other approaches because only the implant body is anchored in the zygomatic arch. Hence, the emergence of the implant head will be more prosthetically correct in comparison to the intrasinus or extrasinus approaches. The main reasons for the introduction of these different surgical approaches for the placement of zygomatic implants are the mechanical resistance arising during mastication due to the location of the implant head and aesthetics. Every surgical approach has its own unique characteristics to increase the survival times of zygomatic implants during physiological function. No specific indication has been found, to date, to determine the best approach for the placement of implants. The intrasinus approach is the most common approach used in the clinical setting; the new extramaxillary approach was introduced to simplify the previous protocol of implant surgery.
Despite the high success rate reported for zygomatic implants, failures occur regardless of the type of surgical approach used. Many clinical reports describe a higher incidence of complications with the use of the classical intrasinus surgical approach. The bulky prosthesis in the intrasinus approach may affect dental hygiene and increase mechanical resistance to cause patient discomfort. Complications of peri-implant soft tissue bleeding and increased probing depth probably occur due to the inappropriate position of the zygomatic implant head and abutment. Mobility of the implant body and fracture of the abutment screw are some of the complications reported for the extramaxillary approach. It can be concluded that most of the complications associated with these surgical approaches for the placement of zygomatic implants are mainly caused by insufficient primary stability achieved by the zygomatic implants in supporting the prostheses.
Finite element analysis (FEA) presents several advantages, including reliable stress and strain distribution, accurate representation of complex geometries and simple model modification. It has also been proven to be an acceptable method to evaluate dental implant systems accurately in relation to other methods. The use of two-dimensional (2D) FEA is not recommended to simulate clinical situations because of the invalidity of model representation compared with three-dimensional (3D) FEA.
To the best of the authors’ knowledge, there have been no comparative studies between these surgical approaches. The objective of the study is to investigate two of the surgical approaches, intrasinus and extramaxillary, for stress and displacement distribution within the bones, framework and implants by using 3D FEA in various occlusal loading locations.
Materials and methods
A series of computed tomography (CT) image datasets was used to develop 3D models of a complete acrylic denture wearer with some degree of resorption by using Mimics 10.01 (Materialise, Leuven, Belgium), as depicted in Fig. 1 . The CT images consisted of 460 axial slices at 0.7 mm intervals with a resolution of 0.7 mm/pixel. The selected region of interest was in the maxilla and the zygomatic bone on both sides, which also covered the infrazygomatic crest, anterior nasal spine, zygomatic process, temporal process, frontal process and the orbital floor surface. The model dimensions were 111.9 mm in length, 46.5 mm in height and 52.4 mm in width. The cortical layer of the maxilla had a thickness ranging from 0.5 to 1.17 mm.

3D models of framework representing a partial prosthesis and soft tissues were also reconstructed based on similar CT image datasets. Subsequently, a partial framework with a flange was modelled to be 1.52–3.46 mm thick, 12.45–19.06 mm wide and 15.41–18.37 mm high. Two different designs of the framework were produced in which the design for the intrasinus approach was bulkier than that for the extramaxillary approach due to the expected emergence of implant heads in the palatal area. The gap along the maxillary arch between the palatal surface of the bone and the inside surface of the complete framework was used to develop a soft tissue model with a thickness of 0.39–5.58 mm.
The height and width of the atrophic maxilla to be treated were measured to determine a suitable approach for treatment, either through the use of zygomatic implants alone or in conjunction with conventional implants. Based on the measurements, the average heights of the anterior and posterior maxilla sections were 8.1 and 5.8 mm, respectively. The width of the alveolar ridge in the molar region was 9.7 mm. These dimensions fulfilled edentulous jaw classification criteria, described by Cawood and Howell, being Class III and Class V for the anterior and posterior maxillae, respectively. Therefore, the patient could be treated with a zygomatic implant placed bilaterally in conjunction with two conventional implants in the anterior region. The distance from the jugale point of the zygoma to the alveolar crest was measured to determine the length of the zygomatic implant to be used. The jugale point is defined as the point on the zygomatic lateral surface at the most depressed point of the transitional region from the lateral margin of the zygomaticofrontal process to the upper margin of the zygomaticotemporal process. The angulation of the zygomatic implant was determined between the long axis of the implant body and the plane through the bilateral infraorbital foramen, perpendicular to the midsagittal plane. The distance from the jugale point to the alveolar crest was 48.9 mm, and the angulation of the zygomatic implant was 45.7°.
3D computer-aided design (CAD) software, SolidWorks 2009 (Dassault Systèmes SolidWorks Corp., Concord, MA, USA) was used to develop implant models. The construction of the implant models required matched abutment models to connect the implant body to the prosthesis. Two 46.5 mm zygomatic implants with different diameters and thread distributions and two multi-unit abutments from Brånemark System ® (Nobel Biocare AB, Gotebörg, Sweden) were used ( Fig. 2 ). Two 4 mm × 10 mm implants with a 30°-angle multi-unit abutment from the same manufacturer were chosen for the conventional implant. The abutment body and screw were modelled as one part. The original feature of the implant body with its thread distribution was ignored and simplified to that of a step cylinder for the zygomatic implant models and a taper cylinder for the conventional implant models. The 3D solid implant models were transferred to other CAD software from ABAQUS, Inc. to generate surface triangular elements before the virtual surgery simulation. All models have been meshed with 0.5 mm triangular element size, which was the size used in a study conducted by Cattaneo et al.

Both the intrasinus and extramaxillary virtual surgical approaches were carried out in accordance with the procedure described by the Brånemark System ® protocol. Two points were identified for the placement of the implants: the starting and end points. The point of incisura (end point) was identified prior to the placement of the zygomatic implant model into the bone site as the implant had to be installed as posteriorly as possible or close to the point.
Several minor adjustments can be made to the position of the implant so that the implant head and its apical part are surrounded by bones. It was important to make sure that the intermediate part of the implant body did not perforate the maxillary anterior wall and that the end of the implant body did not emerge into the infratemporal fossa. The implant body had to be adjusted so that the implant platform could be positioned parallel to the occlusal plane to achieve a satisfactory prosthetic outcome. As a result, the position of the apical part of the implant was slightly diverging in the dorsal direction towards the infratemporal fossa. Similar steps were repeated for inserting another zygomatic implant model into the opposite side. The extramaxillary approach was introduced to simplify the earlier classical surgical approaches and was much easier to perform and simulate in comparison to the intrasinus approach. Zygomatic implants of a different design were placed externally into the maxilla and only anchored in the zygoma. The final positions of the zygomatic implants in the extramaxillary approach were found to have increased the framework cantilever length (23% longer) and decreased the horizontal implant offset (45% shorter) and anterior–posterior (A–P) distance. The A–P distance is determined between the centre of the most anterior implant and the most distal aspect of the posterior implant (41% shorter) ( Fig. 3 ). Conventional implants were located in the anterior region adjacent to the lateral incisors. The rationale was to distribute the overall implant configuration equally within the arch to achieve optimal support for the stability of the prosthesis.

The total number of elements for the intrasinus model was about 791,000, whereas the extramaxillary model had 787,000 tetrahedral elements. There were approximately 196,000 and 194,000 nodes for the intrasinus and extramaxillary approaches, respectively. The friction coefficients, μ , for all contacting surfaces of the implants and the framework model were set to 0.3 to simulate the immediate loading condition. The thread distributions of the zygomatic implant designs were different for the two approaches and were accordingly simulated via their contact properties. For both the approaches, the threaded part of the implant body was assigned a friction coefficient of 0.5 to represent its strong attachment to the bone. The contact surfaces between cortical-cancellous and cortical-gingival soft tissues were assumed to be as perfectly bonded by merging the nodes between the two contacted models.
All materials for FEA models were assumed to be isotropic, homogenous, and linearly elastic. The material properties of all models are shown in Table 1 . The implants and abutments were made of Ti6Al4V titanium alloy, whereas the framework was made of gold alloy.
Material | Young’s modulus, E (MPa) | Poisson’s ratio, v | References |
---|---|---|---|
Cortical | 13,400 | 0.30 | Geng et al. |
Cancellous | 1000 | 0.30 | Meyer et al. |
Soft tissue | 2.8 | 0.40 | Cheng et al. |
Framework | 100,000 | 0.30 | Ujigawa et al. |
Implants | 110,000 | 0.33 | Geng et al. |
Two types of load were applied to the finite element models being simulated: occlusal and masseter loads. A simulated occlusal load of 150 N was applied as a vertical load at different locations on the framework surface, specifically at the central incisor (L1), first premolar (L2), first molar (L3) and second molar region (L4) to represent more realistic food positions during biting or chewing. All vertical loads were applied along the z -axis, which is parallel to the standard implant axis. A simulated masseter load of 300 N with force components of −12.42 N along the x -axis, −53.04 N along the y -axis and 25.14 N along the z -axis was applied to the left side of the bone. For the right side, similar magnitudes of forces were applied but in the opposite direction, except for the z -axis force component. The simulated masseter load represented the masseter muscle action and was applied to the muscle attachment area on the zygoma as a distributed load ( Fig. 4 ).

For the boundary conditions, the posterior and top cutting planes were constrained in the x , y and z directions to prevent any movements.
A comparison was made in terms of mechanical stress and displacement pattern to determine the behaviour of the bones and implant reactions under the applied simulated mastication loading conditions. Equivalent von Mises (EQV) stress is an appropriate stress measurement to be chosen for ductile materials such as titanium. The data for EQV are shown in Table 2 , and the displacement data are presented in Table 3 . The results have also been presented in a colour band contour plot with blue representing low stress or displacement magnitude and grey representing high magnitude.
Models | IA | EA | ||||||
---|---|---|---|---|---|---|---|---|
L1 | L2 | L3 | L4 | L1 | L2 | L3 | L4 | |
Cortical bone | 1.96 | 1.79 | 1.65 | 1.97 | 2.02 | 1.70 | 1.69 | 2.04 |
Cancellous bone | 1.64 | 1.46 | 1.34 | 1.35 | 1.67 | 1.37 | 1.35 | 1.39 |
Framework | 158.00 | 193.00 | 196.68 | 294.27 | 136.05 | 226.25 | 223.57 | 459.35 |
ZI1 | 32.51 | 64.80 | 64.48 | 63.73 | 42.07 | 37.61 | 53.58 | 91.30 |
ZI2 | 22.35 | 23.50 | 15.40 | 15.12 | 59.38 | 32.91 | 31.81 | 40.47 |
CI1 | 20.14 | 23.60 | 7.48 | 4.80 | 22.49 | 10.04 | 4.50 | 5.04 |
CI2 | 17.42 | 16.83 | 5.73 | 4.49 | 23.45 | 12.00 | 6.97 | 16.83 |
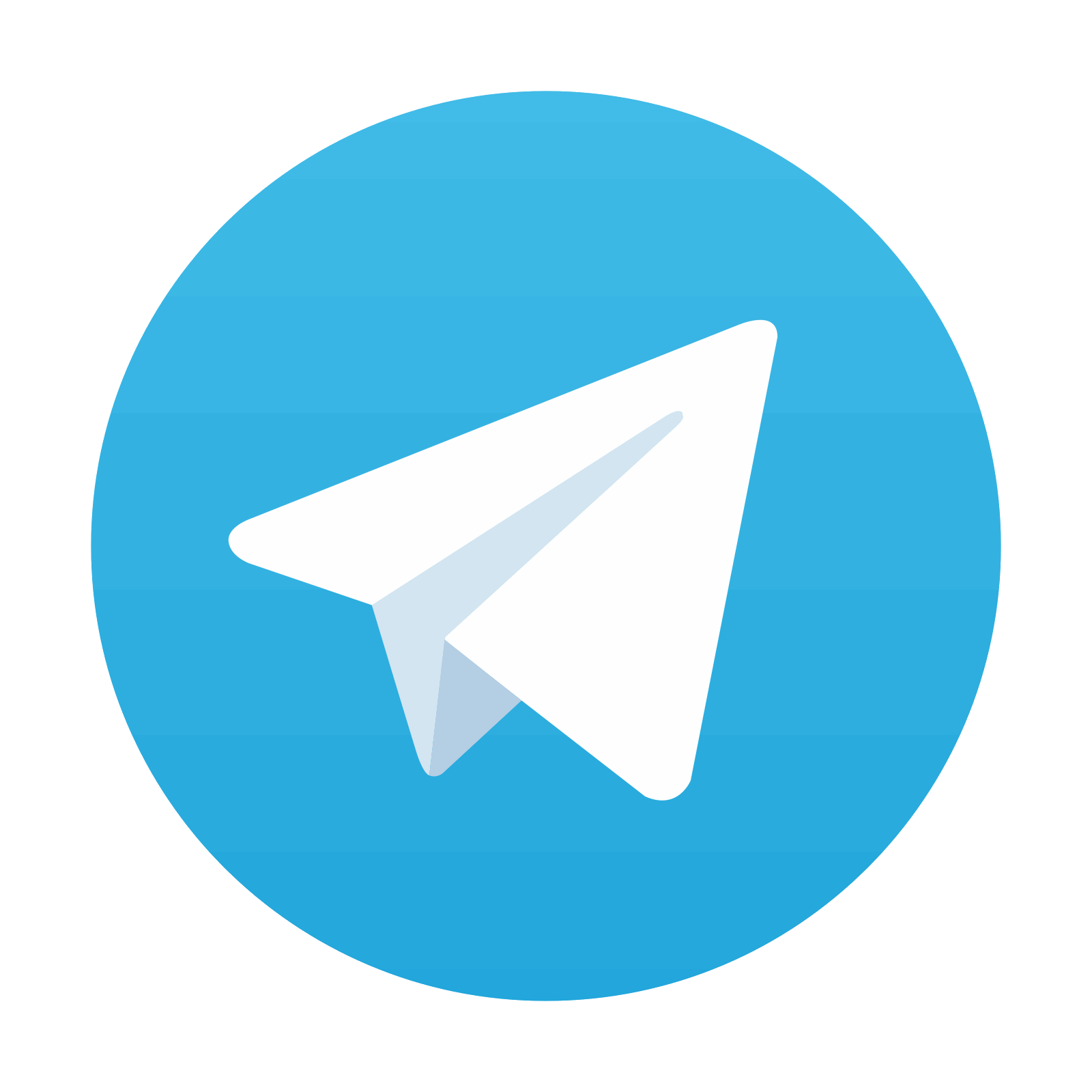
Stay updated, free dental videos. Join our Telegram channel

VIDEdental - Online dental courses
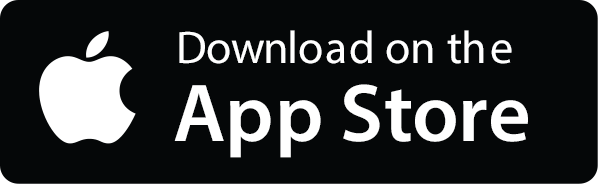
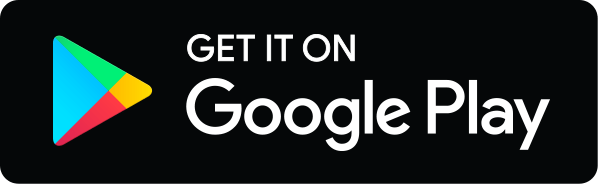
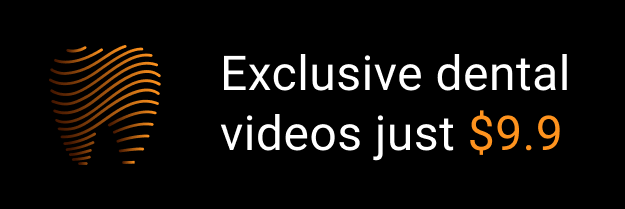